Introduction
High-resolution ultrasound is a novel non-invasive tool that is increasingly used to screen, diagnose, and facilitate the treatment of patients with suspected neurologic disorders. In particular, it has been used as part of a comprehensive evaluation of neuromuscular diseases. Such an approach requires the integration of clinical assessments, electrodiagnostic tests, neuroimaging, pathology, and laboratory investigations including targeted genetic testing. Muscle and nerve imaging complements the clinical and electrophysiology exam by accurately depicting the specific area or pattern of abnormalities, allowing direct visualization of spontaneous muscle movements, as well as monitoring for other associated pathologies.
In the early years of neuromuscular imaging (1985-2000), muscle computed tomography (CT) was developed as a tool to scan the whole body in search of decreased tissue density caused by fatty degeneration that would point to a pattern of involvement in a specific myopathy or neuropathy.Reference Hawley, Schellinger and O’Doherty 1 , Reference Marconi, Mahjneh and Pizzi 2 , Reference Swash, Brown and Thakkar 3 However, CT has two main drawbacks, including low-tissue resolution and the use of ionizing radiation that make it a less desirable option since more patient-friendly, high-resolution techniques including high-frequency neuromuscular ultrasound and magnetic resonance myography and neurography have become available.Reference Stoll, Wilder-Smith and Bendszus 4 , Reference Simon, Noto and Zaidman 5
MRI of muscle or nerve can accurately depict tissue size, texture, composition, organization, and anatomical context, both in superficial and deep body layers. It can either create a large overview of body regions to help search for a pattern of muscle involvement,Reference ten Dam, van der Kooi, Verhamme, Wattjes and de Visser 6 , Reference Leung 7 or can very focally depict specific tissue changes within a certain body region to look for atrophy, fatty degeneration, edema, inflammation, morphological or tissue texture changes, and continuity.Reference Leung 7 – Reference Chhabra, Madhuranthakam and Andreisek 9 Both visual evaluation and quantified measurement, for example, of fat fractions or nerve size, are possible. The main drawbacks of MRI seem to be the limited availability of the necessary dedicated protocols, software or coils at many centers, and the need for sedation in young children or claustrophobic patients.
Ultrasound is a feasible alternative to MRI for neuromuscular imaging of superficial structures; it is a portable and readily available technology that can be used in children and adults. Recent studies have shown that neuromuscular ultrasound can reliably screen for myopathies and neuropathies, can help provide a more certain diagnosis of amyotrophic lateral sclerosis (ALS) at the time of presentation, and can be used as a non-invasive biomarker for treatment trials in muscular dystrophy and other neuromuscular diseases.Reference Cartwright, Hobson-Webb and Boon 10 – Reference Zaidman, Wu and Kapur 15 This review will focus on the clinical applications of neuromuscular ultrasound. It offers added diagnostic values that adult and pediatric neurologists, clinical neurophysiologists, and other specialists can use for patient-friendly, point of care imaging of muscles and nerves.
Practical Uses of Muscle Ultrasound
As a Screening Tool for Neuromuscular Diseases
Ultrasound is a valid and reliable diagnostic imaging technique for the evaluation of nerves and muscles.Reference Cartwright, Demar, Griffin, Balakrishnan, Harris and Walker 16 A recent systematic review based on publications from 2000 to 2014 confirmed the utility and acceptability of ultrasound for the diagnosis of pediatric skeletal muscle disorders.Reference Rahmani, Mohseni-Bandpei, Vameghi, Salavati and Abdollahi 17 In comparison to electromyography (EMG), muscle ultrasound is better tolerated. Among 498 children with suspected neuromuscular diseases, Hellmann et alReference Hellmann, von Kleist-Retzow, Haupt, Herkenrath and Schauseil-Zipf 18 reported that 17% of EMG studies were suboptimal due to intolerance to pain, even though EMG was very sensitive in detecting neurogenic disorders. As well, EMG had more difficulty in detecting myopathies in young children; the sensitivity increased from 25% in infants to 91% in children above 5 years of age.Reference Hellmann, von Kleist-Retzow, Haupt, Herkenrath and Schauseil-Zipf 18 Alternatively, MRI can visualize deeper muscles and detect changes that are indicative of disease progression in neuromuscular diseases; however, as mentioned above, MRI has a number of potential limitations, especially in the pediatric population. Furthermore, Zaidman et alReference Zaidman, Seelig, Baker, Mackinnon and Pestronk 19 found that nerve ultrasound was more sensitive than MRI in detecting focal neuropathies or brachial plexopathy.
The potential diagnostic benefit of muscle ultrasound was highlighted in several pediatric studies. Following an initial pilot study of 33 children, Pillen et al prospectively studied 150 (90 males, age ranged from birth to 18 years) children referred consecutively for suspected neuromuscular disorders.Reference Pillen, Scholten, Zwarts and Verrips 20 , Reference Pillen, Verrips, van Alfen, Arts, Sie and Zwarts 21 The presenting symptoms were muscle weakness, fatigue, myalgia, hypotonia, and motor developmental delay. A 15-minute standard muscle ultrasound protocol (see Figure 1A) including measurement of muscle thickness (MT) and echo intensity (EI) in two proximal (i.e. biceps brachii, see Figure 2; and rectus femoris, see Figure 3) and two distal (i.e. flexor carpi radialis, see Figure 4; and tibialis anterior, see Figure 5) muscles were used in this study, and the results were compared with their published pediatric normative values;Reference Scholten, Pillen, Verrips and Zwarts 22 additional investigations included EMG, biochemical and genetic evaluations, plus muscle biopsies were performed as clinically indicated. In total, 65 (43%) patients were subsequently diagnosed with a neuromuscular disease; 54 (36%) did not show any evidence of a neurogenic or myopathic disorder; and 31 (21%) were excluded from further analysis due to inconclusive results.Reference Pillen, Verrips, van Alfen, Arts, Sie and Zwarts 21 According to Pillen et al,Reference Pillen, Verrips, van Alfen, Arts, Sie and Zwarts 21 the EI of all examined muscles were found to be significantly higher in patients with neuromuscular diseases than those without. Abnormal muscle ultrasound in their study was defined by an EI >3.5 standard deviation (SD) in one muscle, or >2.5 SD in two muscles, or >1.5 SD in three muscles; these ultrasound criteria were associated with a sensitivity of 71% (95% confidence interval [CI] 60%-82%), a specificity of 91% (CI 83%-99%), and a positive predictive value (PPV) of 91% (CI 82%-98%) in diagnosing pediatric neuromuscular diseases. Normal muscle ultrasound in turn was defined by an EI of less than 2.0 SD in all four muscles, plus <1.5 SD in three muscles, and <1.0 SD in two muscles; these parameters were associated with a sensitivity of 91% (CI 84%-98%), a specificity of 67% (CI 54%-80%), and a negative predictive value (NPV) of 86% (CI 76%-96%).Reference Pillen, Verrips, van Alfen, Arts, Sie and Zwarts 21
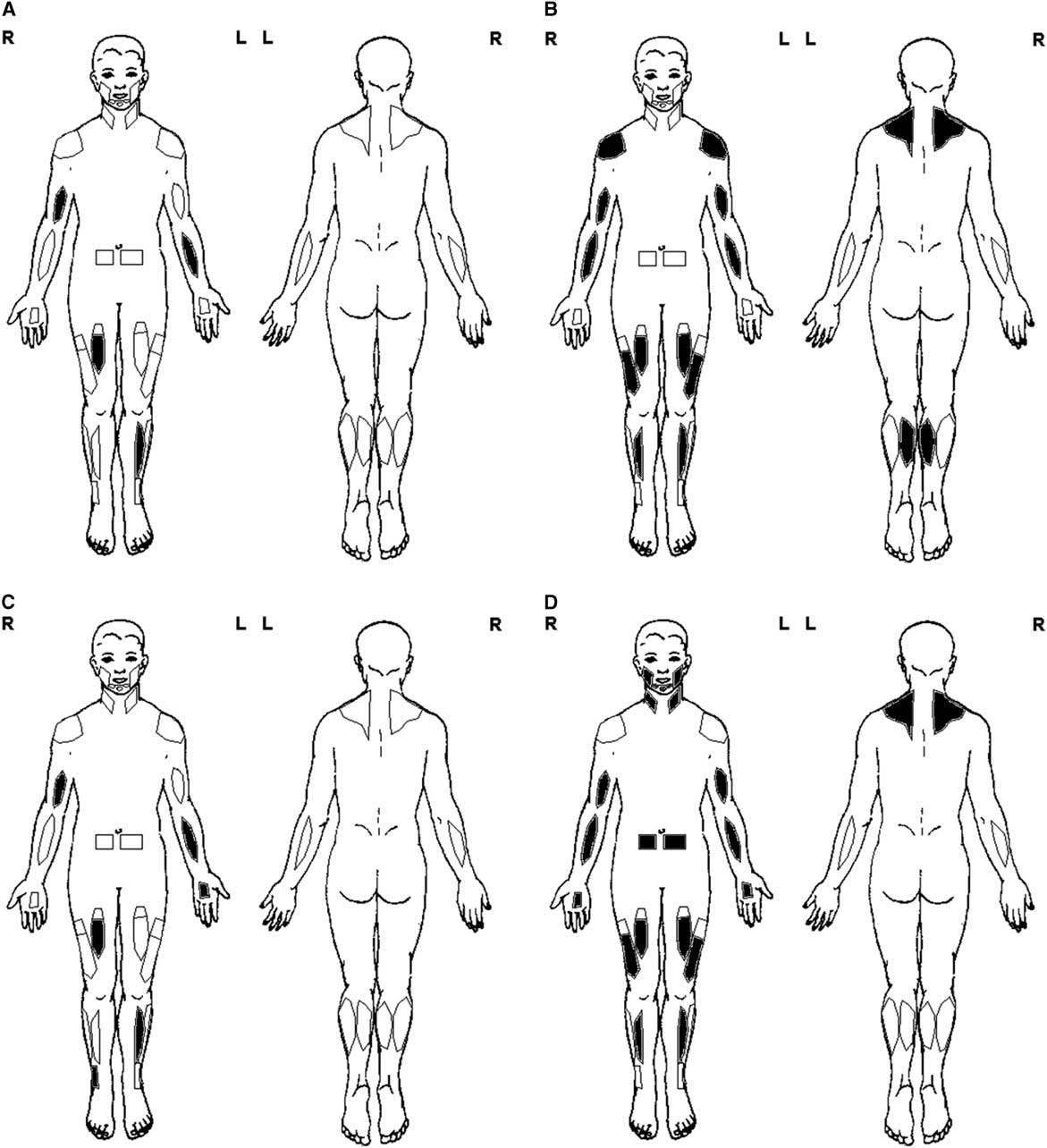
Figure 1 Basic muscle ultrasound protocol. (A) General screen—unilateral exam of the biceps brachii, flexor carpi radialis, rectus femoris, and tibialis anterior. (B) Myopathy screen—bilateral exam of the trapezius, deltoid, biceps brachii, flexor carpi radialis, rectus femoris, vastus lateralis, tibialis anterior, and medial gastrocnemius. (C) Polyneuropathy screen—general screen protocol (A) and additional exam of distal muscles such as the peroneus tertius and first dorsal interosseous. (D) Motor neuron disease screen—myopathy screen protocol (B) and additional exam of rectus abdominis, sternocleidomastoid, trapezius, masseter, first dorsal interosseous, and submental muscles, plus fasciculation screening of 30-second scan time per muscle.

Figure 2 Muscle ultrasound image of the biceps brachii, as recorded at 2/3 of the distance from the acromion to the antecubital crease.

Figure 3 Muscle ultrasound of the rectus femoris, as recorded at 1/2 of the distance between the anterior superior iliac spine and the upper pole of the patella.

Figure 4 Muscle ultrasound of the flexor carpi radialis, as recorded at 1/3 of the distance from the antecubital crease to the distal radius.
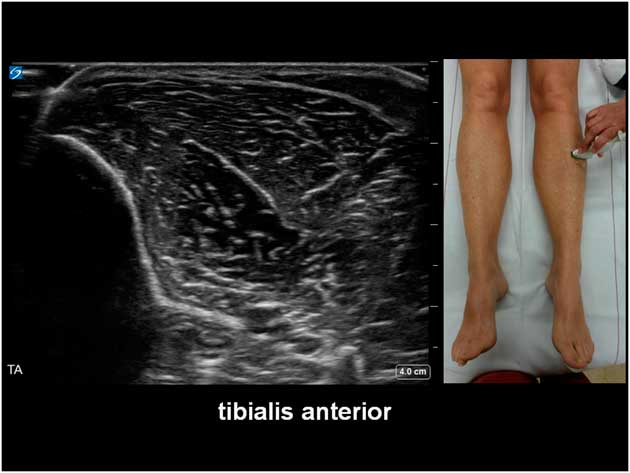
Figure 5 Muscle ultrasound of the tibialis anterior, as recorded at 1/3 of the distance from the inferior border of the patella to the lateral malleolus.
The sensitivity of muscle ultrasound in detecting neuromuscular diseases was lower among 43 patients who were less than 3 years of age, with a false-negative rate of 25%, but no false positives were found in this age group (PPV 100%); the false negatives were seven children with early stages of neuromuscular diseases or metabolic myopathies, including one case of congenital myopathy with fiber-type disproportion, two with mitochondrial cytopathies, one with Pompe disease, one with an unspecified myopathy, one with presymptomatic Duchenne muscular dystrophy (DMD), and one with early spinal muscular atrophy (SMA).Reference Pillen, Verrips, van Alfen, Arts, Sie and Zwarts 21 As well, two children were presumed to be false positives, with abnormal EI on ultrasound but no other evidence of neuromuscular diseases.Reference Pillen, Verrips, van Alfen, Arts, Sie and Zwarts 21 Furthermore, the authors reported in a companion study that muscle ultrasound was less sensitive (25%-46%) as a screening tool in young children with mitochondrial disorders, due to only subtle structural changes in the muscles.Reference Pillen, Morava and Van Keimpema 23
In addition, the distribution of ultrasound abnormalities as well as the pattern of muscle involvement can aid in the diagnosis of neuromuscular diseases. The common patterns of ultrasound abnormalities in specific neuromuscular disorders are summarized in Table 1. Although MT measurements showed more prominent atrophy of the lower extremities in neurogenic disorders, discrimination between neurogenic disorders and myopathies based on the degree of muscle atrophy alone had low diagnostic accuracy, with an area under the receiver operating characteristic curve of <0.72.Reference Pillen, Verrips, van Alfen, Arts, Sie and Zwarts 21 Instead, a combined cut-off based on EI (increased in legs by more than 1 SD than arms) and MT (atrophy greater in legs than arms) was better at detecting neurogenic disorders, with a sensitivity of 67% (CI 49%-85%), a specificity of 94% (CI 87%-100%), a PPV of 86% (CI 71%-100%), and a NPV of 84% (CI 74%-94%); further discrimination of myopathies from neurogenic and non-neuromuscular disorders was limited by disease heterogeneity in the study cohort.Reference Pillen, Verrips, van Alfen, Arts, Sie and Zwarts 21
Table 1 Common patterns of ultrasound abnormalities in neuromuscular disorders

Modified from Pillen et al.Reference Pillen, Arts and Zwarts 124
↑=slightly increased echo intensity or muscle thickness; ↑↑=moderately increased echo intensity; ↑↑↑=severely increased echo intensity; ↓=slight atrophy; ↓↓=moderate atrophy; ↓↓↓=severe atrophy; N=normal echo intensity or muscle thickness; US=ultrasound.
Similarly, others authors have used ultrasound parameters such as muscle inhomogeneity, echogenicity, and thickness in a quantitative or qualitative approach to help differentiate between myopathies and neuropathies among adults and children with suspected neuromuscular disorders.Reference Maurits, Bollen, Windhausen, De Jager and Van Der Hoeven 24 , Reference Maurits, Beenakker, van Schaik, Fock and Van Der Hoeven 25 , Reference Brandsma, Verbeek and Maurits 26 Interestingly, a recent study found that boys with DMD may not show significant muscle atrophy over the course of their disease,Reference Jansen, van Alfen, Nijhuis van der Sanden, van Dijk, Pillen and de Groot 11 and muscle pseudo-hypertrophy can occur in both neurogenic and myopathic disorders.Reference Gutmann 27 , Reference Reimers, Schlotter, Eicke and Witt 28 Both findings might explain why MT is not a very good discriminator for detecting and differentiating neuromuscular diseases.
On the other hand, selective pattern of muscle involvement can provide important diagnostic clues for the differential diagnosis of various myopathies. A systematic approach involving bilateral ultrasound exam of muscles as described in the standard ultrasound protocol, plus other proximal and distal muscles such as the trapezius, deltoid, vastus lateralis, and medial gastrocnemius are recommended as part of a myopathy screen (see Figure 1B). The presence of a peripheral rim of abnormal signal in the vastus lateralis and a central area of abnormal signal within the rectus femoris can be found in collagen VI myopathies, and early selective involvement of the rectus femoris and vastus lateralis is typically seen in RYR1-related central core myopathies (Figure 6).Reference Zaidman and van Alfen 14 , Reference Bönnemann, Brockmann and Hanefeld 29 – Reference Wattjes, Kley and Fischer 31 As well, muscle ultrasound can detect a greater degree of muscle changes in patients with myotonic dystrophies and other non-dystrophic myotonic disorders, at times better than muscle MRI.Reference Trip, Pillen, Faber, van Engelen, Zwarts and Drost 32 , Reference Tieleman, Vinke, van Alfen, van Dijk, Pillen and van Engelen 33

Figure 6 Transverse axis ultrasound of the quadriceps showing increased echo intensity of the rectus femoris and vastus intermedius in an adult with RYR1-related central core myopathy.
Improving the Diagnosis of Motor Neuron Diseases
Ultrasound enhances the ability to detect fasciculations in 10% to 30% of muscles that may be subclinically involved or negative on EMG, thus increasing the diagnostic certainty for patients with motor neuron diseases and other neuromuscular disorders.Reference Reimers, Ziemann, Scheel, Rieckmann, Kunkel and Kurth 34 – Reference Johansson, Ellegaard, Tankisi, Fuglsang-Frederiksen and Qerama 37 In addition to bilateral examination of the muscles as described in the standard muscle ultrasound protocol, screening of lower motor neuron disease usually involves additional scanning of the rectus abdominis, sternocleidomastoid, trapezius, masseter, first dorsal interosseous, and submental muscles, with fasciculation screening of up to 60-second scan time per muscle (see Figure 1D).
Arts et alReference Arts, van Rooij and Overeem 35 used ultrasound as part of a cross-sectional study to examine ten muscles in each of their 48 patients with ALS and 27 patients with ALS mimics; their study found that the increase in EI was more striking than the reduction of MT in ALS. Fasciculations were identified in all but one of the 25 patients screened using a 10-second scan time, with a mean of 6.5 (range 0-10) muscles showing fasciculations; the authors concluded that ultrasound could detect muscle changes during the early phase of ALS.Reference Arts, van Rooij and Overeem 35 In another prospective study of 59 adults with suspected ALS, muscle ultrasound was able to differentiate patients with ALS (n=27) from others (n=32) with ALS mimics (see example in Figures 7A–7B); the corresponding sensitivity and specificity was 96% and 84%.Reference Arts, Overeem and Pillen 38 In two recent studies, fasciculations were detected in 58%Reference Johansson, Ellegaard, Tankisi, Fuglsang-Frederiksen and Qerama 37 to 68%Reference Noto, Shibuya and Shahrizaila 39 of patients with ALS based on an ultrasound scan time of 10 or 60 seconds per muscle, respectively; higher sensitivities were reported when the results of ultrasound were combined with EMG. In the cranial region of ALS patients, the muscle ultrasound detection rate for fasciculations was found to be similar to that of EMG, although frequent fasciculations were again more easily detected with ultrasound.Reference O’Gorman, Weikamp and Baria 40

Figure 7 Example of muscle ultrasound showing different pattern of involvement in amyotophic lateral sclerosis (ALS) versus ALS mimics. (a) ALS, with mild to moderately increased echo intensity, diffuse fasciculations, and atrophy of paretic muscles; (b) Inclusion body myositis, with severely increased echo intensity, either none or some fasciculations, and pronounced atrophy.
As well, among 81 adults consecutively referred for suspected ALS, Misawa et al reported that the combination of ultrasound and EMG increased the diagnostic category of probable or definite ALS from 48% (based on the revised El Escorial criteria) to 79% (based on the Awaji criteria), in part due to the enhanced ability of muscle ultrasound to detect fasciculations.Reference Misawa, Noto and Shibuya 36 In ALS patients who had fasciculations, a score based on the ultrasound results of nine muscles was able to discriminate between ALS and ALS mimics with 92% sensitivity and 100% specificity.Reference Tsuji, Noto, Shiga, Teramukai, Nakagawa and Mizuno 41 Other publications also confirmed the utility of muscle ultrasound as part of a comprehensive assessment of patients with suspected ALS.Reference Johansson, Ellegaard, Tankisi, Fuglsang-Frederiksen and Qerama 37 , Reference Grimm, Prell and Décard 42 Nerve ultrasound can also help differentiate between patients with ALS and multifocal motor neuropathy (MMN), with a sensitivity ranging from 87% to 100%, and a specificity of 92% to 94%.Reference Grimm, Décard, Athanasopoulou, Schweikert, Sinnreich and Axer 43 , Reference Loewenbrück, Liesenberg and Dittrich 44 Furthermore, ultrasound can potentially be used to monitor for disease progression in adults with ALS,Reference Shen and Cartwright 13 although others have found conflicting results.Reference Arts, Overeem, Pillen, Schelhaas and Zwarts 45
Improving the Diagnosis of Metabolic Myopathies
Although earlier studies suggest that muscle ultrasound has lower sensitivity in detecting metabolic myopathies compared with structural myopathies and muscular dystrophies in children, more recent studies show that muscle ultrasound can still be clinically useful, for example, in the diagnosis of Pompe disease due to acid-maltase deficiency, or by showing muscle changes in other glycogen storage diseases.Reference Verbeek, Sentner and Smit 46 In one study, muscle ultrasound showed focal abnormalities affecting the deeper layers of biceps brachii, with relative sparing of the triceps brachii; more severe involvement of the vastus intermedius in comparison with the rectus femoris was also reported in adults with Pompe disease.Reference Zaidman, Malkus, Siener, Florence, Pestronk and Al-Lozi 47 Vill et alReference Vill, Schessl and Teusch 48 did not find a selective pattern of muscle vulnerability in patients with Pompe disease; instead, they reported that bone echogenicity was less attenuated even in advance stages of the disease, and muscle ultrasound was used as a screening tool for adults (but not in infants) with late-onset disease. More recently, Hwang et alReference Hwang, Hsu, Lee, Wang, Chiou and Niu 49 advocated for the use of semi-quantitative muscle ultrasound as a diagnostic tool to help discriminate between neonates with low acid alpha-glucosidase activity related to infantile or late-onset Pompe disease; the sensitivity of muscle ultrasound was reported as 100% (CI 69.2%-100%) and the specificity was 84% (CI 63.9%-95.5%).
Guiding Needle Placement
Ultrasound has been used increasingly to guide botulinum toxin injections and other bedside treatment procedures.Reference Davidson and Jayaraman 50 , Reference Walter and Dressler 51 For the treatment of spasticity and dystonia with botulinum toxin, ultrasound guidance has been shown to improve clinical outcomes,Reference Santamato, Micello and Panza 52 , Reference Grigoriu, Dinomais, Rémy-Néris and Brochard 53 and ultrasound-guided needle muscle biopsy offers a viable alternative to open biopsy for the diagnosis of neuromuscular diseases, with comparable tissue samples results.Reference O’Sullivan, Gorman, Hardiman, Farrell and Logan 54 Ultrasound can help determine the optimal muscle biopsy site; the use of tissue Doppler evaluation before and after the biopsy can also help minimize potential complications by avoiding vessels and nerves.Reference O’Sullivan, Gorman, Hardiman, Farrell and Logan 54 Furthermore, ultrasound can be used to guide needle EMG to distinguish muscles in close proximity,Reference Wininger, Buckalew, Kaufmanan and Munin 55 and to enhance patient safety when needling the diaphragm,Reference Boon and O’Gorman 56 the periscapular muscles,Reference Krzesniak-Swinarska, Caress and Cartwright 57 or other muscles in patients on anticoagulants.Reference Boon 58 In a cadaveric study, ultrasound greatly improved needle EMG placement accuracy in most muscles.Reference Yun, Chung and Kim 59 As well, a recent review showed that ultrasound guidance increased the success rates of lumbar puncture and reduced the procedural time in patients with poorly palpable landmarks related to obesity, prior spinal surgery, and other difficult clinical circumstances.Reference Soni, Franco-Sadud and Schnobrich 60 Further studies are needed to determine whether ultrasound may facilitate the delivery of novel disease-modifying treatment such as intrathecal injections for patients with SMA.
Assessing Muscles that Cannot be Easily Accessed with EMG
Dysphagia is a common symptom in patients with neuromuscular diseases. A recent systematic review of dysphagia in children focused primarily on boys with DMD; there was a lack of widely accepted screening or evaluation protocol for other pediatric neuromuscular disorders.Reference Audag, Goubau, Toussaint and Reychler 61 Boys with DMD were found to have progressive involvement of the oropharyngeal muscles, necessitating the modification of feeding strategies to minimize dysphagia.Reference van den Engel-Hoek, Erasmus and Hendriks 62 As transporting and swallowing requires the use of more than 25 pairs of oral and masticatory muscles that are difficult to be assessed by EMG, van den Engel-Hoek et alReference van den Engel-Hoek, Harding, van Gerven and Cockerill 63 proposed to use muscle ultrasound as a standard diagnostic tool for the assessment of dysphagia (Figures 8A–8B), based on their extensive experience with ultrasound in the neuromuscular population.

Figure 8 Dysphagia evaluation using Motion mode (M-mode) ultrasound of the tongue while swallowing 5 ml of water. (a) Healthy control showing clearly defined phases of tongue movement; (b) Young adult male with Duchenne muscular dystrophy showing minimal tongue movement due to severe weakness.
As well, ultrasound can be used as a non-invasive diagnostic test to determine the functional status of facial muscles, and to help identify ideal candidates for facial nerve reconstruction surgery even after an extended period of denervation.Reference van Alfen, Gilhuis, Keijzers, Pillen and van Dijk 64 , Reference Volk, Pohlmann, Sauer, Finkensieper and Guntinas-Lichius 65
Patients in the intensive care unit (ICU) are at risk of developing critical illness neuromuscular disorders. Traditionally, the clinical history, physical exam, and electrophysiological evaluations such as EMG and nerve conduction study are essential for the diagnosis of critical illness neuromyopathy; however, these assessments may be limited by a lack of patient cooperation due to sedation or encephalopathy, electrical interference, treatment with anticoagulants, or other confounding factors in the ICU. Therefore, Cartwright et alReference Cartwright, Kwayisi and Griffin 66 proposed that serial quantitative ultrasound can be used to study ICU-acquired weakness and muscle atrophy, without requiring patient cooperation. However, more recent studies suggest that ultrasound has difficulty detecting early changes (less than 2 weeks) in muscles of patients with ICU-acquired weakness, and the reliability of muscle ultrasound in this disorder is not fully established.Reference Bunnell, Ney, Gellhorn and Hough 67 – Reference Witteveen, Sommers and Wieske 68 In a recent review, Ong et alReference Ong, Lee, Leow and Puthucheary 69 summarized the limitations of ultrasound, including a substantial intra-rater variability in quadriceps MT that resulted in its low reliability and accuracy as a marker for critical illness neuromyopathy in the pediatric population. The diagnostic utility of ultrasound in this clinical context remains undefined.
In contrast to evaluating ICU-acquired weakness, ultrasound is an ideal non-invasive bedside procedure for the detection of early neuromuscular diaphragmatic dysfunction of any cause, with a sensitivity of 93% and a specificity of 100%.Reference Boon, Sekiguchi and Harper 70 , Reference Unbrello and Formenti 71
Biomarker for Treatment Trials
A longitudinal observational study of 18 boys with DMD showed high correlations of increasing muscle echogenicity with disease progression and functional status.Reference Jansen, van Alfen, Nijhuis van der Sanden, van Dijk, Pillen and de Groot 11 As well, among 5 young boys (age 0.5-2.8 years) with DMD, there was evidence of disease progression with increased muscle EI over 2.5 years, despite functional improvements during early childhood;Reference Zaidman, Malkus and Connolly 72 quantitative muscle ultrasound by backscatter or grayscale analysis from superficial regions of muscles provided comparable measures of disease pathology in this population.Reference Shklyar, Geisbush and Mijialovic 73 More recently, Zaidman et alReference Zaidman, Wu and Kapur 15 examined 36 ambulatory boys (age 2-14 years) with DMD for up to 2 years; the authors found that ultrasound was more sensitive in detecting clinical deterioration in DMD than motor functional assessments, including the 6-minute walk test or the supine-to-stand test. Boys with DMD showed significant increases in muscle EI due to progressive fibro-fatty tissue replacement (Figure 9); the changes were significant as early as 6 months from baseline for boys less than 7 years old, and at 12 months for boys 7 years or older when compared with age- and gender-matched healthy controls.Reference Zaidman, Wu and Kapur 15 Therefore, muscle ultrasound may serve as a potential biomarker for disease progression as well as a non-invasive outcome measure in multicenter drug trial for DMD, without requiring patient cooperation.Reference Rutkove, Kapur and Zaidman 74

Figure 9 Abnormal transverse axis quadriceps muscle ultrasound in a child with Duchenne muscular dystrophy; diffuse fibro-fatty tissue replacement in this disorder is shown with increased echogenicity, leading to a “ground glass” appearance.
Similarly, Ng et alReference Ng, Connolly and Zaidman 12 found evidence of progressive quadriceps atrophy and increased muscle EI in three infants with SMA type 1 at 2-4 months after initial assessments (Figure 10). The rapid decline in ultrasound measures was consistent with the severe phenotype of early onset SMA; ultrasound could potentially be used as a biomarker for disease progression and to determine responsiveness to new emerging treatment for this disease.Reference Ng, Connolly and Zaidman 12

Figure 10 Abnormal transverse quadriceps muscle ultrasound in a child with spinal muscular atrophy. The image shows increased subcutaneous fatty tissue, and a “moth-eaten” appearance representing areas of denervated atrophic fibers versus reinnervated, hypertrophic muscle fibers (the “moth holes”).
Practical Uses of Nerve Ultrasound
High-resolution ultrasound has been helpful in the evaluation of genetic and acquired neuropathies, as recently reviewed by a number of leading experts.Reference Shen and Cartwright 13 , Reference Boon and O’Gorman 56 , Reference Hobson-Webb and Padua 75 Ultrasound offers superior spatial resolution and ability to detect subtle structural abnormalities in peripheral nerves through the availability of high-frequency (12-18 MHz) transducers, advances in image processing, and sensitive Doppler technology.Reference Gallardo, Noto and Simon 76
Common clinical applications of nerve ultrasound include screening for entrapment, diagnosing genetic and acquired neuropathies, complementing EMG in the assessment of nerve trauma, surveying for tumor progression, and providing precise landmark for nerve injections. In experienced hands, ultrasound is more sensitive than MRI (93% vs. 67%) and has a comparable specificity (86%) for detecting peripheral nerve pathology.Reference Zaidman, Seelig, Baker, Mackinnon and Pestronk 19 As well, ultrasound examination was able to identify degenerative or post-traumatic changes, soft-tissue masses, and other distinct extraneural findings in nearly one-quarter of patients without peripheral nerve abnormalities; many of these patients benefited from the additional evaluation of these extraneural findings.Reference Bignotti, Zaottini, Airaldi, Martinoli and Tagliafico 77
Screening for Nerve Entrapment
Peripheral nerve injury leads to a number of anatomical changes, including altered dimension, echogenicity, and vascularity. In nerve entrapment syndromes, there may be focal areas of nerve enlargement just proximal to the site of the compression, with associated loss of the internal fascicular architecture, and reduction in nerve echogenicity.Reference Cartwright and Walker 78 Ultrasound has been helpful in confirming the precise anatomic localization of the injury, assessing for nerve continuity, as well as identifying other structural changes in a number of neuromuscular conditions.
Carpal Tunnel Syndrome (CTS)
Median mononeuropathy across the wrist is the most common type of nerve entrapment, and it has been studied extensively with the use of ultrasound (Figures 11A–11B).Reference Chen, Williams, Zak and Fredericson 79 A systemic review confirmed that the accuracy of ultrasound is comparable to EMG and nerve conduction studies for the diagnosis of CTS; based on a median nerve cross-sectional area (CSA) cut-off value of 8.5 mm2 to 10 mm2, Cartwright et alReference Cartwright, Hobson-Webb and Boon 10 concluded that ultrasound had an overall sensitivity of 65% to 97%, a specificity of 72.7% to 98%, and a PPV of 79% to 97% for the diagnosis of CTS. A greater than 40% increase in nerve size from the forearm to carpal tunnel was also found to be suspect for entrapment in patients with CTS,Reference Hobson-Webb, Massey, Juel and Sanders 80 but later studies have emphasized that the CSA itself remains the most robust measure to confirm the disorder.Reference Ažman, Hrabač and Demarin 81 Scanning the whole carpal tunnel instead of just the proximal inlet increased the sensitivity by 15% to 20%.Reference Paliwal, Therimadasamy, Chan and Wilder-Smith 82 , Reference Csillik, Bereczki, Bora and Arányi 83 Additionally, as ultrasound can detect other anatomic abnormality such as a ganglion cyst or accessory muscle, the authors concluded that ultrasound provides additional value to electrodiagnostic studies when assessing patients with CTS.Reference Cartwright, Hobson-Webb and Boon 10 The use of tissue Doppler plus standard ultrasound parameters may help confirm the diagnosis plus identify optimal treatment and improve surgical outcomes for CTS.Reference Chen, Williams, Zak and Fredericson 79 , Reference Ažman, Hrabač and Demarin 81
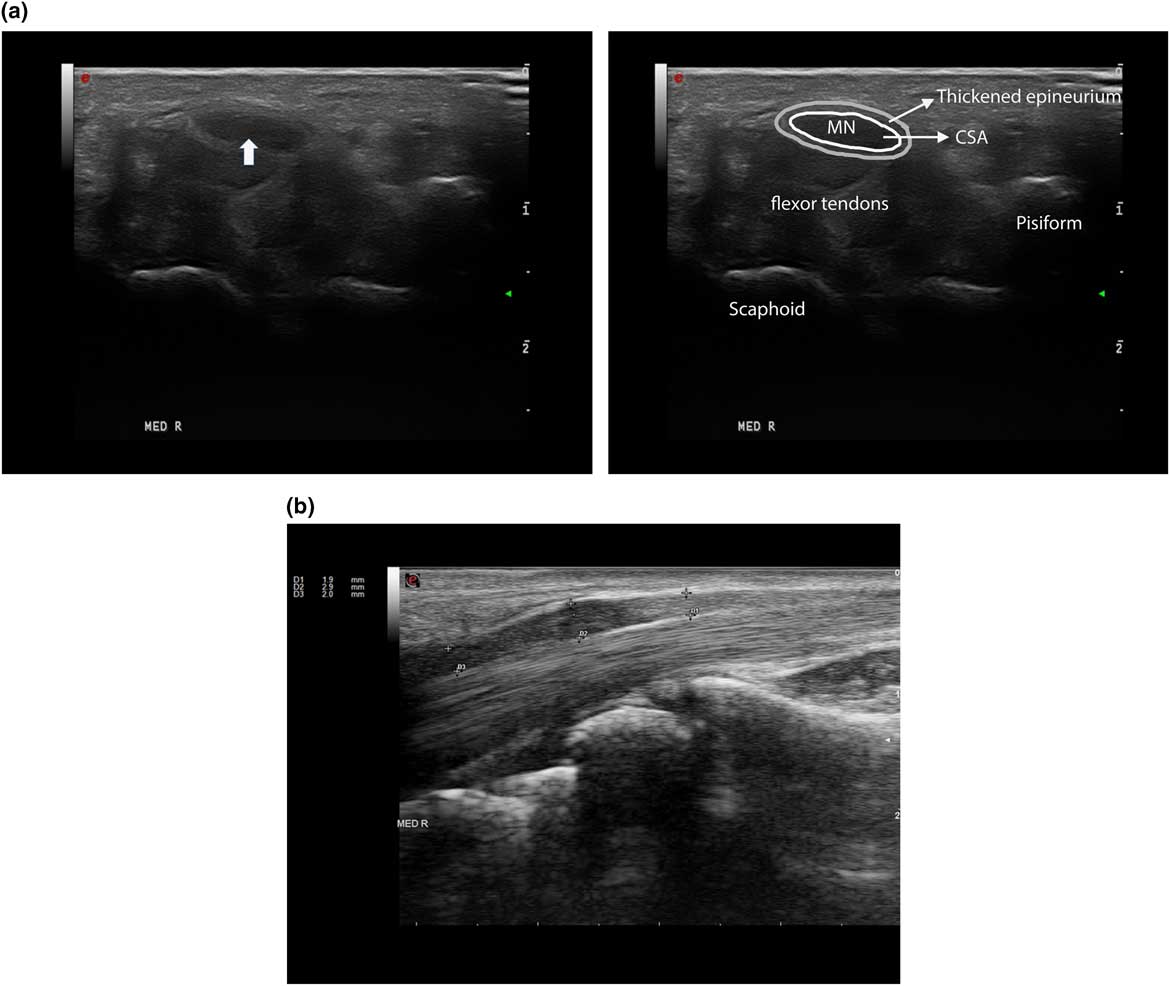
Figure 11 An example of a patient with Carpel tunnel syndrome. (a) Right median nerve in short axial view, with a cross-sectional area (CSA) of 17 mm2 (see arrow), normal<11 mm2. (b) Same nerve in longitudinal view showing focal nerve enlargement (marked by arrows) just proximal to notching caused by compression of the carpal ligament.
Other Entrapment Syndromes
Other entrapment syndromes including ulnar neuropathy at the elbow or wrist, and fibular neuropathy at the knee (Figure 12) can be evaluated according to specific nerve ultrasound study protocol.Reference Cartwright and Walker 78 As well, ultrasound has been useful for the diagnosis of meralgia parestheticaReference Suh, Kim, Park and Park 84 and neurogenic thoracic outlet syndrome.Reference Arányi, Csillik, Böhm and Schelle 85
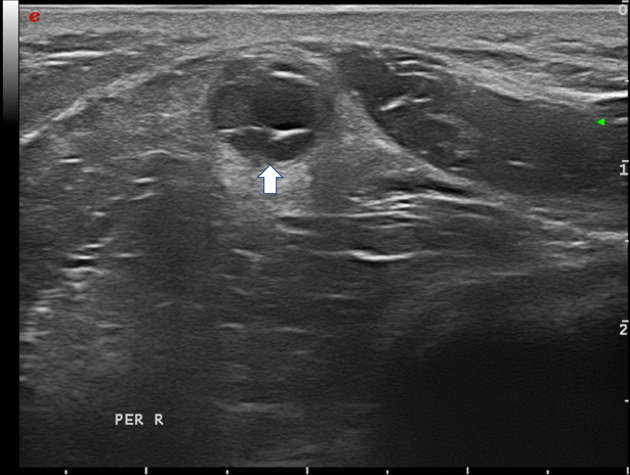
Figure 12 Fibular nerve with intraneural ganglion cyst (arrow); the hypoechoic cystic areas can be seen on the right, while the displaced fascicles are on the left side in the nerve.
Neuropathy in Hereditary Diseases
A recent cross-sectional study showed that nerve ultrasound is a useful screening tool for CTS in children with mucopolysaccharidosis type 1, 2 and 6, with higher (92%) sensitivity than EMG (77%) or clinical exam (26%) alone.Reference Bäumer, Bühring, Schelle, Münchau and Muschol 86 One year post carpal tunnel release surgery, there was improvement in the median distal motor latencies and compound motor action potential (CMAP) amplitudes; however, the median nerve CSA at the wrist and the wrist to forearm ratio remained unchanged.Reference Bäumer, Bühring, Schelle, Münchau and Muschol 86 As well, ultrasound was able to detect multifocal nerve enlargement in two siblings with neuropathies secondary to mucolipidosis type 3; electrophysiological studies were normal in both patients apart from distal median neuropathies.Reference Mulroy, Chancellor and Pelosi 87
Beyond the diagnosis of entrapment syndromes in lysosomal storage diseases, ultrasound has been used to assist with the diagnosis of hereditary neuropathies. Among the genetic neuropathies, Charcot-Marie-Tooth type 1A (CMT1A) is one of the most prevalent subtypes. It is an autosomal dominant inherited demyelinating motor and sensory polyneuropathy caused by duplication of the PMP22 gene. In patients with genetically confirmed CMT1A, ultrasound revealed uniformed nerves enlargement, with significantly increased CSA of the nerve roots, brachial plexus, and peripheral nerves, compared with those in healthy subjects and patients with CMT type 2.Reference Gallardo, Noto and Simon 76 , Reference Shahrizaila, Noto and Simon 88 Ultrasound may be particularly helpful in CMT type 1 patients with hypertrophic nerves and absent CMAP response due to distal muscle atrophy, or an inability to tolerate nerve conduction study due to the need for high stimulation intensity.Reference Gallardo, Noto and Simon 76 , Reference Shahrizaila, Noto and Simon 88 An increase in nerve CSA is also seen disproportionately in children with CMT1AReference Yiu, Brockley and Lee 89 and other demyelinating CMT subtypes.Reference Noto, Shiga and Tsuji 90 , Reference Luigetti, Sabatelli, Bellone, Fabrizi, Padua and Granata 91
In addition, hereditary neuropathy with liability to pressure palsy (HNPP) due to a deletion of the PMP22 gene is associated with focal nerve enlargement at common entrapment sites,Reference Padua, Coraci and Lucchetta 92 whereas non-inflammatory axonal neuropathies generally show normal nerve sizes.Reference Zaidman, Al-Lozi and Pestronk 93 , Reference Goedee, Brekelmans, van Asseldonk, Beekman, Mess and Visser 94 Loewenbrück et al further proposed the use of diagnostic ultrasound models to discriminate between demyelinating and axonal forms of CMT; they reported high sensitivity (84%-100%) and specificity (86%-100%) with ultrasound, especially for the axonal CMT subtypes.Reference Loewenbrück, Dittrich and Böhm 95 , Reference Loewenbrück, Dittrich and Böhm 96 Therefore, a combination of clinical exam, electrodiagnostic study, and nerve ultrasound may provide important clues for targeted gene testing.
Similarly, nerve ultrasound has been used to elucidate peripheral nerve involvement in Friedreich ataxia, autosomal dominant spinocerebellar ataxia, plus other cerebellar ataxia with neuropathy syndromes.Reference Mulroy, Pelosi and Leadbetter 97 – Reference Pelosi, Leadbetter, Mulroy, Chancellor, Mossman and Roxburgh 99
Diagnosis of Other Neuropathies
As summarized below, nerve ultrasound can aid in confirming the diagnosis of inflammatory neuropathies, nerve tumors, and traumatic nerve injuries; it can also be used in the evaluation of small fiber polyneuropathy.Reference Ebadi, Siddiqui, Ebadi, Ngo, Breiner and Bril 100 A polyneuropathy screen generally includes an examination of standard ultrasound muscles, with the addition of distal muscles such as the peroneus tertius, first dorsal interosseous, and other clinically affected muscles (see Figure 1C), as well as ultrasound scanning of common peripheral (median, ulnar, fibular, and tibial) nerves, plus proximal brachial plexus and nerve roots as clinically indicated.
Acquired Inflammatory Neuropathies
Acute (AIDP) or chronic (CIDP) inflammatory demyelinating polyneuropathy is associated with patchy enlargement of the peripheral nerves and nerve roots that can be visualized by ultrasound.Reference Gallardo, Noto and Simon 76 , Reference Décard, Fladt, Axer, Fischer and Grimm 101 , Reference Di Pasquale, Morino, Loreti, Bucci, Vanacore and Antonini 102 In one study of AIDP, multifocal nerve enlargements with increased CSA were seen within 3 weeks after the onset of symptoms, with sonographic improvement after treatment 3 months later.Reference Razali, Arumugam, Yuki, Rozalli, Goh and Shahrizaila 103 Another recent study provided class II evidence that a compact nerve ultrasound protocol with scanning of bilateral median nerves and brachial plexus has high positive and NPV (100% and 98%, respectively) for discriminating CIDP, Lewis-Sumner syndrome, and MMN from other clinical mimics.Reference Goedee, van der Pol and van Asseldonk 104 Thus nerve ultrasound may be used in conjunction with the clinical exam and electrophysiology to distinguish between different types of acquired demyelinating neuropathies and to optimize treatment plans.
In neuralgic amyotrophy, nerve inflammation with enlarged CSA can be seen involving the brachial plexus as well as peripheral nerves in the distal upper arm.Reference Lieba-Samal, Jengojan, Kasprian, Wöber and Bodner 105 – Reference ArÁnyi, Csillik and DéVay 108 Focal nerve enlargement can also at times be demonstrated by ultrasound in other axonal inflammatory disorders such as sarcoid neuropathyReference Kerasnoudis, Woitalla, Gold, Pitarokoili and Yoon 109 and neuropathy associated with vasculitis syndromes.Reference Goedee, van der Pol and van 110
Neurofibromatosis and Neurolymphomatosis
Ultrasound has been used to detect early or subclinical peripheral nerve involvement in neurofibromatosis type 1 (NF1) and 2 (NF2). Affected nerves show focal areas of gross hypoechoic enlargement of the various fascicles. Isolated focal enlargement constitute a localized neurofibroma, while multiple serpentine-like affected fascicles are seen in a plexiform neurofibroma. The abnormalities often extend over long segments of the main limb nerves and can also occur in skin nerve branches (Figure 13).Reference Telleman, Stellingwerff, Brekelmans and Visser 111 – Reference Winter, Rattay and Axer 113
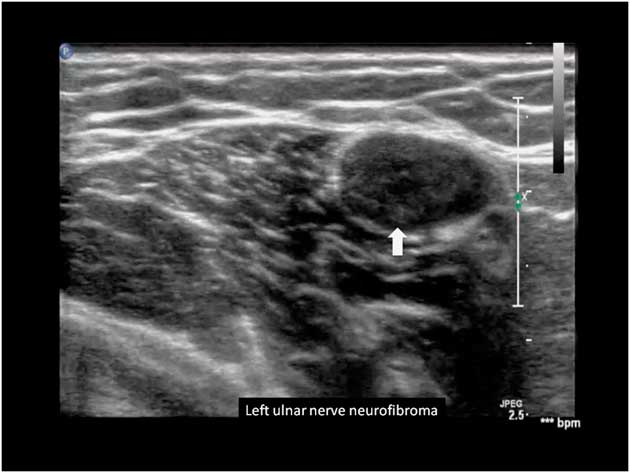
Figure 13 Left ulnar nerve with neurofibroma, marked by the arrow.
As well, in a recent case report of three adults with lymphoma and clinical evidence of focal neuropathy, nerve ultrasound was helpful in identifying lymphomatous peripheral nerve infiltration or neurolymphomatosis that was subsequently confirmed by biopsy.Reference Vijayan, Chan, Therimadasamy and Wilder-Smith 114 Therefore, ultrasound may be beneficial for peripheral nerve tumor surveillance in patients with neurofibromatosis and possibly neurolymphomatosis.Reference Briani, Visentin and Cavallaro 115
Traumatic Nerve Injuries
Ultrasound has also been used to assess patients with focal nerve injuries, including infants with obstetrical brachial plexopathy and adults with acquired peripheral nerve or brachial plexus trauma.Reference Gruber, Glodny and Galiano 116 – Reference Simon, Spinner, Kline and Kliot 117 Pillen et alReference Pillen, Semmekrot, Meulstee, Verrips and Van Alfen 118 recently showed that the cervical roots, brachial plexus, and proximal nerves can be visualized by ultrasound as early as the neonatal period, and reference data are available from their standardized nerve ultrasound protocol. As well, Smith et alReference Smith, Miller and Carroll 119 reported on the utility of ultrasound for patients with combat-related peripheral nerve injuries, in addition to standard clinical, EMG, and MRI exams. In their study, ultrasound was superior to electrodiagnostic test in the ability to clearly demonstrate the specific site of focal nerve involvement among four adults post gunshot or blasts injuries with non-excitable nerve responses; the location was later confirmed by surgical inspection.Reference Smith, Miller and Carroll 119
Injections for Entrapment Neuropathies
Ultrasound provides clear anatomic landmarks for steroids injections as part of the treatment for entrapment neuropathies;Reference Strakowski 120 in addition, it can be used to determine the clinical response to treatment. Lee et al found a significant reduction in the median nerve CSA starting 1-week post injection for CTS; changes in ultrasound parameters correlated with patient-reported improvement in pain scores.Reference Lee and Choi 121
Diagnostic Values of Ultrasound in Neuromuscular Disorders
A summary of the diagnostic values for ultrasound in specific myopathies, neuropathies, and motor neuron disorders is provided in Table 2. In experienced hands, ultrasound is a sensitive and specific tool for the detection of many neuromuscular disorders, and it can also be used as a screening tool among children with suspected peripheral nervous system diseases. It is not well suited as a general screening test for metabolic myopathies, and the utility of ultrasound may be reduced in young children under 3 years of age.Reference Pillen, Morava and Van Keimpema 23 , Reference Brockmann, Becker, Schreiber, Neubert, Brunner and Bönnemann 30 Alternative methods using texture analysis and acoustic structural quantification of sonographic images may further increase the diagnostic utility of ultrasound for many patients with neuromuscular diseases.Reference Molinari, Caresio, Acharya, Mookiah and Minetto 122 , Reference Weng, Tsui and Lin 123
Table 2 Examples of diagnostic values of ultrasound in specific neuromuscular disorders
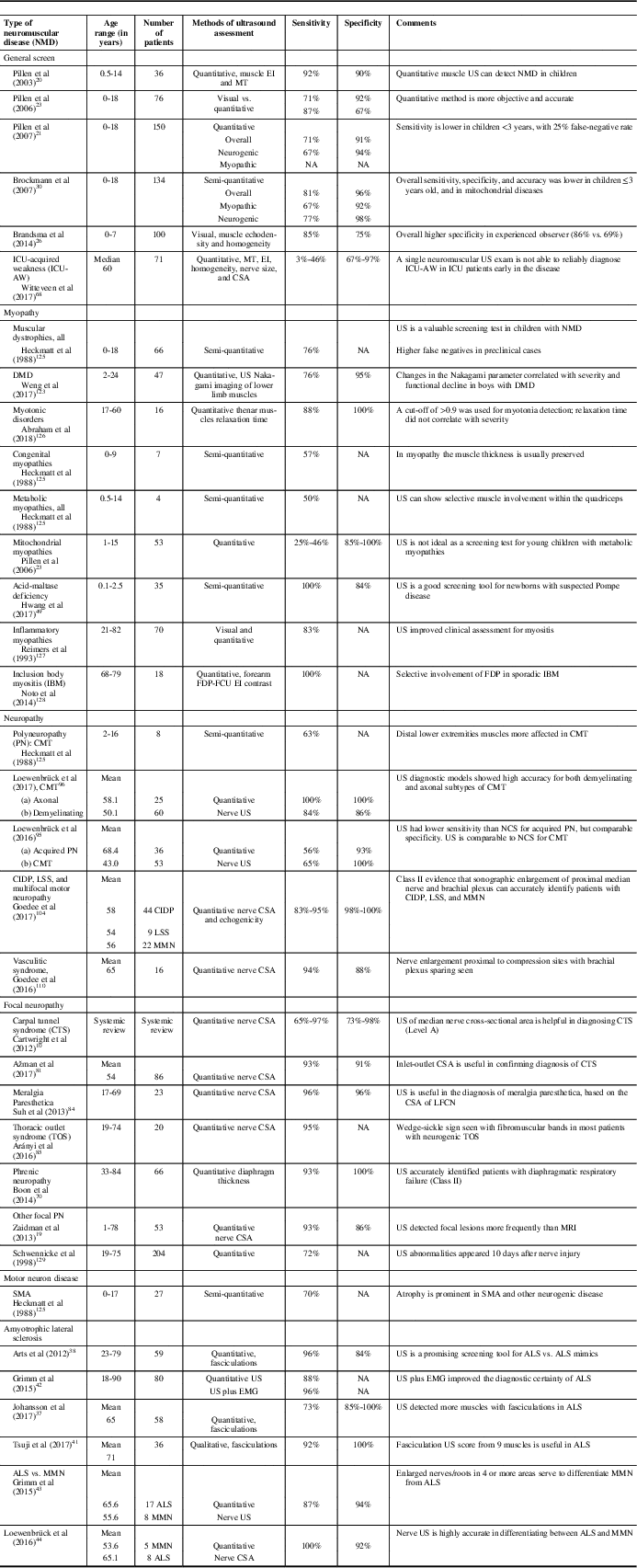
ALS=amyotrophic lateral sclerosis; CIDP=chronic inflammatory demyelinating polyneuropathy; CMT=Charcot-Marie-Tooth disease; CSA=cross-sectional area; DMD=Duchenne muscular dystrophy; EI=echointensity; EMG=electromyography; FCU=flexor carpi ulnaris; FDP=flexor digitorum profundus; IBM=inclusion body myositis; ICU=intensive care unit; LFCN=lateral femoral cutaneous nerve; LSS=Lewis-Sumner Syndrome; MMN=multifocal motor neuropathy; MT=muscle thickness; NA=not available; NCS=nerve conduction study; PN=polyneuropathy; SMA=spinal muscular atrophy; US=ultrasound.
Conclusion
This review has focused on the clinical applications of nerve and muscle ultrasound. It has shown that neuromuscular ultrasound can provide added diagnostic values in both adult and pediatric neurology practice. Ultrasound can be used as a point-of-care and patient-friendly screening tool for many peripheral nervous system disorders, and it may obviate the need for more invasive testing. It is expected that over the next 5-10 years, neuromuscular ultrasound will play a definitive role in the diagnostic workup for many of these patients.
Acknowledgments
The authors thank their colleague Dr. Sigrid Pillen for her contributions to the artwork.
Disclosures
The authors have nothing to disclose.
Statement of Authorship
JKM analyzed the literature and drafted the manuscript; NvA performed the literature search, critically revised the manuscript for content and provided the images.