- 1Human Microbiome Research Program, Faculty of Medicine, University of Helsinki, Helsinki, Finland
- 2Translational Immunology Research Program, Faculty of Medicine, University of Helsinki, Helsinki, Finland
- 3Department of Experimental Oncology, European Institute of Oncology, Milan, Italy
- 4Translational Genomics Research Institute, Pathogen and Microbiome Division, Flagstaff, Arizona, AZ, United States
- 5Helsinki University and Helsinki University Hospital, Department of Rheumatology, Helsinki, Finland and ORTON Orthopedic Hospital of the Orton Foundation, Helsinki, Finland
Odoribacter splanchnicus, belonging to the order Bacteroidales, is a common, short-chain fatty acid producing member of the human intestinal microbiota. A decreased abundance of Odoribacter has been linked to different microbiota-associated diseases, such as non-alcoholic fatty liver disease, cystic fibrosis and inflammatory bowel disease (IBD). The type strain of O. splanchnicus has been genome-sequenced, but otherwise very little is known about this anaerobic bacterium. The species surfaces in many microbiota studies and, consequently, comprehension on its interactions with the host is needed. In this study, we isolated a novel strain of O. splanchnicus from a healthy fecal donor, identified it by genome sequencing and addressed its adhesive, epithelium reinforcing and immunoregulatory properties. Our results show that O. splanchnicus strain 57 is non-adherent to enterocytes or mucus, does not reinforce nor compromise Caco-2 monolayer integrity and most likely harbors penta-acylated, less endotoxic lipid A as part of its lipopolysaccharide (LPS) structure based on the lack of gene lpxM and in vitro results on low-level NF-κB activity. The studies by transmission electron microscopy revealed that O. splanchnicus produces outer membrane vesicles (OMV). O. splanchnicus cells, culture supernatant i.e., spent medium or OMVs did not induce interleukin-8 (IL-8) response in HT-29 enterocyte cells suggesting a very low proinflammatory capacity. On the contrary, the treatment of HT-29 cells with O. splanchnicus cells, spent medium or OMVs prior to exposure to Escherichia coli LPS elicited a significant decrease in IL-8 production as compared to E. coli LPS treatment alone. Moreover, O. splanchnicus spent supernatant induced IL-10 production by immune cells, suggesting anti-inflammatory activity. Our in vitro findings indicate that O. splanchnicus and its effector molecules transported in OMVs could potentially exert anti-inflammatory action in the gut epithelium. Taken together, O. splanchnicus seems to be a commensal with a primarily beneficial interaction with the host.
Introduction
Odoribacter splanchnicus type strain 1651/6T, previously classified as Bacteroides splanchnicus, was originally isolated from a human stool specimen and abdominal abscess (Werner et al., 1975). In 2008, the new genus Odoribacter was separated from Bacteroides forming its own cluster within the order Bacteroidales and family Odoribacteraceae (Hardham et al., 2008; Munoz et al., 2016). The two other species belonging to the genus are Odoribacter laneus, isolated from human feces (Nagai et al., 2010), and Odoribacter denticanis, isolated from canine periodontitis (Hardham et al., 2008). Gram-negative, strictly anaerobic and non-spore forming O. splanchnicus is a common member of human intestinal microbiota (Göker et al., 2011). The complete genome sequence of the type strain revealed that only 61% of the protein-coding genes could be associated with a putative function (Göker et al., 2011). The growth of O. splanchnicus is enhanced by hemin and bile acid. Its main fermentation products are acetic, propionic and succinic acids, but the bacterium also produces butyric, isovaleric and isobutyric acids in smaller quantities (Werner et al., 1975). In fact, the beneficial effects of Odoribacter as part of a healthy, balanced human gut microbiota are primarily attributed to its capacity to produce short-chain fatty acids (SCFA). Dietary fiber and resistant starch are metabolized by commensal bacteria to SCFAs which in turn serve as a vital energy source for the intestinal epithelial cells (Koh et al., 2016). These bacterial metabolites, especially butyrate, have a wide range of health-promoting effects on the gut epithelium, such as maintaining epithelial barrier function and gut homeostasis, exerting anti-inflammatory and immunomodulatory activity in the intestinal mucosa as well as suppressing proinflammatory cytokines (Parada Venegas et al., 2019).
The lower relative abundance of Odoribacter has been associated with different disorders, such as non-alcoholic fatty liver disease (NAFLD), cystic fibrosis and inflammatory bowel disease (IBD) (Morgan et al., 2012; Lewis et al., 2015; Burke et al., 2017; Li et al., 2018; Wang et al., 2018). The abundance of Odoribacter was found to be reduced in the microbiota of patients with ileal Crohn’s disease (CD) and pancolitis, which is the most severe form of ulcerative colitis (UC) affecting the entire colon (Morgan et al., 2012). Pediatric CD patients had also reduced relative abundance of Odoribacter compared to the healthy individuals (Lewis et al., 2015; Wang et al., 2018). The levels of Odoribacter and other SCFA-producing gut bacteria were elevated and maintained after Infliximab therapy in pediatric CD patients compared to the controls, suggesting that a sustained response to the therapy was associated with the abundance of SCFA-producing taxa in the gut (Wang et al., 2018). Furthermore, diarrhea-predominant irritable bowel syndrome (IBS) patients with a higher relative abundance of Odoribacter and Faecalibacterium at baseline level were responding better to a multispecies probiotic combination treatment, having reduction in symptoms and beneficial changes in inflammatory markers (Hod et al., 2018).
The role of Odoribacter abundance and the availability of SCFAs in metabolic disorders still remains an open question. The abundance of genus Odoribacter was negatively correlated with systolic blood pressure in obese and overweight pregnant women, suggesting that SCFA-producing taxa could have an influence on the host blood pressure (Gomez-Arango et al., 2016). Interestingly, Odoribacter seems to be associated with metabolic health benefits coupled with Akkermansia based on previous studies on metabolism-related pathologies, such as obesity, metabolic syndrome and diabetes (Brahe et al., 2015; Etxeberria et al., 2017; Lim et al., 2017; Lai et al., 2018). In a twin study, SCFA-producing genera Akkermansia and Odoribacter were enriched in healthy individuals compared to adults with metabolic syndrome (Lim et al., 2017). Furthermore, these two genera positively correlated with a healthy lipid metabolism profile in obese women (Brahe et al., 2015). On the contrary, one study showed a higher prevalence of Odoribacter in male subjects with hypercholesterolemia (Granado-Serrano et al., 2019). In a genetic murine model of obesity, both Akkermansia and Odoribacter were increased during a Pterostilbene treatment, which has been shown to improve metabolic profile, such as insulin sensitivity (Etxeberria et al., 2017). In addition, Odoribacter was associated with the beneficial effect of fecal microbiota transplantation (FMT) in a mouse model regardless of the diet, as the relative abundance of the bacterium increased as a result of FMT in high fat and normal diet groups (Lai et al., 2018).
Besides SCFAs, O. splanchnicus has been shown to produce specific bacterial sphingolipids i.e., sulfonolipids, which potentially exert a bioactive function in the intestine (Walker et al., 2017). Sphingolipids, produced by eukaryotes and a small subset of bacterial species, and their metabolites can regulate various cellular processes in the host, such as cell proliferation, apoptosis, differentiation and inflammation (Heaver et al., 2018). Bacteroides-derived sphingolipids participate in maintaining gut homeostasis as shown in a study where germ-free mice colonized with sphingolipid-deficient B. thetaiotaomicron developed intestinal inflammation and altered ceramide pools (Brown et al., 2019). Sphingolipids and other bacterial molecules are likely transported in outer membrane vesicles (OMV), which are spherical, membranous blebs released from the outer membrane of Gram-negative bacteria, and able to navigate through the thick mucus layer to the epithelium (Schwechheimer and Kuehn, 2015; Heaver et al., 2018). Indeed, OMVs produced by Bacteroides spp. have been localized in the intestinal mucosa interacting with the host immune cells (Hickey et al., 2015; Maerz et al., 2018).
The studies on the host-microbe interactions of O. splanchnicus are scarce creating a clear need to investigate its properties. In this study, we addressed the adhesive, epithelium reinforcing and immunomodulatory properties of O. splanchnicus strain isolated from a healthy fecal donor. We also studied the effect of O. splanchnicus cells, LPS, spent medium and OMVs on inflammatory responses in different human cells. To our knowledge, this is the first study to visualize O. splanchnicus OMVs and to study their anti-inflammatory potential.
Results
Genome of the Strain O. splanchnicus 57
The genome of novel O. splanchnicus 57 strain (isolated from a healthy fecal donor) was obtained by whole genome sequencing (WGS) to accurately identify the bacterium on species level with Kraken (Wood and Salzberg, 2014). The assembled genome size was 4.2 Mb with a G + C content of 43.3% (Table 1). Of the 3544 genes, 1687 (47.6%) were hypothetical proteins without a putative function assigned. O. splanchnicus 57 genome assembly matched the O. splanchnicus type strain 1651/6T genome (NC_015160.1) with 99.84% identity and 85% query coverage. The assembled genome data of O. splanchnicus 57 was screened for genes that encode enzymes for Kdo2-lipid A modification using BLAST (Basic Local Alignment Search Tool, BLASTn algorithm). The genes encoding LpxA-LpxL acyltransferases for the Kdo2-lipid A moiety synthesis were found, but the genome lacked the gene lpxM needed for the hexa-acylation of the lipid A, which indicates that O. splanchnicus 57 is likely to produce less toxic, penta-acylated form of LPS.
Mucosal Adherence and Epithelium Reinforcing Properties
We studied the adherence of O. splanchnicus 57 to (8 days post-plating) Caco-2 and HT-29 cell lines as well as to porcine mucus. The relative adherence percentage of the bacterium to the enterocyte cell lines and mucus was below 1%, which is considered to be unspecific, background level binding (Vesterlund et al., 2006; Figure 1A). Thus, O. splanchnicus 57 can be considered as non-adherent. In addition, we studied the ability of the isolate to enhance Caco-2 monolayer integrity using transepithelial electrical resistance (TER) measurements (Figure 1B). O. splanchnicus 57 did not induce changes in the epithelial integrity as the change in TER values remained at the same level as the medium control indicating that the isolate did not reinforce or compromise the epithelial monolayer.
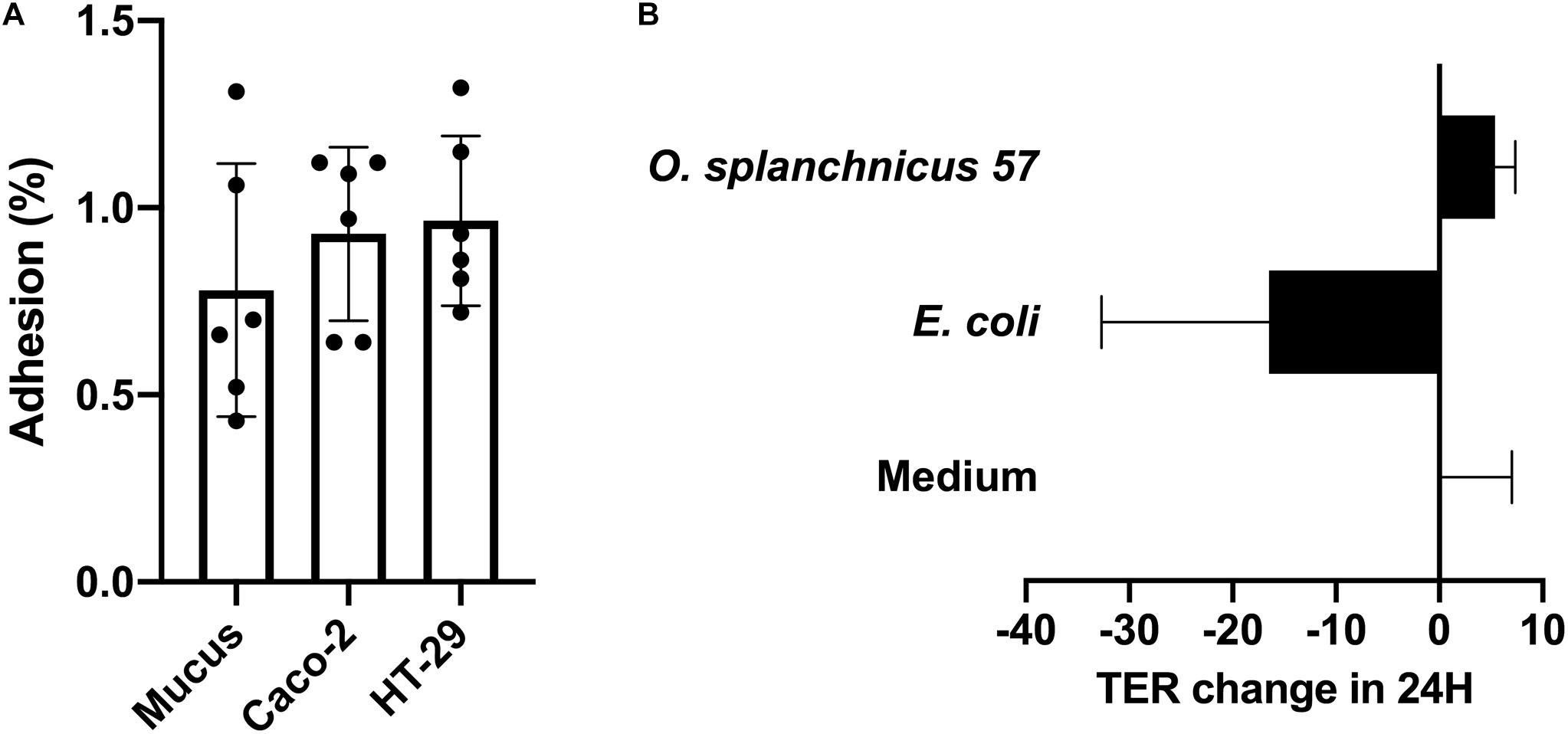
Figure 1. (A) Adherence (%) of O. splanchnicus 57 to 8-day-old Caco-2 and HT-29 cell lines as well as mucus. Adherence-% reflects the proportion of bound bacteria from that of the added. Data is shown as a mean and standard deviation of six technical replicates. (B) The effect of O. splanchnicus 57 cells on TER of 8-day-old Caco-2 monolayer. Results are shown as the mean of TER value change (Ω/cm2) in 24 h and standard deviation of three technical replicates (parallel wells). E. coli K12 was used as negative control and RPMI 1640 as the medium control. In both panels (A,B) results are from a representative experiment.
Stimulation of PBMCs With O. splanchnicus 57 Cells, Spent Medium and LPS
Next, we examined the immunomodulatory effect of O. splanchnicus 57 cells and the culture supernatant, i.e., spent medium, on the TNF-α and IL-10 production by healthy human peripheral blood mononuclear cells (PBMCs). Heat-killed E. coli K12 was used as a proinflammatory control stimulus in both experiments. There was no significant difference in the TNF-α/IL-10 ratio released from PBMCs following a 24-h stimulation with O. splanchnicus 57 cells or E. coli K12 (Data not shown). However, the spent growth medium of O. splanchnicus 57 induced significantly higher levels of IL-10 in relation to TNF-α compared to E. coli K12 suggesting different amounts of pro- and anti-inflammatory bacterial effector molecules present in the spent medium as compared to intact bacterial cells (Figure 2A; Supplementary Figure 1). TNF-α and IL-10 production by PBMCs is presented as ratios collectively from all donors in Figure 2A and as total concentrations from each donor separately in Supplementary Figure 1. PBMCs from each individual donor produced significantly higher IL-10 and lower TNF-α levels after a stimulation with O. splanchnicus 57 spent medium compared to E. coli (Supplementary Figure 1). The O. splanchnicus 57 spent medium stimulated IL-10 release in human PBMCs ranging between 100–330 pg/ml depending on the donor (Supplementary Figure 1). The GAM growth medium for O. splanchnicus 57 itself (i.e., unspent medium control) did not affect the cytokine production in PBMCs. Stimulation of human PBMCs with LPS isolated from O. splanchnicus 57 and E. coli K12 showed no significant difference in the ratio of proinflammatory cytokine TNF-α to anti-inflammatory cytokine IL-10 measured after 24 h of stimulation (Figure 2B). The ratio of TNF-α/IL-10 produced by PBMCs was lower but not statistically significant after induction with O. splanchnicus 57 or B. fragilis type strain LPS as compared to E. coli K12 LPS. While B. fragilis type strain LPS stimulated statistically higher IL-10 production in PBMCs from all donors, O. splanchnicus 57 LPS stimulated higher IL-10 production in PBMCs of one out of four donors as compared to E. coli LPS (Supplementary Figure 2).
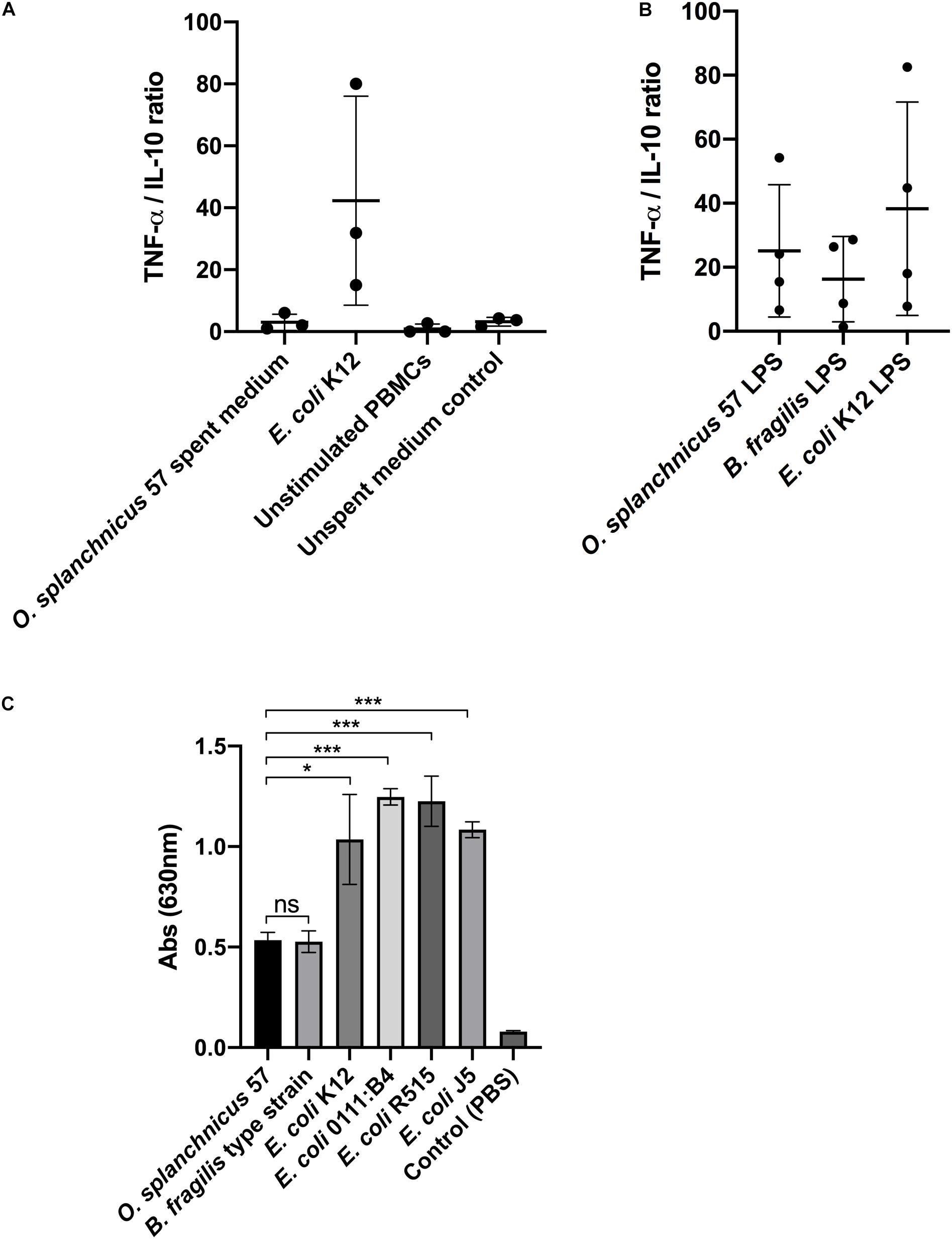
Figure 2. (A) Ratio of TNF-α and IL-10 released from PBMCs after stimulation with O. splanchnicus 57 spent medium. Heat-killed E. coli K12, unstimulated PBMCs and unspent medium were used as controls. The results are presented as a mean and standard deviation of three biological experiments using PBMCs isolated from the buffy coats of three different donors. (B) The ratio of TNF-α/IL-10 released from PBMCs after stimulation with 1 ng/ml of O. splanchnicus 57 and B. fragilis type strain LPS. E. coli K12 LPS was used as a control. The results are presented as a mean and standard deviation of four biological experiments using PBMCs isolated from the buffy coats of four different donors. (C) Activation of NF-kB in human THP1 dual reporter cells by stimulation with 10 ng/ml LPS extracted from O. splanchnicus 57, B. fragilis type strain and E. coli K12. LPS from three other commercial E. coli strains, as well as PBS, were used as controls.
O. splanchnicus 57 LPS-Induced NF-κB Signaling in THP-1 Cells
The lack of gene lpxM in the O. splanchnicus 57 genome indicated that the bacterium potentially harbors a penta-acylated lipid A, making its LPS less proinflammatory as compared to hexa-acylated LPS of E. coli. LPS extract from O. splanchnicus 57 was used to stimulate human THP-1 dual reporter cells to study the induction of NF-κB signaling (Figure 2C). After a 24-h stimulation, the level of NF-κB activity induced by O. splanchnicus 57 LPS was equivalent to B. fragilis type strain that was used as control (Figure 2C). The lipid A of B. fragilis type strain LPS has a penta-acylated, less endotoxic form compared to E. coli LPS (Weintraub et al., 1989), as also shown by the level of NF-κB signaling in human THP-1 cells induced by different bacterial LPSs in our experiments. Taken together, the results suggest that O. splanchnicus 57 LPS is less pro-inflammatory as compared to E. coli LPS and that the spent medium of O. splanchnicus 57 contains substances that counteract the pro-inflammatory effect of LPS.
O. splanchnicus 57 Secreted Outer Membrane Vesicles
Considering the potentially bioactive effector molecules secreted by O. splanchnicus 57, we studied the presence of OMVs in the bacterial culture. We used a previously published protocol (Elhenawy et al., 2014) with modifications to isolate OMVs. Transmission electron microscopy (TEM) was used to confirm the presence of OMVs in the preparations. Also, O. splanchnicus cells were also subjected to TEM. In the electron micrographs, O. splanchnicus 57 bacterial cells appeared as short rods as previously described in the literature (Göker et al., 2011). OMVs were clearly visible in the electron micrographs (Figure 3). The OMVs of O. splanchnicus 57 were observed to be smaller than 100 nm in size.
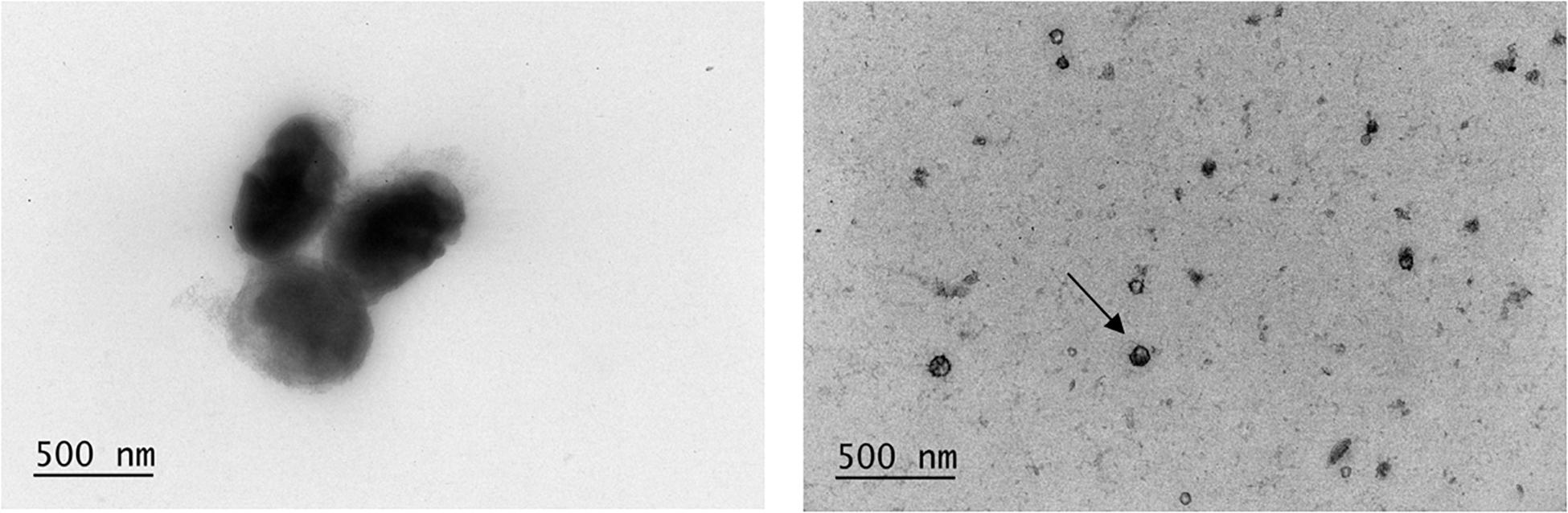
Figure 3. Electron micrographs of O. splanchnicus 57 cells and outer membrane vesicles (OMV, example pointed with an arrow).
Immunoregulatory Effect of O. splanchnicus 57 on HT-29 Cell Line
Next, we assessed the effect of O. splanchnicus 57 cells, spent medium and OMVs on the induction of proinflammatory cytokine IL-8 in enterocytes. HT-29 cell line was used to assess the ability of the strain to attenuate LPS-induced inflammatory responses i.e., IL-8 release from enterocytes as Caco-2 cell line has defects in the TLR4 signaling, which causes unresponsiveness to LPS stimulation (Van De Walle et al., 2010). HT-29 cells were incubated with different concentrations of the bacterium, spent medium and vesicles (Figures 4A–C). E. coli K12 and its OMVs were used as proinflammatory controls and B. fragilis as a control that does not elicit a reaction in HT-29 cells. McCoy 5A medium, the growth medium for HT-29 cells, was included as the background control. The 1:10 dilution of O. splanchnicus 57 and B. fragilis cells (corresponding to 107 cells per ml) prompted IL-8 responses that were above background but were four times lower as compared to the responses induced by E. coli K12 (Figure 4A). The 1:100 and 1:1000 dilutions of O. splanchnicus 57 and B. fragilis cells induced IL-8 release statistically at the similar level as the background showing a dose-dependent decrease in response, whereas E. coli K12 triggered a strong response with all the concentrations showing a saturated induction already with the most diluted sample. Thus, the difference in the proinflammatory capacity of E. coli and the two other commensals was clear. The same result could be observed when using spent medium, as both O. splanchnicus 57 and B. fragilis spent media diluted to 1/2 or 1/4 of the adjusted concentration elicited only a minor IL-8 response, which did not differ significantly from the background and GAM medium control (Figure 4B). In contrast, the 1/2 dilution of E. coli K12 spent medium was excessively toxic to the HT-29 cells (not shown due to partial cell death resulting in unreliable quantification), and 1/4 spent medium dilution triggered a major induction of IL-8 in HT-29 cells (582 ± 78 pg/ml) significantly above the background level. Also, OMVs isolated from O. splanchnicus 57 and E. coli K12 induced significantly distinct IL-8 responses with all the amounts used (107 – 1010 vesicles) as E. coli K12 OMVs elicited a four-fold stronger response than O. splanchnicus 57 OMVs (Figure 4C).
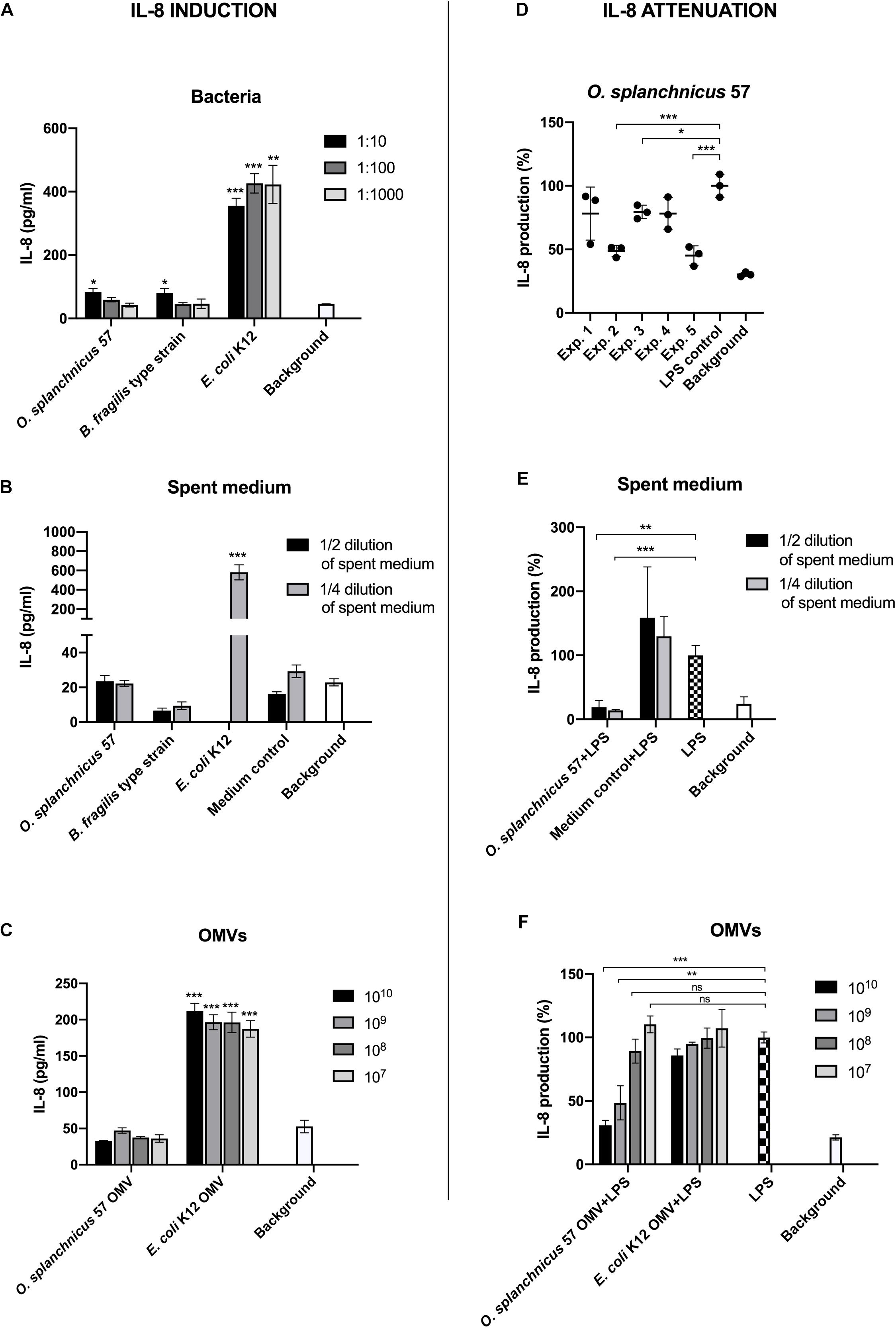
Figure 4. The induction of IL-8 production in HT-29 cells by O. splanchnicus 57 cells (A), spent medium (B), and OMVs (C). Representative results are shown as a mean and standard deviation of three technical replicates. In the section B, the result of 1/2 dilution of E. coli K12 spent medium is not shown due to excessive toxicity to the HT-29 cells. The medium control in the spent media experiments refers to GAM medium. An asterisk indicates a significant (* = p < 0.05, ** = p < 0.01, *** = p < 0.001) IL-8 production above the background level. The anti-inflammatory capacity of O. splanchnicus 57 cells (D), spent medium (E), and OMVs (F) as assessed by a decrease in LPS-induced IL-8 production in HT-29 cells. In section D, experiment refers to an individual biological experiment using a new passage of HT-29 cells. Representative results are shown as a mean and standard deviation of three technical replicates. The growth medium for HT-29 cells, McCoy 5A, was used as background control in all experiments. The medium control in the spent media experiments refers to GAM medium. * = p < 0.05, ** = p < 0.01, *** = p < 0.001, ns = not significant.
Subsequently, we also examined the anti-inflammatory capacity of O. splanchnicus 57 by assessing whether the bacterium could attenuate E. coli LPS –induced IL-8 production in HT-29 cells (Figure 4D). In three biological experiments out of five, incubation with O. splanchnicus 57 with HT-29 monolayer prior to LPS-induced inflammation significantly decreased the IL-8 release. In the two other experiments, the IL-8 levels after O. splanchnicus 57 treatment were also lower than LPS control, but the difference did not reach statistical significance. The culture supernatant of O. splanchnicus 57 drastically attenuated the LPS-induced IL-8 response to the background level with both, 1/2 and 1/4, dilutions (Figure 4E). Medium control, i.e., GAM broth, did not have an effect as the induced IL-8 levels were the same or higher than the LPS control. Interestingly, also O. splanchnicus 57 OMVs dose-dependently decreased the IL-8 production in LPS-induced HT-29 cells suggesting an anti-inflammatory capacity (Figure 4F). The decrease was significant only with the two highest concentrations, 1010 and 109 OMVs per well, which yielded a clear, 50% or more decrease as compared to the LPS control.
Discussion
In the present study, we addressed the immunomodulatory properties of the relatively abundant, but poorly characterized O. splanchnicus. A recent study about distinct microbial niches in the colonic mucosa of twelve transplant donors showed that Odoribacter did not exhibit colonizing preference between cecum, transverse colon and sigmoid colon (James et al., 2020). The abundance of Odoribacter varied from undetectable to 1.6% of the total bacterial load, which corresponds to normal microbiota variability between individuals. Due to the lack of previous studies on the characteristics of this fairly abundant bacterium, we decided to address its immunomodulatory properties. The draft genome of O. splanchnicus 57 lacked the lpxM gene needed for hexa-acylation of lipid A, the moiety that determines the endotoxicity of LPS based on the ligand affinity to TRL4-MD2 complex (Park et al., 2009; Han et al., 2013). Thus, O. splanchnicus potentially harbors only penta-acylated lipid A, which is 100-fold less toxic compared to the E. coli LPS (Park et al., 2009). Hexa-acylated E. coli lipid A contains six acyl chains and two phosphate groups activating the TLR4–Mal–MyD88 pathway, whereas penta-acylated lipid A signals through TLR4–TRAM–TRIF causing lower cytokine release (Park et al., 2009). Bacteroidetes species are major contributors of the penta-acylated LPS biosynthesis in a healthy gut, as 80–90% of the total produced LPS is of Bacteroidetes origin (d’Hennezel et al., 2017). It has been suggested that the immunoinhibitory activity of gut microbial LPS load in the gut could facilitate host-tolerance to microbes due to the silencing of TLR4 signaling (d’Hennezel et al., 2017). The similar, low-level NF-κB activity induced by O. splanchnicus 57 and penta-acylated B. fragilis LPS in the THP-1 reporter cell line support our previous hypothesis of O. splanchnicus 57 lipid A containing only five acyl chains (Weintraub et al., 1989). The LPS from O. splanchnicus 57 and B. fragilis also induced lower TNF-α/IL-10 ratios in human PBMCs compared to E. coli K12, but the effect was not statistically significant.
IL-10 is an essential anti-inflammatory cytokine for colon homeostasis and regulation of inflammation, whereas TNF-α mediates pro-inflammatory response (Kany et al., 2019). Recently, O. splanchnicus was found to correlate negatively with TNF-α production after LPS stimulation in a microbiome-cytokine interaction pattern study describing a connection between the abundance of a specific bacterial species in the gut and a high/low response of a certain cytokine (Schirmer et al., 2016). The microbiome could affect TNF-α production in a stimulus-dependent manner, as the microbial associations with TNF-α were only detected after LPS stimulation (Schirmer et al., 2016). There was no difference in our results regarding the effect of O. splanchnicus 57 cells and E. coli K12 on the ratio of TNF-α/IL-10 produced by human PBMCs. However, the spent medium of O. splanchnicus 57 did elicit a significant amount of IL-10 in relation to TNF-α, which indicated that the secreted bacterial molecules in the medium could have an anti-inflammatory impact on the host cells. A highly prevalent gut anaerobe, Faecalibacterium prausnitzii, and its culture supernatant decreased TNF-α and increased IL-10 secretion in mice with 2,4,6-trinitrobenzenesulphonic acid (TNBS)-induced colitis (Sokol et al., 2008). As O. splanchnicus is a known producer of SCFAs (Werner et al., 1975), these metabolites may have enhanced to some extent the upregulation of IL-10 production in PBMCs (Saemann et al., 2000; Cavaglieri et al., 2003; Chen et al., 2019). In a mouse colitis model, an induction of IL-10 upon FMT was observed, which was associated with the increased abundance of fecal SCFA-producing taxa, including Odoribacter (Burrello et al., 2018).
We found O. splanchnicus 57 cells to be non-adherent to HT-29 and Caco-2 cells or mucus. In addition, the bacterial cells did not have an effect on enterocyte monolayer integrity as measured by TER. These findings also insinuate that secreted molecules rather than the bacterial cells play a key role in the host-microbe interactions of O. splanchnicus. Many Bacteroides spp. have been shown in vitro to beneficially interact with enterocytes without cell-cell contact (Hiippala et al., 2020). Another well-studied example of closely related bacteria is B. fragilis, which produces an anti-inflammatory molecule, polysaccharide A (PSA), packed in OMVs and able to activate T-cell dependent immune responses in the host (Mazmanian et al., 2005; Shen et al., 2012). Besides SCFAs, very little is known about the effector molecules produced by O. splanchnicus. We hypothesized that O. splanchnicus 57 OMVs could transport beneficial effector molecules from the bacterium to the gut epithelium and tested this in an in vitro setting. We isolated and visualized O. splanchnicus 57 OMVs by TEM followed by assessing the anti-inflammatory capacity of the vesicles in vitro. O. splanchnicus 57 produced OMVs ubiquitously under normal, presumably non-stressed culture conditions.
Cytokine IL-8 is a neutrophil chemoattractant secreted by intestinal epithelial cells as a response to inflammatory stimuli, such as bacterial molecules (Lammers et al., 1994; Mitsuyama et al., 1994). Colonic IL-8 levels have been shown to correlate with the degree of intestinal inflammation and the number of neutrophils in the mucosa in UC and CD patients (Mitsuyama et al., 1994; Pearl et al., 2013). Undifferentiated HT-29 cells were used in the IL-8 assays due to the downregulation of IL-8 in LPS-induced differentiated HT-29 cells as well as due to the assay optimization in our previous studies using undifferentiated HT-29 cells (Böcker et al., 2000; Hiippala et al., 2016, 2020). However, gut epithelium consists of a cell population ranging from undifferentiated to fully differentiated enterocytes, and therefore bacterial anti-inflammatory activity should be studied also in vivo, ex vivo using tissue samples and/or gut organoid models to assess the response in an epithelium with enterocytes that have different maturation stages. First, we determined the IL-8 response in HT-29 cells induced by O. splanchnicus 57, its spent medium and OMVs. The bacterial cells or secreted molecules with different dilutions consistently triggered a cytokine production at a similar level as the background control or B. fragilis. Thus, O. splanchnicus 57 or its metabolites do not seem to provoke a substantial inflammatory reaction in the epithelium. Furthermore, O. splanchnicus 57 culture supernatant and OMVs clearly and consistently decreased the LPS-induced IL-8 release in HT-29 cells. Especially OMVs showed a dose-dependent attenuation capacity in enterocytes lowering the IL-8 response by over 50% with the two highest vesicle concentrations. To our knowledge, there are no previous studies on OMVs produced by O. splanchnicus and their immunoregulatory potential, which makes our findings novel in the field of gut microbiology.
Previously, the supernatant of F. prausnitzii, a highly abundant, butyrate-producing and anti-inflammatory bacterium in the healthy human intestinal mucosa and depleted in IBD patients (Sokol et al., 2008; Lopez-Siles et al., 2016; James et al., 2020), was found not to induce IL-8 secretion by Caco-2 cells, and on the other hand, to reduce IL-8 release from IL-1β-induced Caco-2 cells (Sokol et al., 2008). In addition, F. prausnitzii supernatant, but not the bacterial cells alone, strongly inhibited NF-κB activation by IL-1β in Caco-2 cells, which was not due to butyrate, as the effect could not be produced by SCFA (Sokol et al., 2008). More recently, the extracellular polymeric matrix and a specific 15 kDa protein have been found to contribute to the anti-inflammatory properties of F. prausnitzii (Rossi et al., 2015; Quevrain et al., 2016). Similarly, the effector molecules of O. splanchnicus need to be addressed in future studies. Our results with isolated OMVs from O. splanchnicus 57 show that the bacterium could exert anti-inflammatory and mucosal homeostasis-promoting effects in the gut, beyond the known beneficial health effects of SCFAs. We acknowledge that in vitro assays have limitations and should be considered as an early phase to explore the bacterium’s potential anti-inflammatory activity, which should be studied further by using more advanced in vitro and animal models.
Bacterial OMVs are known to carry a wide variety of bacterial molecules to the epithelium via the thick mucus layer that is physically inaccessible to bacterial cells and thus, to play a vital role in bacteria-host interactions (Lynch and Alegado, 2017). For example, an inflammation-silencing gut symbiont Bacteroides vulgatus has been shown to produce OMVs, which induce a tolerant semi-mature phenotype in CD11c + bone marrow-derived dendritic cells (Maerz et al., 2018). Furthermore, A. muciniphila-derived OMVs could attenuate the release of pro-inflammatory IL-6 from colon epithelial cells in vitro and decrease the severity of colitis in DSS-induced mouse model (Kang et al., 2013). OMVs produced by A. muciniphila also improved gut barrier integrity in vitro and metabolic functions in vivo (Chelakkot et al., 2018). Extracellular vesicles of F. prausnitzii upregulated anti-inflammatory cytokines and decreased proinflammatory cytokines in vitro (Jafari et al., 2019; Rabiei et al., 2019), whereas F. prausnitzii supernatant ameliorated colitis in mice models (Miquel et al., 2015; Huang et al., 2016). Concerning the possible effector molecules carried by OMVs, for example bacterial sphingolipids are also likely to be transported in OMVs through the mucus layer to the intestinal epithelium (Heaver et al., 2018). Sulfobacin B, a type of sulfonolipid produced by O. splanchnicus, suppressed LPS-induced inflammatory responses in vitro and in vivo in a murine model of colitis by inhibiting dose-dependently LPS-stimulated production of TNF-α in cultured mouse macrophages (Maeda et al., 2010).
In summary, we isolated O. splanchnicus strain 57 from a stool batch of healthy FMT donor, whose recipients were effectively treated for recurrent Clostridioides difficile infection. Our in vitro findings implicate O. splanchnicus as a non-adherent gut commensal with innocuous impact on the epithelial monolayer integrity. The genome data and in vitro assays implicate that O. splanchnicus possibly harbors a less toxic, penta-acylated LPS inducing similar effect as B. fragilis. The novel O. splanchnicus fecal isolate 57 produces OMVs under normal growth conditions as visualized by TEM. The bacterium, its culture supernatant or OMVs did not induce an IL-8 response in enterocytes indicating a low proinflammatory capacity. On the contrary, O. splanchnicus 57 and its effector molecules elicited in vitro anti-inflammatory action in enterocytes, as the bacterial cells, spent medium and OMVs decreased E. coli LSP-induced IL-8 production in HT-29 cells. The effect was especially robust with O. splanchnicus culture supernatant and OMVs, indicating that the bacterium’s epithelial interactions do not require cell-cell contact. The beneficial immunoregulatory properties of secreted bacterial molecules and their impact on gut homeostasis is an exciting, thus far relatively unexplored domain in the microbiome research. Further studies are warranted to identify the potential inflammation-ameliorating, OMV-transported effector molecules of O. splanchnicus.
Materials and Methods
Isolation and Identification of O. splanchnicus Isolate 57
Odoribacter splanchnicus isolate 57 was isolated from the feces of a healthy, pre-screened FMT donor as described in our previous study (Hiippala et al., 2020). The use of the donor sample was approved by the Ethics Committee of Hospital District of Helsinki and Uusimaa Finland (DnroHUS124/13/03/01/11). The donor, described in a previous study (Jalanka et al., 2016), provided a written informed consent. In short, the frozen banked fecal solution (saline-10% glycerol) was thawed, serially diluted in PBS and cultivated on Gifu anaerobic medium (GAM; Nissui Pharmaceutical Co., Ltd., Japan) for 48 h under anaerobic conditions (Concept Plus anaerobic workstation, Ruskinn Technology Ltd., Bridgend, United Kingdom) at 37°C. Separate colonies were picked and re-streaked on new agar plates until they were purified. Gram-staining and microscopy were used to evaluate the purity of the isolate followed by partial 16S rRNA gene sequencing for tentative identification. Bacterial mass from the isolate was resuspended in TE buffer (10 mM Tris–HCl, 1 mM EDTA, pH 8), heated to 95°C for 15 min to break the cells and used as PCR template. PCR amplification of the partial 16S rRNA gene was carried out using 27F DegL (5′-AGR GTT YGA TYM TGG CTC AG-3′) forward and Pd (5′-GTA TTA CCG CGG CTG CTG-3′) reverse primers. Sanger sequencing of the PCR product using the forward primer was carried out in the Institute of Biotechnology core facility, University of Helsinki. The partial 16S rRNA gene sequence was compared to NCBI 16S ribosomal RNA sequences database using BLASTn to acquire a genus-level identification.
Genome Sequencing
Genomic DNA was extracted from O. splanchnicus 57 cell pellet with DNeasy Blood and Tissue Kit (Qiagen, Hilden, Germany) following the manufacturer’s protocol for Gram-negative bacteria and fragmented to approximately 500 bp using a Q800R2 Sonicator (QSonica, Newtown, CT, United States). Genome libraries were prepared for paired end sequencing using the NEBNext® UltraTM II Kit (New England Biolabs, Ipswich, MA, United States) and quantification was carried out using the Library Quantification Kit (KAPA Biosystems, Waltham, MA, United States). Libraries were pooled in equimolar amounts and sequenced on the MiSeq (Illumina, Inc., San Diego, CA, United States). Short read data were assembled using unmanned genome assembly pipeline (UGAP;1) using the SPAdes genome assembler. The species of each genome was identified with Kraken (Wood and Salzberg, 2014). Read data were deposited in the NCBI SRA under BioProject PRJNA575760. Genomic comparison to O. splanchnicus type strain 1651/6T genome (NC_015160.1) and screening of the genes that encode enzymes for Kdo2-lipid A modification we carried out using BLAST (Basic Local Alignment Search Tool, BLASTn algorithm).
Other Bacterial Strains and Growth Conditions
Bacteroides fragilis type strain E-022248T (= DSM 2151 = ATCC 25285) from the VTT Culture Collection (VTT Technical Research Center of Finland) was grown under anaerobic conditions for 2 days at 37°C on Brucella agar with hemin and vitamin K (Sigma Aldrich, St. Louis, MO, United States) along with 5% defibrinated sheep blood (Bio Karjalohja Oy, Finland). Escherichia coli K12-derived TOP10 (Invitrogen, United States) was aerobically cultivated overnight in Luria–Bertani broth (Becton Dickinson, United States).
Epithelial Cell Lines
The human colonic epithelial cell lines Caco-2 (ACC 169) and HT-29 (ACC 299) were obtained from the German Collection of Microorganisms and Cell Cultures (DSMZ). Cell lines were grown at 37°C in an incubator under an oxic atmosphere with 5% CO2 and passaged after reaching 80% confluence (approximately every 3–4 days) using TryplExpress (Lonza, United States) to detach the cells. Passages 6–28 were used in the experiments. HT-29 cells were cultivated in McCoy 5A (Lonza, Belgium) medium containing 10% heat-inactivated (30 min at 56°C) fetal bovine serum (FBS; Gibco) and 100 U ml–1 PEST. Caco-2 cells were grown in RPMI 1640 medium (Sigma-Aldrich, United States) supplemented with 20% FBS, non-essential amino acids (1%, NEAA; Lonza, Belgium), 15 mM HEPES (Lonza, Belgium), 100 U ml-1 penicillin and streptomycin (PEST; Lonza, Belgium) and 2 mM L-glutamine (Lonza, Belgium). Human THP-1 dual reporter monocytic cell line (thpd-nfis, Invivogen, United States) were cultured at 1 × 105 cells/ml in 96-well plates in RPMI 1640 (Gibco, United States) supplemented with 10% fetal bovine serum (Gibco) and 100 U ml–1 penicillin and streptomycin.
Adhesion to HT-29, Caco-2 and Mucus
The adherence of O. splanchnicus isolate 57 to Caco-2 and HT-29 cell lines (8 days post-plating) and mucus was studied as previously described (Kainulainen et al., 2013; Reunanen et al., 2015). The bacterium was grown in GAM medium supplemented with 10 μl ml–1 of [6′-3H]thymidine (17,6 Ci mmol–1, Perkin Elmer, United States) to metabolically radiolabel the cells. Six technical replicates (parallel wells) were used in each experiment. To assess the adherence to intestinal mucus, porcine mucus (Sigma-Aldrich, 50 μg well-1 in PBS) was allowed to absorb to Maxisorp microtiter plate wells overnight at 4°C. 10,000 Caco-2 or HT-29 cells per well were seeded onto 96-well microplate. [3H]Thymidine-labeled O. splanchnicus isolate 57 cells were washed with an appropriate medium (McCoy 5A for HT-29 cells, RPMI 1640 for Caco-2 cells and PBS for the mucus assay) without the supplements and adjusted to OD600nm 0.25 corresponding to approximately 108 cells/ml. After 1 h of incubation on the epithelial cell monolayer or mucus at 37°C in a CO2 incubator, the bacterial suspensions were removed, and wells were washed three times to remove the non-adherent bacteria. Adhered bacteria were lysed with 1% SDS-0.1 M NaOH solution and radioactivity was measured with a liquid scintillator (Wallac Winspectral 1414, Perkin-Elmer, Waltham, MA, United States). The percentage of bound bacteria was calculated relative to the radioactivity of the bacterial suspension initially added to the wells.
Effect on Caco-2 Monolayer Integrity
Caco-2 cell line is suitable for the monolayer integrity studies measuring TER due to the cells’ enterocytic differentiation and expression of intercellular junctional complexes (Hidalgo et al., 1989; Klingberg et al., 2005; Hsieh et al., 2015). The effect of O. splanchnicus 57 isolate on the TER of Caco-2 monolayer was studied as previously described (Kainulainen et al., 2015; Reunanen et al., 2015). Briefly, 50,000 Caco-2 cells were seeded on PET inserts with a pore size of 3 μm (Sarstedt, Nümbrecht, Germany) and grown for 8 days. The medium in the well was changed every 3 days. E. coli TOP10 was used as a negative control in the experiments due to its detrimental effect on monolayer integrity (Reunanen et al., 2015). O. splanchnicus 57 was washed with RPMI medium including the supplements, adjusted to OD600nm 0.25 and 100 μl of bacterial suspension was added onto cell monolayer. The TER was measured after 0 and 24 h using an EVOM epithelial voltmeter with an electrode (World Precision Instruments, United Kingdom). The blank resistance (measurement at time point 0) was subtracted from the measurements made after 24 h of incubation, and the unit area resistance (Ω cm2) was calculated by multiplying the tissue resistance values by the surface area of the filter membrane. The change in TER during the 24 h was calculated comparing the samples to the medium control.
LPS Purification by the Chloroform-Methanol Method
Odoribacter splanchnicus 57, B. fragilis and E. coli K12 were grown in an appropriate culture broth. Cells were harvested by centrifugation (4,000 rpm, 10 min) and resuspended in single-phase Bligh and Dyer mixture consisting of chloroform/methanol/water (1:2:0.8 v/v) to remove phospholipids (Vatanen et al., 2016). After 20 min of incubation at room temperature, the suspension was centrifuged, and the cell pellet was washed twice with Bligh and Dyer mixture and the remaining LPS preparation was suspended in PBS. The yielded LPS concentration was quantified using the chromogenic LAL assay (Thermofisher, United States), by following the manufacturer’s instructions.
LPS-Induced Activation of NF-κB in THP-1 Cell Line
Following overnight incubation, THP-1 dual reporter cells were induced to M0 macrophage differentiation with 15 ng/ml PMA for 24 h. Upon differentiation, cells were stimulated for 24 h with 10 ng/ml LPS from different bacterial strains: O. splanchnicus 57; B. fragilis type strain; E. coli K12 (tlrl-eklps InvivoGen; United States), smooth LPS from E. coli 0111:B4 (tlrl-eblps, Invivogen; United States), semi-rought LPS from E. coli R515 and rough LPS from E. coli J5 (IAX-100-007-M001 and 014-M001, respectively, Adipogen; United States). PBS was used as a control. Following 24-h stimulation, the supernatant was collected for the measurement of NF-κB activity.
THP-1 dual cell line NF-κB activity was measured according to the manufacturer’s instructions (InvivoGen; United States). Briefly, the reporter cells have a stable integrator secreted alkaline phosphatase (SEAP) reporter gene for monitoring nuclear factor (NF)-κB activation. Upon NF-κB activation, the SEAP reporter gene is activated, leading to the secretion of alkaline phosphatase, which is then quantifiable by a colorimetric assay (QUANTI-Blue, InvivoGen; United States) using a microplate reader (FluoStar Omega, BMG Labtech; Germany) with absorbance set for 630 nm.
Peripheral Blood Mononuclear Cell Isolation
Buffy coat blood from healthy donors were obtained from the IRCCS Policlinico San Matteo, Pavia, Italy. PBMCs were isolated using density gradient centrifugation (Ficoll-Paque Plus, GE Healthcare) according to the manufacturer’s instructions. Briefly, blood sample was diluted with DPBS and layered on Ficoll-Paque Plus. After centrifugation (300 g, 30 min), the separated mononuclear cells were collected, resuspended in DPBS and washed 3 times with DBPS to remove the platelets. Cells were resuspended in DPBS + 0.5% BSA + 2mM EDTA and counted.
TNF-α/IL-10 Released From Human PBMCs After Co-Incubation With Odoribacter LPS, Odoribacter Cells and Spent Medium
PBMCs were added to 48-well plates (5,00,000 cells per well) in DMEM medium (ThermoFisher Sci.) supplemented with 10% FBS. O. splanchnicus 57, B. fragilis and E. coli K12 LPS preparations were diluted to 1 ng/ml in DMEM + 10% FBS. O. splanchnicus 57 was grown in GAM broth under anaerobic conditions for 2 days. The bacterial culture was centrifuged (10, 000 rpm, 3 min) and the cell pellet was washed with DMEM + 10% FBS, adjusted to OD600nm 0.25 and 50 μl of this suspension was added onto PBMCs. DMEM + 10% FBS was used as background control. For the experiments with O. splanchnicus 57 spent medium, the bacterial suspension was adjusted to OD600nm 1.0, centrifuged (16 000 rpm, 3 min) and the supernatant was filtered with 0.2 μm filter. 40 μl of the spent medium preparation (5%) was added onto PBMCs. Heat-killed E. coli K12 MG1655 (108 cells/ml) and the bacterial growth medium (GAM) were used as controls in the experiments with bacterial cells and spent medium. PBMCs were incubated with LPS, bacterial cells or spent medium for 24 h and the supernatants were collected for cytokine analysis. TNF-α and IL-10 (hIL-10 JES3-19F1 clone; hTNF-a MAb11 clone, Biolegend, United States) were measured by using ELISA assay according to the manufacturer’s instructions. Unstimulated PBMCs were used as control in all experiments. In the LPS stimulation, the results were calculated as a ratio of TNF-α/IL-10 using PBMCs isolated from the buffy coats of four different donors. In the experiment of O. splanchnicus 57 spent medium and bacterial cell stimulation, the TNF-α/IL-10 ratio was calculated as a mean and standard deviation of three or four experiments by using PMBCs isolated from buffy coats of three or four donors, respectively.
OMV Isolation
Outer membrane vesicles isolation from 400 ml of O. splanchnicus 57 and E. coli K12 broth culture was carried out as previously described (Elhenawy et al., 2014) with modifications by pelleting the bacteria (centrifugation 4000 rpm for 10 min), filtering the supernatant with 0.2 μm filter to remove any remaining bacteria and concentrating the filtered supernatant by using Amicon 100 kDa filters (Millipore). The concentrated supernatant was ultracentrifuged (Beckman Coulter) for 2 h at 32,000 rpm. The remaining OMV pellet was resuspended in PBS and ultracentrifuged (2 h, 32,000 rpm) again. The isolated OMVs were suspended in PBS for nanoparticle tracking analysis (NTA) for quantification and electron microscopy (EM).
Sample Preparation and Transmission Electron Microscopy
OMVs and bacterial samples were prepared for electron microscopy (EM) by loading to carbon coated and glow discharged 200 mesh copper grids with pioloform support membrane (Puhka et al., 2017). Samples were fixed with 2.0% PFA in NaPO4 buffer, stained with 2% neutral uranyl acetate, further stained and embedded in uranyl acetate and methyl cellulose mixture (1.8/0.4%). OMVs and bacterial cells were viewed with transmission EM using Jeol JEM-1400 (Jeol Ltd., Tokyo, Japan) operating at 80 kV. Images were taken with Gatan Orius SC 1000B CCD-camera (Gatan Inc., United States) with 4008 × 2672 px image size and no binning.
The Induction of IL-8 Production in HT-29 Cells
The measurement of IL-8 response in 8 days post-plating HT-29 cells by bacterial cells, spent medium and OMVs was carried out as previously described (Kainulainen et al., 2013). In brief, the bacterial suspensions were washed with McCoy 5A medium supplemented with 10% FBS and adjusted to OD600nm 0.25. Bacteria were diluted to 1:10, 1:100, and 1:1,000 and incubated on the HT-29 cells for 3 h at 37°C in a CO2 incubator. Spent media from broth cultures of O. splanchnicus 57, B. fragilis type strain and E. coli K12 were prepared by adjusting the bacterial culture to OD600nm 1.0, pelleting the bacteria by centrifugation and filtering the supernatant through 0.2 μm filter to remove any remaining cells. Spent medium preparation was diluted to 1:4 and 1:2 using McCoy 5A medium with supplements. Bacterial growth medium (GAM) diluted with McCoy 5A medium was used as control. OMVs were diluted to McCoy 5A with supplements and added on monolayers using 1010, 109, 108, and 107 concentrations. Three technical replicates (parallel wells) were used in each experiment. OptEIA Human IL-8 ELISA kit (BD Biosciences, United States) was used according to the manufacturer’s instructions to measure the concentration of the chemokine in the culture media.
Anti-Inflammatory Capacity
IL-8 assays were only performed using the HT-29 cell line, because Caco-2 cells are known to be unresponsive to LPS stimulation possibly due to defects in TLR4 signaling (Funda et al., 2001; Van De Walle et al., 2010; Hsu et al., 2011). The capacity of O. splanchnicus 57 isolate or its effector molecules to attenuate LPS-induced IL-8 production in HT-29 cells was measured as previously described (Kainulainen et al., 2015; Hiippala et al., 2020). In brief, HT-29 cells were seeded 10,000 cells per well onto 96-well microplates for the attenuation assay. Broth culture of O. splanchnicus 57 was washed once with McCoy 5A medium supplemented with FBS (10%) and adjusted to OD600nm 0.25 using the same medium. 100 μl of bacterial suspension was added onto 8 days old HT-29 cells and incubated at 37°C for 1 h under oxic atmosphere with 5% CO2. Spent medium and OMV dilutions were prepared as in the experiments measuring IL-8 release. McCoy 5A medium containing 10% FBS without LPS was used as the background control. In the experiments with spent medium, GAM broth with LPS was used as medium control. Next, the bacterial suspension, spent medium or OMVs were removed from the HT-29 monolayer and 200 μl McCoy 5A medium with 1 ng/ml of E. coli LPS (Sigma) was added. After a 4-h incubation with LPS, supernatants were collected and IL-8 levels were measured by using an ELISA assay (BD OptEIATM Set). The IL-8 levels were calculated using four parametric logistic curves. The attenuation capacity of the isolate, spent medium and OMVs were assessed by comparing the LPS-induced IL-8 production (%) of the sample to that of the LPS control (100%). The experiment with O. splanchnicus 57 bacterial cells was repeated five times.
Statistical Testing
All the experiments were repeated two to five times (biological replicates) depending on the assay to confirm the results. An unpaired t-test was used to determine significant differences between two groups, such as the sample and the control. Homoscedasticity testing was performed with Levene’s test to identify equal or unequal variances. All statistical analyses were carried out with GraphPad Prism 8.4.1 (GraphPad Software, United States). A p-value of <0.05 was considered statistically significant.
Data Availability Statement
The datasets presented in this study can be found in online repositories. The names of the repository/repositories and accession number(s) can be found below: https://www.ncbi.nlm.nih.gov/, PRJNA575760.
Ethics Statement
The studies involving human participants were reviewed and approved by the Ethics Committee of Hospital District of Helsinki and Uusimaa Finland (DnroHUS124/13/03/01/11). The patients/participants provided their written informed consent to participate in this study.
Author Contributions
RS and KH conceptualization. RS, KH, GB, CB, FF, VK, and JB methodology. KH, GB, CB, AD-B, MS, JB, and VK validation. KH, DL, and JB formal analysis. KH and RS writing—original draft preparation. KH and GB writing—review and editing, all authors, and visualization. RS, FF, KE, and DE supervision. RS, KH, and VK funding acquisition. All authors have read and agreed to the published version of the manuscript.
Funding
This research was funded by the Sigrid Juselius Foundation, FI (Senior Researcher’s grant for RS), Päivikki and Sakari Sohlberg Foundation for RS, the Doctoral Program in Microbiology and Biotechnology, University of Helsinki, FI and Emil Aaltonen Foundation funding for KH, and Mary och Georg C. Ehrnrooths stiftelse for VK.
Conflict of Interest
The authors declare that the research was conducted in the absence of any commercial or financial relationships that could be construed as a potential conflict of interest.
Acknowledgments
We would like to thank Erika Mileti (European Institute of Oncology, Milan, Italy) and Katariina Nurmi (Translational Immunology Research Program, University of Helsinki) for technical assistance in the laboratory work. We would also like to acknowledge the work done by Extracellular Vesicle Core [Department of Biosciences and the Faculty of Pharmacy and the Institute for Molecular Medicine Finland (FIMM)] and Electron Microscopy Unit (Institute of Biotechnology, University of Helsinki).
Supplementary Material
The Supplementary Material for this article can be found online at: https://www.frontiersin.org/articles/10.3389/fmicb.2020.575455/full#supplementary-material
Footnotes
References
Böcker, U., Schottelius, A., Watson, J. M., Holt, L., Licato, L. L., Brenner, D. A., et al. (2000). Cellular differentiation causes a selective down-regulation of interleukin (IL)-1beta-mediated NF-kappaB activation and IL-8 gene expression in intestinal epithelial cells. J. Biol. Chem. 275, 12207–12213. doi: 10.1074/jbc.275.16.12207
Brahe, L. K., Le Chatelier, E., Prifti, E., Pons, N., Kennedy, S., Hansen, T., et al. (2015). Specific gut microbiota features and metabolic markers in postmenopausal women with obesity. Nutr. Diabetes 5:e159. doi: 10.1038/nutd.2015.9
Brown, E. M., Ke, X., Hitchcock, D., Jeanfavre, S., Avila-Pacheco, J., Nakata, T., et al. (2019). Bacteroides-derived sphingolipids are critical for maintaining intestinal homeostasis and symbiosis. Cell Host Microbe 25, 668–680.e7. doi: 10.1016/j.chom.2019.04.002
Burke, D. G., Fouhy, F., Harrison, M. J., Rea, M. C., Cotter, P. D., O’Sullivan, O., et al. (2017). The altered gut microbiota in adults with cystic fibrosis. BMC Microbiol. 17:58. doi: 10.1186/s12866-017-0968-8
Burrello, C., Garavaglia, F., Cribiù, F. M., Ercoli, G., Lopez, G., Troisi, J., et al. (2018). Therapeutic faecal microbiota transplantation controls intestinal inflammation through IL10 secretion by immune cells. Nat. Commun. 9:5184. doi: 10.1038/s41467-018-07359-8
Cavaglieri, C. R., Nishiyama, A., Fernandes, L. C., Curi, R., Miles, E. A., and Calder, P. C. (2003). Differential effects of short-chain fatty acids on proliferation and production of pro- and anti-inflammatory cytokines by cultured lymphocytes. Life Sci. 73, 1683–1690. doi: 10.1016/s0024-3205(03)00490-9
Chelakkot, C., Choi, Y., Kim, D. K., Park, H. T., Ghim, J., Kwon, Y., et al. (2018). Akkermansia muciniphila-derived extracellular vesicles influence gut permeability through the regulation of tight junctions. Exp. Mol. Med. 50:e450. doi: 10.1038/emm.2017.282
Chen, L., Sun, M., Wu, W., Yang, W., Huang, X., Xiao, Y., et al. (2019). Microbiota metabolite butyrate differentially regulates Th1 and Th17 cells’ differentiation and function in induction of colitis. Inflamm. Bowel Dis. 25, 1450–1461. doi: 10.1093/ibd/izz046
d’Hennezel, E., Abubucker, S., Murphy, L. O., and Cullen, T. W. (2017). Total lipopolysaccharide from the human gut microbiome silences toll-like receptor signaling. mSystems 2:e00046-17. doi: 10.1128/mSystems.00046-17
Elhenawy, W., Debelyy, M. O., and Feldman, M. F. (2014). Preferential packing of acidic glycosidases and proteases into Bacteroides outer membrane vesicles. mBio 5:e00909-14. doi: 10.1128/mBio.00909-14
Etxeberria, U., Hijona, E., Aguirre, L., Milagro, F. I., Bujanda, L., Rimando, A. M., et al. (2017). Pterostilbene-induced changes in gut microbiota composition in relation to obesity. Mol. Nutr. Food Res. 61:1500906. doi: 10.1002/mnfr.201500906
Funda, D. P., Tuckova, L., Farre, M. A., Iwase, T., Moro, I., and Tlaskalova-Hogenova, H. (2001). CD14 is expressed and released as soluble CD14 by human intestinal epithelial cells in vitro: lipopolysaccharide activation of epithelial cells revisited. Infect. Immun. 69, 3772–3781. doi: 10.1128/iai.69.6.3772-3781.2001
Göker, M., Gronow, S., Zeytun, A., Nolan, M., Lucas, S., Lapidus, A., et al. (2011). Complete genome sequence of Odoribacter splanchnicus type strain (1651/6). Stand. Genomic Sci. 4, 200–209. doi: 10.4056/sigs.1714269
Gomez-Arango, L. F., Barrett, H. L., McIntyre, H. D., Callaway, L. K., Morrison, M., and Dekker Nitert, M. (2016). Increased systolic and diastolic blood pressure is associated with altered gut microbiota composition and butyrate production in early pregnancy. Hypertension 68, 974–981. doi: 10.1161/hypertensionaha.116.07910
Granado-Serrano, A. B., Martin-Gari, M., Sanchez, V., Riart Solans, M., Berdun, R., Ludwig, I. A., et al. (2019). Faecal bacterial and short-chain fatty acids signature in hypercholesterolemia. Sci. Rep. 9:1772. doi: 10.1038/s41598-019-38874-3
Han, Y., Li, Y., Chen, J., Tan, Y., Guan, F., and Wang, X. (2013). Construction of monophosphoryl lipid A producing Escherichia coli mutants and comparison of immuno-stimulatory activities of their lipopolysaccharides. Mar. Drugs 11, 363–376. doi: 10.3390/md11020363
Hardham, J. M., King, K. W., Dreier, K., Wong, J., Strietzel, C., Eversole, R. R., et al. (2008). Transfer of Bacteroides splanchnicus to Odoribacter gen. nov. as Odoribacter splanchnicus comb. nov., and description of Odoribacter denticanis sp. nov., isolated from the crevicular spaces of canine periodontitis patients. Int. J. Syst. Evol. Microbiol. 58(Pt 1), 103–109. doi: 10.1099/ijs.0.63458-0
Heaver, S. L., Johnson, E. L., and Ley, R. E. (2018). Sphingolipids in host-microbial interactions. Curr. Opin. Microbiol. 43, 92–99. doi: 10.1016/j.mib.2017.12.011
Hickey, C. A., Kuhn, K. A., Donermeyer, D. L., Porter, N. T., Jin, C., Cameron, E. A., et al. (2015). Colitogenic Bacteroides thetaiotaomicron antigens access host immune cells in a sulfatase-dependent manner via outer membrane vesicles. Cell Host Microbe 17, 672–680. doi: 10.1016/j.chom.2015.04.002
Hidalgo, I. J., Raub, T. J., and Borchardt, R. T. (1989). Characterization of the human colon carcinoma cell line (Caco-2) as a model system for intestinal epithelial permeability. Gastroenterology 96, 736–749. doi: 10.1016/0016-5085(89)90897-4
Hiippala, K., Kainulainen, V., Kalliomäki, M., Arkkila, P., and Satokari, R. (2016). Mucosal prevalence and interactions with the epithelium indicate commensalism of Sutterella spp. Front. Microbiol. 7:1706. doi: 10.3389/fmicb.2016.01706
Hiippala, K., Kainulainen, V., Suutarinen, M., Heini, T., Bowers, J. R., Jasso-Selles, D., et al. (2020). Isolation of anti-inflammatory and epithelium reinforcing Bacteroides and Parabacteroides Spp. from a healthy fecal donor. Nutrients 12:935. doi: 10.3390/nu12040935
Hod, K., Dekel, R., Aviv Cohen, N., Sperber, A., Ron, Y., Boaz, M., et al. (2018). The effect of a multispecies probiotic on microbiota composition in a clinical trial of patients with diarrhea-predominant irritable bowel syndrome. Neurogastroenterol. Motil. 30:e13456. doi: 10.1111/nmo.13456
Hsieh, C. Y., Osaka, T., Moriyama, E., Date, Y., Kikuchi, J., and Tsuneda, S. (2015). Strengthening of the intestinal epithelial tight junction by Bifidobacterium bifidum. Physiol. Rep. 3:e12327. doi: 10.14814/phy2.12327
Hsu, R. Y., Chan, C. H., Spicer, J. D., Rousseau, M. C., Giannias, B., Rousseau, S., et al. (2011). LPS-induced TLR4 signaling in human colorectal cancer cells increases beta1 integrin-mediated cell adhesion and liver metastasis. Cancer Res. 71, 1989–1998. doi: 10.1158/0008-5472.can-10-2833
Huang, X. L., Zhang, X., Fei, X. Y., Chen, Z. G., Hao, Y. P., Zhang, S., et al. (2016). Faecalibacterium prausnitzii supernatant ameliorates dextran sulfate sodium induced colitis by regulating Th17 cell differentiation. World J. Gastroenterol. 22, 5201–5210. doi: 10.3748/wjg.v22.i22.5201
Jafari, B., Khavari Nejad, R. A., Vaziri, F., and Siadat, S. D. (2019). Evaluation of the effects of extracellular vesicles derived from Faecalibacterium prausnitzii on lung cancer cell line. Biologia 74, 889–898. doi: 10.2478/s11756-019-00229-8
Jalanka, J., Mattila, E., Jouhten, H., Hartman, J., de Vos, W. M., Arkkila, P., et al. (2016). Long-term effects on luminal and mucosal microbiota and commonly acquired taxa in faecal microbiota transplantation for recurrent Clostridium difficile infection. BMC Med. 14:155. doi: 10.1186/s12916-016-0698-z
James, K. R., Gomes, T., Elmentaite, R., Kumar, N., Gulliver, E. L., King, H. W., et al. (2020). Distinct microbial and immune niches of the human colon. Nat. Immunol. 21, 343–353. doi: 10.1038/s41590-020-0602-z
Kainulainen, V., Reunanen, J., Hiippala, K., Guglielmetti, S., Vesterlund, S., Palva, A., et al. (2013). BopA does not have a major role in the adhesion of Bifidobacterium bifidum to intestinal epithelial cells, extracellular matrix proteins, and mucus. Appl. Environ. Microbiol. 79, 6989–6997. doi: 10.1128/aem.01993-13
Kainulainen, V., Tang, Y., Spillmann, T., Kilpinen, S., Reunanen, J., Saris, P. E., et al. (2015). The canine isolate Lactobacillus acidophilus LAB20 adheres to intestinal epithelium and attenuates LPS-induced IL-8 secretion of enterocytes in vitro. BMC Microbiol. 15:4. doi: 10.1186/s12866-014-0337-9
Kang, C. S., Ban, M., Choi, E. J., Moon, H. G., Jeon, J. S., Kim, D. K., et al. (2013). Extracellular vesicles derived from gut microbiota, especially Akkermansia muciniphila, protect the progression of dextran sulfate sodium-induced colitis. PLoS One 8:e76520. doi: 10.1371/journal.pone.0076520
Kany, S., Vollrath, J. T., and Relja, B. (2019). Cytokines in inflammatory disease. Int. J. Mol. Sci. 20:6008. doi: 10.3390/ijms20236008
Klingberg, T. D., Pedersen, M. H., Cencic, A., and Budde, B. B. (2005). Application of measurements of transepithelial electrical resistance of intestinal epithelial cell monolayers to evaluate probiotic activity. Appl. Environ. Microbiol. 71, 7528–7530. doi: 10.1128/aem.71.11.7528-7530.2005
Koh, A., De Vadder, F., Kovatcheva-Datchary, P., and Backhed, F. (2016). From dietary fiber to host physiology: short-chain fatty acids as key bacterial metabolites. Cell 165, 1332–1345. doi: 10.1016/j.cell.2016.05.041
Lai, Z. L., Tseng, C. H., Ho, H. J., Cheung, C. K. Y., Lin, J. Y., Chen, Y. J., et al. (2018). Fecal microbiota transplantation confers beneficial metabolic effects of diet and exercise on diet-induced obese mice. Sci. Rep. 8:15625. doi: 10.1038/s41598-018-33893-y
Lammers, K. M., Jansen, J., Bijlsma, P. B., Ceska, M., Tytgat, G. N., Laboisse, C. L., et al. (1994). Polarised interleukin 8 secretion by HT 29/19A cells. Gut 35, 338–342. doi: 10.1136/gut.35.3.338
Lewis, J. D., Chen, E. Z., Baldassano, R. N., Otley, A. R., Griffiths, A. M., Lee, D., et al. (2015). Inflammation, antibiotics, and diet as environmental stressors of the gut microbiome in pediatric crohn’s disease. Cell Host Microbe 18, 489–500. doi: 10.1016/j.chom.2015.09.008
Li, F., Sun, G., Wang, Z., Wu, W., Guo, H., Peng, L., et al. (2018). Characteristics of fecal microbiota in non-alcoholic fatty liver disease patients. Sci. China Life Sci. 61, 770–778. doi: 10.1007/s11427-017-9303-9
Lim, M. Y., You, H. J., Yoon, H. S., Kwon, B., Lee, J. Y., Lee, S., et al. (2017). The effect of heritability and host genetics on the gut microbiota and metabolic syndrome. Gut 66, 1031–1038. doi: 10.1136/gutjnl-2015-311326
Lopez-Siles, M., Martinez-Medina, M., Suris-Valls, R., Aldeguer, X., Sabat-Mir, M., Duncan, S. H., et al. (2016). Changes in the abundance of Faecalibacterium prausnitzii phylogroups I and II in the intestinal mucosa of inflammatory bowel disease and patients with colorectal cancer. Inflamm. Bowel Dis. 22, 28–41. doi: 10.1097/mib.0000000000000590
Lynch, J. B., and Alegado, R. A. (2017). Spheres of hope, packets of doom: the good and bad of outer membrane vesicles in interspecies and ecological dynamics. J. Bacteriol. 199:e00012-17. doi: 10.1128/jb.00012-17
Maeda, J., Nishida, M., Takikawa, H., Yoshida, H., Azuma, T., Yoshida, M., et al. (2010). Inhibitory effects of sulfobacin B on DNA polymerase and inflammation. Int. J. Mol. Med. 26, 751–758. doi: 10.3892/ijmm_00000522
Maerz, J. K., Steimle, A., Lange, A., Bender, A., Fehrenbacher, B., and Frick, J. S. (2018). Outer membrane vesicles blebbing contributes to B. vulgatus mpk-mediated immune response silencing. Gut Microbes 9, 1–12. doi: 10.1080/19490976.2017.1344810
Mazmanian, S. K., Liu, C. H., Tzianabos, A. O., and Kasper, D. L. (2005). An immunomodulatory molecule of symbiotic bacteria directs maturation of the host immune system. Cell 122, 107–118. doi: 10.1016/j.cell.2005.05.007
Miquel, S., Leclerc, M., Martin, R., Chain, F., Lenoir, M., Raguideau, S., et al. (2015). Identification of metabolic signatures linked to anti-inflammatory effects of Faecalibacterium prausnitzii. mBio 6:e00300-15. doi: 10.1128/mBio.00300-15
Mitsuyama, K., Toyonaga, A., Sasaki, E., Watanabe, K., Tateishi, H., Nishiyama, T., et al. (1994). IL-8 as an important chemoattractant for neutrophils in ulcerative colitis and Crohn’s disease. Clin. Exp. Immunol. 96, 432–436. doi: 10.1111/j.1365-2249.1994.tb06047.x
Morgan, X. C., Tickle, T. L., Sokol, H., Gevers, D., Devaney, K. L., Ward, D. V., et al. (2012). Dysfunction of the intestinal microbiome in inflammatory bowel disease and treatment. Genome Biol. 13:R79. doi: 10.1186/gb-2012-13-9-r79
Munoz, R., Rossello-Mora, R., and Amann, R. (2016). Revised phylogeny of Bacteroidetes and proposal of sixteen new taxa and two new combinations including Rhodothermaeota phyl. nov. Syst. Appl. Microbiol. 39, 281–296. doi: 10.1016/j.syapm.2016.04.004
Nagai, F., Morotomi, M., Watanabe, Y., Sakon, H., and Tanaka, R. (2010). Alistipes indistinctus sp. nov. and Odoribacter laneus sp. nov., common members of the human intestinal microbiota isolated from faeces. Int. J. Syst. Evol. Microbiol. 60(Pt 6), 1296–1302. doi: 10.1099/ijs.0.014571-0
Parada Venegas, D., De la Fuente, M. K., Landskron, G., Gonzalez, M. J., Quera, R., Dijkstra, G., et al. (2019). Short chain fatty acids (SCFAs)-mediated gut epithelial and immune regulation and its relevance for inflammatory bowel diseases. Front. Immunol. 10:277. doi: 10.3389/fimmu.2019.00277
Park, B. S., Song, D. H., Kim, H. M., Choi, B. S., Lee, H., and Lee, J. O. (2009). The structural basis of lipopolysaccharide recognition by the TLR4-MD-2 complex. Nature 458, 1191–1195. doi: 10.1038/nature07830
Pearl, D. S., Shah, K., Whittaker, M. A., Nitch-Smith, H., Brown, J. F., Shute, J. K., et al. (2013). Cytokine mucosal expression in ulcerative colitis, the relationship between cytokine release and disease activity. J. Crohns Colitis 7, 481–489. doi: 10.1016/j.crohns.2012.07.022
Puhka, M., Nordberg, M. E., Valkonen, S., Rannikko, A., Kallioniemi, O., Siljander, P., et al. (2017). KeepEX, a simple dilution protocol for improving extracellular vesicle yields from urine. Eur. J. Pharm. Sci. 98, 30–39. doi: 10.1016/j.ejps.2016.10.021
Quevrain, E., Maubert, M. A., Michon, C., Chain, F., Marquant, R., Tailhades, J., et al. (2016). Identification of an anti-inflammatory protein from Faecalibacterium prausnitzii, a commensal bacterium deficient in Crohn’s disease. Gut 65, 415–425. doi: 10.1136/gutjnl-2014-307649
Rabiei, N., Ahmadi Badi, S., Ettehad Marvasti, F., Nejad Sattari, T., Vaziri, F., and Siadat, S. D. (2019). Induction effects of Faecalibacterium prausnitzii and its extracellular vesicles on toll-like receptor signaling pathway gene expression and cytokine level in human intestinal epithelial cells. Cytokine 121:154718. doi: 10.1016/j.cyto.2019.05.005
Reunanen, J., Kainulainen, V., Huuskonen, L., Ottman, N., Belzer, C., Huhtinen, H., et al. (2015). Akkermansia muciniphila adheres to enterocytes and strengthens the integrity of the epithelial cell layer. Appl. Environ. Microbiol. 81, 3655–3662. doi: 10.1128/aem.04050-14
Rossi, O., Khan, M. T., Schwarzer, M., Hudcovic, T., Srutkova, D., Duncan, S. H., et al. (2015). Faecalibacterium prausnitzii strain HTF-F and its extracellular polymeric matrix attenuate clinical parameters in DSS-induced colitis. PLoS One 10:e0123013. doi: 10.1371/journal.pone.0123013
Saemann, M. D., Bohmig, G. A., Osterreicher, C. H., Burtscher, H., Parolini, O., Diakos, C., et al. (2000). Anti-inflammatory effects of sodium butyrate on human monocytes: potent inhibition of IL-12 and up-regulation of IL-10 production. FASEB J. 14, 2380–2382. doi: 10.1096/fj.00-0359fje
Schirmer, M., Smeekens, S. P., Vlamakis, H., Jaeger, M., Oosting, M., Franzosa, E. A., et al. (2016). Linking the human gut microbiome to inflammatory cytokine production capacity. Cell 167, 1125–1136.e8. doi: 10.1016/j.cell.2016.10.020
Schwechheimer, C., and Kuehn, M. J. (2015). Outer-membrane vesicles from Gram-negative bacteria: biogenesis and functions. Nat. Rev. Microbiol. 13, 605–619. doi: 10.1038/nrmicro3525
Shen, Y., Giardino Torchia, M. L., Lawson, G. W., Karp, C. L., Ashwell, J. D., and Mazmanian, S. K. (2012). Outer membrane vesicles of a human commensal mediate immune regulation and disease protection. Cell Host Microbe 12, 509–520. doi: 10.1016/j.chom.2012.08.004
Sokol, H., Pigneur, B., Watterlot, L., Lakhdari, O., Bermudez-Humaran, L. G., Gratadoux, J. J., et al. (2008). Faecalibacterium prausnitzii is an anti-inflammatory commensal bacterium identified by gut microbiota analysis of Crohn disease patients. Proc. Natl. Acad. Sci. U.S.A. 105, 16731–16736. doi: 10.1073/pnas.0804812105
Van De Walle, J., Hendrickx, A., Romier, B., Larondelle, Y., and Schneider, Y. J. (2010). Inflammatory parameters in Caco-2 cells: effect of stimuli nature, concentration, combination and cell differentiation. Toxicol. In Vitro 24, 1441–1449. doi: 10.1016/j.tiv.2010.04.002
Vatanen, T., Kostic, A. D., d’Hennezel, E., Siljander, H., Franzosa, E. A., Yassour, M., et al. (2016). Variation in microbiome LPS immunogenicity contributes to autoimmunity in humans. Cell 165, 842–853. doi: 10.1016/j.cell.2016.04.007
Vesterlund, S., Karp, M., Salminen, S., and Ouwehand, A. C. (2006). Staphylococcus aureus adheres to human intestinal mucus but can be displaced by certain lactic acid bacteria. Microbiology 152(Pt 6), 1819–1826. doi: 10.1099/mic.0.28522-0
Walker, A., Pfitzner, B., Harir, M., Schaubeck, M., Calasan, J., Heinzmann, S. S., et al. (2017). Sulfonolipids as novel metabolite markers of Alistipes and Odoribacter affected by high-fat diets. Sci. Rep. 7:11047. doi: 10.1038/s41598-017-10369-z
Wang, Y., Gao, X., Ghozlane, A., Hu, H., Li, X., Xiao, Y., et al. (2018). Characteristics of faecal microbiota in paediatric crohn’s disease and their dynamic changes during infliximab therapy. J. Crohns Colitis 12, 337–346. doi: 10.1093/ecco-jcc/jjx153
Weintraub, A., Zahringer, U., Wollenweber, H. W., Seydel, U., and Rietschel, E. T. (1989). Structural characterization of the lipid A component of Bacteroides fragilis strain NCTC 9343 lipopolysaccharide. Eur. J. Biochem. 183, 425–431. doi: 10.1111/j.1432-1033.1989.tb14945.x
Werner, H., Rintelen, G., and Kunstek-Santos, H. (1975). [A new butyric acid-producing Bacteroides species: B. splanchnicus n. sp. (author’s transl)]. Zentralbl. Bakteriol. Orig. A 231, 133–144.
Keywords: Odoribacter, LPS, OMV, host-microbe interactions, immunoregulation, gut microbiota
Citation: Hiippala K, Barreto G, Burrello C, Diaz-Basabe A, Suutarinen M, Kainulainen V, Bowers JR, Lemmer D, Engelthaler DM, Eklund KK, Facciotti F and Satokari R (2020) Novel Odoribacter splanchnicus Strain and Its Outer Membrane Vesicles Exert Immunoregulatory Effects in vitro. Front. Microbiol. 11:575455. doi: 10.3389/fmicb.2020.575455
Received: 25 June 2020; Accepted: 26 October 2020;
Published: 12 November 2020.
Edited by:
Hari S. Misra, Bhabha Atomic Research Centre (BARC), IndiaReviewed by:
Thomas Secher, Université de Tours, FranceTimothy James Wells, The University of Queensland, Australia
Copyright © 2020 Hiippala, Barreto, Burrello, Diaz-Basabe, Suutarinen, Kainulainen, Bowers, Lemmer, Engelthaler, Eklund, Facciotti and Satokari. This is an open-access article distributed under the terms of the Creative Commons Attribution License (CC BY). The use, distribution or reproduction in other forums is permitted, provided the original author(s) and the copyright owner(s) are credited and that the original publication in this journal is cited, in accordance with accepted academic practice. No use, distribution or reproduction is permitted which does not comply with these terms.
*Correspondence: Kaisa Hiippala, kaisa.hiippala@helsinki.fi