- 1Lydia Becker Institute of Immunology and Inflammation, Division of Immunology, Immunity to Infection and Respiratory Medicine, Faculty of Biology, Medicine and Health, University of Manchester, Manchester Academic Health Science Centre, Manchester, United Kingdom
- 2Manchester Centre for Clinical Neurosciences, Salford Royal Hospital, Salford, United Kingdom
Autoimmune diseases are characterized by a significant sex dimorphism, with women showing increased susceptibility to disease. This is, at least in part, due to sex-dependent differences in the immune system that are influenced by the complex interplay between sex hormones and sex chromosomes, with contribution from sociological factors, diet and gut microbiota. Sex differences are evident in the number and function of lymphocyte populations. Women mount a stronger pro-inflammatory response than males, with increased lymphocyte proliferation, activation and pro-inflammatory cytokine production, whereas men display expanded regulatory cell subsets. Ageing alters the immune landscape of men and women in differing ways, resulting in changes in autoimmune disease susceptibility. Here we review the current literature on sex differences in lymphocyte function, the factors that influence this, and the implications for autoimmune disease. We propose that improved understanding of sex bias in lymphocyte function can provide sex-specific tailoring of treatment strategies for better management of autoimmune diseases.
Background
Autoimmune diseases occur in those who are genetically susceptible, with contributions from environmental and biological factors, resulting in subsequent gene-environment interactions (1). There is wide disparity in the epidemiology of autoimmune diseases between males and females, with variation seen in prevalence, age of onset, phenotype, disease severity, and response to treatment (Table 1) (2–4, 8, 11–13, 16). Women mount stronger innate and adaptive immune responses than men, which provides a survival advantage to infectious diseases (32), but also a four times increased likelihood of developing autoimmunity (33, 34). Despite this, sex differences are often not fully investigated or acknowledged in immunological research and treatment strategies. Animal models of autoimmune diseases have historically been predominantly male, resulting in male-biased data influencing treatment decisions, and dual-sex immunology studies frequently failing to explore sex differences (35). More recently, there has been a drive to better understand sex differences in the immune response and distinguish between the effects of hormones and genetic factors. This has resulted in the identification of numerous sex differences in both the innate and adaptive arms of the immune system.
Lymphocytes play a central role in the development and progression of autoimmune diseases. Defects in immune tolerance mechanisms result in the expansion of autoreactive T cells and autoantibody-producing B cells leading to target organ damage and dysfunction (36). Hundreds of genes are differentially expressed between male and female lymphocytes (37). The X chromosome is significantly bigger and more complex than the Y chromosome, and contains many genes relating to immune function, including toll-like receptors (TLRs), cytokine receptors, and transcription factors (38, 39). During embryogenesis, X chromosome inactivation (XCI) randomly silences one of the X chromosomes in females. Around 15% of genes escape XCI in women, particularly those on the short arm which is evolutionarily a more recent addition, and are therefore expressed bi-allelically (40, 41). Incomplete or skewed XCI results in over-expression of X immune-related genes and micro RNAs (mRNA) in female lymphocytes, and is associated with autoimmune diseases (41–47).
Furthermore, lymphocytes express cell membrane and nuclear sex hormone receptors, signaling through which can cause epigenetic and transcriptional changes, thereby regulating the function of the immune system (48, 49). Nuclear hormone receptors, upon engagement, can upregulate the expression of several key immune related genes either directly by binding to hormone response elements in the promoter region, or indirectly by binding to transcription factors which then bind to the gene promoters (49–51). Sex hormone receptors on the cell membrane can activate cytoplasmic signaling and cause signal transduction effects via mechanisms such as rapid calcium fluxes (49–51).
The full extent and implication of the sex differences in lymphocytes are yet to be fully elucidated. It is essential to continue to better understand and acknowledge these differences, in order to advance the knowledge of autoimmune disease pathophysiology, and to allow the development of tailored sex-specific therapies. This review focuses on sex differences in lymphocytes, and the implications this has for autoimmune disease pathophysiology.
Sex bias in T cells
Development and maturation
Sex-dependent differences in the frequencies of T cells have been reported by multiple independent studies. Females have been shown to display higher frequencies of naïve T cells, cluster of differentiation (CD)4 T cells and higher CD4:CD8 T cell ratios compared to males of the same age (52–55). Conversely, males display higher frequencies of CD8 T cells, and a higher CD8:CD4 T cell ratio (52–55). These differences in T cell subset frequencies have been shown to be influenced by sex hormones (51, 55). For instance, castration of male mice results in thymic enlargement, increased output of naïve, CD4 and CD8 T cells, and increase in autoimmune disease susceptibility, as observed in female mice, indicating that testosterone has a suppressive effect on lymphopoiesis (56, 57). These effects of androgens on T cells are observed largely in early development, as T cells lose expression of androgen receptors (ARs) upon maturation. In contrast, estrogen receptors (ERs) continue to be expressed by T cells at later stages of development (58). The effects of estrogen on T cell populations are dose dependent. Whereas low doses have been shown to expand CD4 T cells (59), high doses (observed during pregnancy) reduce CD4 and CD8 T cell numbers (60).
Proliferation, differentiation and function
T cells can be classified into functionally distinct subsets based on the cytokines they produce. These subsets include interferon (IFN)-γ/interleukin (IL)-2/tumor necrosis factor (TNF)-α-producing T helper 1 (Th1) cells, IL-4/IL-5/IL-13-producing Th2 cells, IL-17/IL-22-producing Th17 cells and regulatory T cells (Tregs) (61). Oestrogen receptors (ER) have two main subtypes, -α and -β, with differing effects upon the T cell milieu. Activation of ER-α, but not ER-β, results in thymic atrophy, drives enhanced T cell activation and proliferation, and alters T cell subset frequencies (55, 60, 62, 63). In contrast, ER-β promotes apoptosis via inhibition of B cell lymphoma (Bcl)-2 signaling and inhibits inflammatory genes such as IFN-γ (64, 65). ER-α and -β expression also differs between T cell subsets; both higher in CD4 than CD8 T cells (60, 66).
The Th1/Th2 balance in women shifts with hormonal changes observed during the menstrual cycle and pregnancy (Figure 1). Low concentrations of estrogen seen in pre-ovulatory females leads to upregulation of the IFN-γ promoter (67), interferon regulatory factor 5 (IRF5) (68, 69), TLRs (70), and T-bet (60, 71, 72). This promotes an expansion of antigen-specific CD4 T cell responses and a Th1 shift, with increased production of IFN-γ (60, 71, 73). In contrast, during the luteal phase of the menstrual cycle, when estrogen levels increase, a Th2 shift is seen, with increased IL-4 and IL-10 and decreased IFN-γ production (74, 75). This Th2 shift has been shown to be via ER-α driven increase in GATA-3 expression (76). Very high levels of estrogen and progesterone that occur in pregnancy further promote Th2 immunity, in order to promote fetal tolerance, with increased production of IL-10 and reduced IL-2, IL-12, and IFN-γ (77–81). The mechanism of these dose-dependent effects remain to be elucidated.
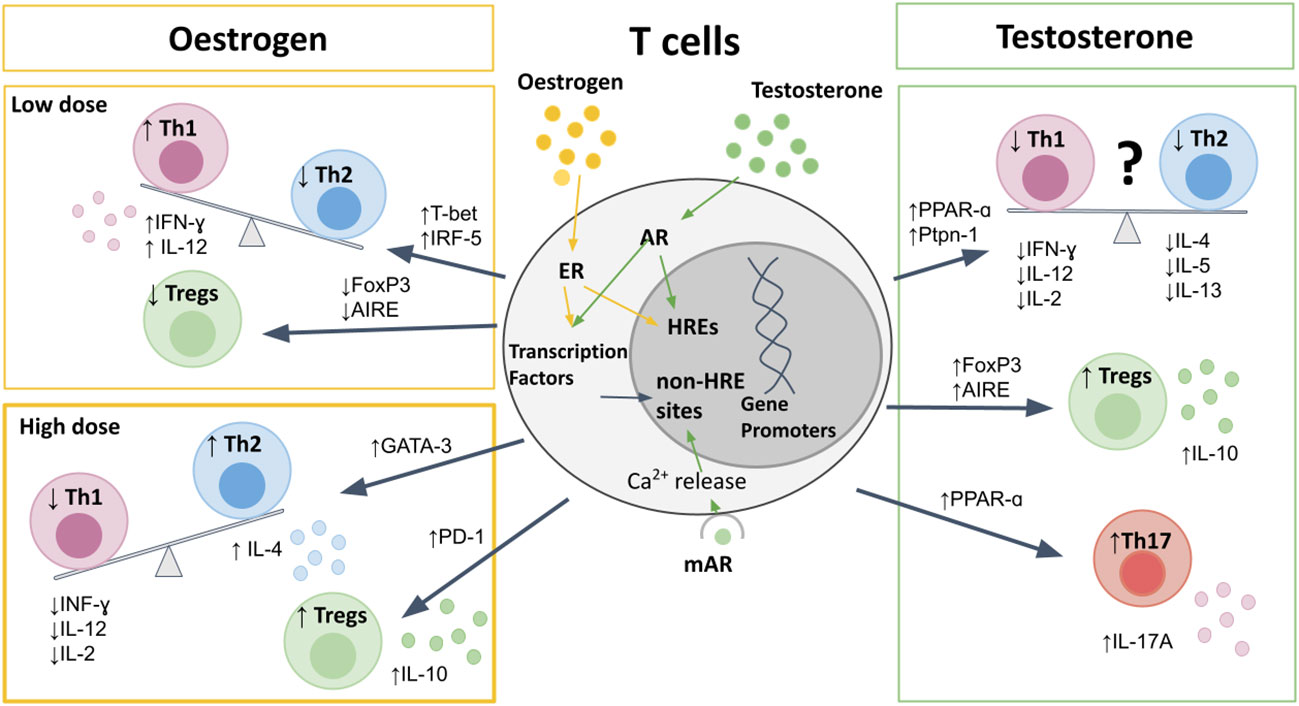
Figure 1 The effects of sex hormones on T cells. Sex hormones bind to nuclear receptors, leading to direct binding to HREs, or indirect effects on gene promotors through transcription factors. Oestrogen at pre-ovulatory concentrations upregulates T-bet and IRF5 to cause a Th1 shift, and downregulates AIRE and FoxP3 to reduce Tregs. High-dose estrogen during pregnancy leads to a Th2 shift, via GATA-3, and promotes Treg expression and PD-1 expression. Testosterone upregulates PPAR-α, and Ptpn-1 leading to reduced Th1 responses, but also suppresses Th2 cytokine production, and therefore the Th1/Th2 bias is less clear. Testosterone does increase Th17 production, as well as increasing expression of FoxP3 and AIRE to promote Treg differentiation and increased IL-10 production. AIRE, autoimmune regulator; AR, androgen receptor; ER, estrogen receptor; FoxP3, Forkhead-box-P3; HREs, hormone response elements; IFN, interferon; IL, interleukin; IRF, interferon regulatory factor; mAR, membrane androgen receptor; PPAR, proliferator-activated receptor; Ptpn, protein-tyrosine phosphatase; Th, T-helper; Treg, regulatory T cell.
There is conflicting data as to the direction of any Th1/Th2 bias in males when compared to females (82–84). On one hand, some studies have described a predominantly Th1 response in males, with a more pronounced Th2 response in females (82, 85). When comparing Th1 and Th2 cytokines from 20 women and 15 men, Escobar and colleagues reported a higher production of IFN-γ and IL-2, mirrored by reduced IL-10 and IL-4 in men compared to women (82). On the other hand, several other studies in humans and mice have demonstrated decreased frequencies of Th1 in males compared to females (60, 84, 86–89). Moreover, testosterone has been shown to upregulate the expression of peroxisome proliferator-activated receptor (PPAR)-α (90), and protein-tyrosine phosphatase (Ptpn)-1 (88, 91), both of which inhibit Th1 differentiation. This is further supported by studies showing that castration of mice results in upregulation of IFN-γ and T-bet expression (91, 92). The contrasting data may be due to variability between species and different disease settings (89, 93), as well as partly due to the dose-dependent effects of estrogen on the Th1/Th2 balance resulting in a constantly shifting bias between men and women. To further complicate the picture, androgens have also been shown to reduce Th2 cytokines IL-4, IL-5, and IL-13 (94–97), and consistently enhance production of IL-10 from T cells (86, 88, 98, 99).
Although upregulation of PPAR-α expression by androgens suppresses Th1 responses, this has been shown to promote Th17 responses in males compared to females (83, 100). The effects of estrogen on Th17 responses are less clear, likely due to differences in estrogen receptor (ER-α vs. ER-β) signaling on Th17 differentiation and function. ER-α signaling has been shown to promote Th17 responses, as ER-α deletion inhibits Th17 differentiation in a mouse model of colitis (101). Moreover, ER-α signaling has been shown to increase production of IL-17A by Th17 cells by promoting mitochondrial respiration, proliferation and by upregulating IL-23R expression (102). In contrast, ER-β signaling in CD4 T cells has been shown to suppress Th17 cell differentiation, as well as promote the induction and maintenance of Tregs (103, 104). Progesterone also suppresses Th17 differentiation, resulting in reduced Th17 responses during pregnancy (4).
Men display higher frequencies of Tregs compared to women due to both genetic and hormonal influences (105). Regulatory protein forkhead box P3 (FoxP3) expression has been shown to be higher in males due to parental imprinting effects, with less methylation on the maternal X allele, which is expressed exclusively in males (106). Additionally, a recent study has demonstrated that Treg frequencies are one of the top features of immune cell profiling that differentiate young post-pubertal men from women, and that male Tregs have higher suppressive capabilities due to increased phosphoinositide 3-kinases (PI3K) signaling (107). The autoimmune regulator (AIRE) gene, which is critical for Treg development, is downregulated by estrogen and progesterone and upregulated by testosterone, thereby resulting in increased thymic expression of AIRE in males compared to females (108). Furthermore, androgens also promote increased expression of the FoxP3 (109), whereas ER-α signaling downregulates FoxP3 expression (101).
Treg numbers fluctuate during the menstrual cycle with maximum expansion in the late follicular phase when estrogen levels are high and the woman is most fertile (110), in order to promote tolerance for a potential pregnancy (110). During pregnancy high levels of estrogen and progesterone further promote Treg differentiation and upregulation of the immunosuppressive molecule programmed cell death protein (PD)-1 (111–117).
Sex bias in B cells
Development and maturation
Females display higher frequencies of B cells than males (55), along with enhanced B cell survival, maturation and class switching (118–121). Although both androgens and estrogens can suppress B cell lymphopoiesis (122), only testosterone reduces numbers of B cells in the bone marrow (56, 58). Estrogen promotes hematopoiesis within the liver, which may lead to autoreactive lymphocytes which escape central tolerance mechanisms (123). Oestrogen also interferes with negative selection, resulting in increased survival and expansion of high-affinity autoreactive B cells (118, 124, 125). Studies using ER-α and ER-β deficient mice have shown that engagement of either receptor can affect B cell maturation, but only ER-α engagement can drive an increase in autoreactive B cells (126). In contrast to T cells, B cells express more ER-β than -α (127), however ER-α expression is around four fold higher in B cells than T cells, with higher expression in females compared to males (128), suggesting another mechanism by which females are prone to autoimmunity.
Proliferation, differentiation and function
Testosterone inhibits proliferation and differentiation of B lymphocytes by reducing B-cell activating factor (BAFF) production by macrophages and through altering key modulators of apoptosis including downregulation of B cell lymphoma (Bcl)-2 and nuclear factor kappa-light-chain-enhancer of activated B cells (NFκB) (Figure 2) (49, 129, 130). Conversely, estrogen enhances B cell proliferation, suppresses apoptosis, and promotes survival of autoreactive B cells via increased expression of survival regulators BAFF, Bcl-2, CD22, and SH2 containing protein tyrosine phosphatase (SHP)-2 (118, 122, 131–133). Oestrogen also increases expression of CD80, a co-stimulatory signal important for B cell activation (71). In mouse models of arthritis, higher frequencies of IL-10-producing regulatory B cells (Bregs) that exhibit immune suppression have been reported in males compared to females (134). However, the sex bias in Breg frequency and function between men and women in health requires further study.
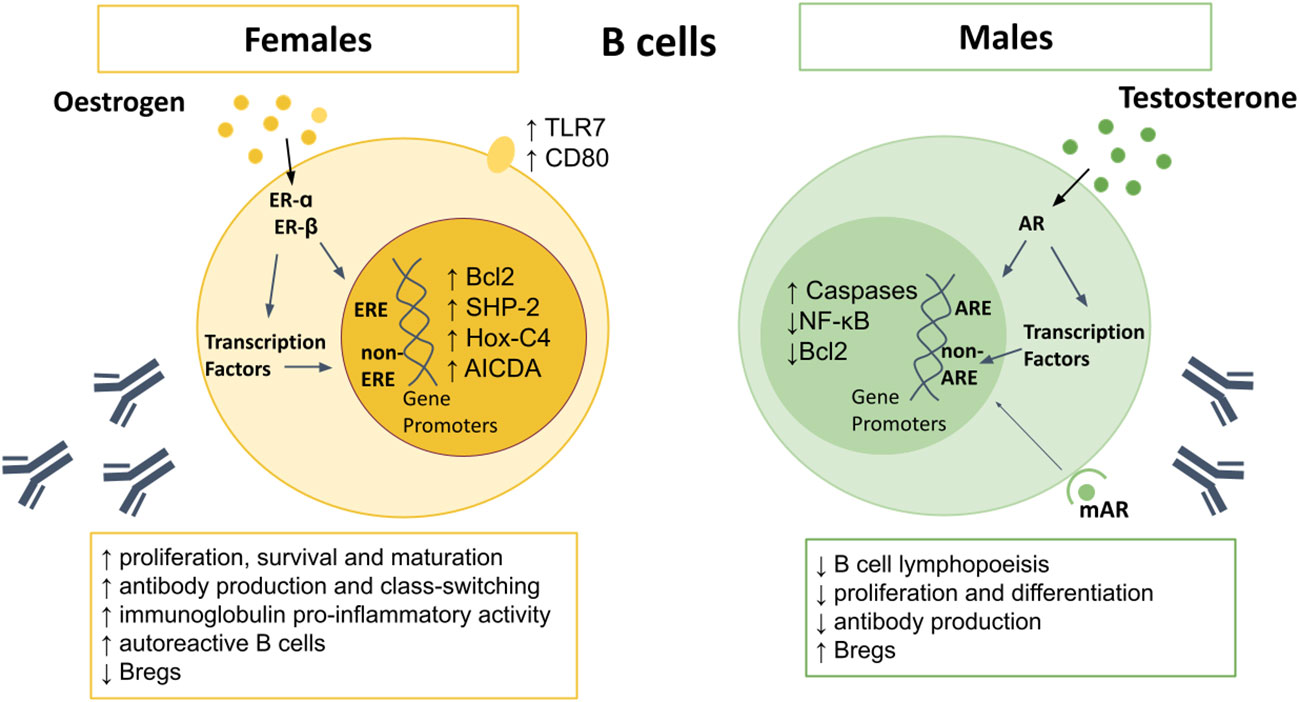
Figure 2 Sex differences in B cells. AR signaling appears to upregulate caspases, and downregulate NF-κB and Bcl2, resulting in increased B cell apoptosis. Males also demonstrate reduced B cell lymphopoiesis in the bone marrow, reduced proliferation and differentiation of B cells, and lower antibody production, but higher Bregs. Oestrogen upregulates Bcl-2, SHP-2, Hox-C4, and AICDA, as well as surface expression of TLR7 and CD80. Female B cells demonstrate higher proliferation, survival and maturation, higher pro-inflammatory antibody production, leading to higher levels of autoreactive B cells. AICDA, Activation-induced cytidine deaminase; AR, androgen receptor; Bcl-2, B cell lymphoma 2; Bregs, regulatory B cells; Hox-C4, homeobox protein C4; IFN, interferon; IL, interleukin; NF-κB, nuclear factor kappa-light-chain-enhancer of activated B cells; SHP-2, Src homology region 2 domain- containing protein tyrosine phosphatase; TLR, toll-like receptor.
Immunoglobulin production
Greater antibody responses and higher basal immunoglobulin (Ig) levels are seen in women (55, 135). Circulating levels of IgM, and in some studies IgG, are higher in females than males (136–138), and are even higher in women with three X chromosomes (139). Higher expression of the X chromosome encoded gene TLR7 in females results in increased IgG class-switching and higher TLR7-driven plasma cell proliferation (140, 141). Oestrogen promotes differentiation of B cells into antibody-secreting cells, with highest frequencies observed just before ovulation, when estrogen is at its peak (142). This is likely an evolutionary attempt to reduce the risk of infection and maximize the chance of conception. Testosterone has the opposite effects and lowers antibody production (122, 135, 143). Moreover, mice with AR-deficient B cells display increased autoantibody production (130).
Activation-induced cytidine deaminase (AICDA) is required for somatic hypermutation and class switch recombination, mechanisms which are important for Ig gene modification in B-cells and antibody production (144). AICDA is upregulated in B-cells by estrogen, due to increased expression of homeobox protein (Hox)-C4, a transcriptional regulator of AICDA (122, 145), explaining the higher antibody production in females to males. However, during pregnancy, progesterone inhibits AICDA transcription, with subsequent reduction in somatic hypermutation and class switch recombination (146), resulting in reduced antibody production.
Sex bias in lymphocytes in autoimmune diseases
It is important to consider the implications of sex differences in lymphocytes within the context of specific autoimmune diseases. Variation in lymphocyte responses between males and females influence disease susceptibility and severity in differing ways dependent upon disease mechanism. Although less common, some autoimmune diseases have a slight male bias, such as late onset myasthenia gravis (LOMG) (1.5:1), ankylosing spondylitis (1.1:1), myocarditis (3.3:1) and type 1 diabetes mellitus (1.2:1) (see Table 1) (14, 21, 24, 25). Males who do develop female predominant autoimmune diseases, such as rheumatoid arthritis (RA) and systemic lupus erythematosus (SLE), have lower androgen concentrations than controls, which likely skews their lymphocyte profile towards autoimmunity (147–149). Additionally, older men are at higher risk of loss of central tolerance mechanisms associated with thymic involution, as seen in LOMG (150). Here we compare and contrast sex differences identified in four common autoimmune diseases; SLE, multiple sclerosis (MS), RA, and myasthenia gravis (MG).
Systemic lupus erythematosus
SLE is a systemic autoimmune condition that can affect multiple organs and tissues and is characterized by autoantibodies directed towards a wide spectrum of cellular components, most commonly anti-nuclear antibody (151). It is a highly heterogeneous disease that can cause symptoms ranging from malar rash and fever to arthritis and nephritis (152).
The expression of miRNAs and immune related genes associated with SLE are frequently higher in females, due to effects of skewed XCI, partial reactivation, DNA demethylation, and estrogen binding (153–156), contributing to the female predominance of the disease. Several upregulated miRNAs target CBL, which increases T cell activation and accelerates T cell activation-induced cell death (153–156). Mature naïve T and B lymphocytes from females with SLE display higher expression of CXCR3, which drives Th1 and Th17 infiltration into the kidney in lupus nephritis (157, 158), and CD40LG, which promotes IgG production (43, 159) (160). TLR7 and CXorf21 (also termed TASL; TLR adapter interacting with SLC15A4 on the lysosome) also escape XCI (140, 141, 161–163), resulting in higher production of IFN-α, which is critical in driving the pathogenesis of SLE (164, 165).
Additionally, different types of lupus nephritis appear to be driven by differing cytokine profiles. Whereas Th1-mediated inflammation promotes diffuse lupus nephritis, seen more commonly in males, Th2 responses result in membranous lupus nephritis, which is more common in females (166, 167). Skin lesions in lupus, also more common in females, are triggered by Th2 cells, which subsequently transition to a Th1-like phenotype as a result of increased TLR7 signaling (168, 169). Therefore, it may be beneficial to target Th1 responses in males and TLR7 in females with SLE.
Oestrogen has been shown to accelerate disease in mouse models of lupus, by driving Th1 and Th2 responses via ER-α signaling (63, 170–172). Binding of ER-α also leads to upregulation of TLR8 and IRF5, amplifies production of IFN-α/β, and promotes survival, expansion and activation of autoreactive B cells in SLE (63, 68, 171, 173, 174). The increased production of IFN-α in females has been shown to increase ER-α expression on murine splenic cells, resulting in a positive feedback loop that promotes inflammation (128). ER-α and -β engagement results in a dose-dependent increase in activation of T cells from SLE patients, with increased calcineurin and CD40L expression (175, 176). Overall, it appears that ER-β has an immunosuppressive effect on disease (63). This is further supported by a study demonstrating that patients with SLE have lower ER-β expression, but similar ER-α, in T cells compared to healthy controls (177).
Whereas estrogen has been shown to accelerate disease, androgens administered to mouse models of lupus have been shown to ameliorate disease (174, 178, 179). There are low levels of androgen and progesterone in bodily fluids of SLE patients compared to controls, and higher levels of estradiol, possibly due to increased conversion of androgen precursors to estrogen (147, 148). SLE-related immune complex-mediated glomerulonephritis has been linked to a switch from predominantly IgM anti-ds DNA, to IgG2a and IgG2b, which occurs earlier in disease in females than males and can be delayed by androgen administration (180, 181).
Sex hormone signaling appears to be dysregulated in SLE. In contrast to promoting Treg differentiation in healthy females, estrogen exposure in vitro inhibits Treg differentiation in peripheral blood mononuclear cells (PBMCs) from SLE patients (148). This may explain the worsening of disease that is seen with pregnancy in SLE, in contrast to improvements in most other autoimmune diseases during pregnancy (17). This reduction in Treg frequencies seen in SLE patients has been linked to the increased risk of pregnancy-related morbidities, including fetal loss, growth restriction, preterm birth, and pre-eclampsia (182).
Taken together, these studies suggest that chromosomal effects resulting in higher expression of SLE-related genes and miRNAs, in combination with dysregulated ER-α signaling, promote T cell activation and autoantibody production, alongside Treg inhibition. While further studies are required to better understand mechanisms of sex differences in SLE, there is sufficient evidence to conclude that alterations in lymphocyte function contribute to the profound female sex bias in SLE.
Multiple sclerosis
MS causes a wide range of neurological symptoms, and potentially significant disability, due to inflammation and demyelination of white matter in the central nervous system (CNS) (9). Although women are more likely to develop MS, men are at a higher risk of developing more severe disease (183). MS and its mouse model experimental autoimmune encephalitis (EAE) are considered to be myelin protein-specific Th1- and Th17-mediated diseases (184, 185). Sex differences in cytokine production have been demonstrated in EAE, with greater Th1 activation seen in females, and increased Th17 responses in males (83, 186, 187).
Kdm6a is a histone demethylase on the X chromosome that regulates transcription of numerous genes. It has been identified as one of the top differentially expressed genes on CD4 T cells between males and females, with higher expression in females due to escape from XCI (107, 188). Mice lacking Kdm6a in CD4 T cells have been shown to display a downregulation of neuroinflammation, TLR signaling and IL-17 signaling genes, and are protected from EAE (188), suggesting that this is an important mechanism to explain the female sex bias in MS.
Both estrogen and testosterone display protective effects in MS, but via differing mechanisms. Androgen administration to EAE mice has been demonstrated to protect against disease through inducing a Th2 bias (86, 87, 189), which is dependent upon AIRE (108). Unlike what is observed in health, estrogen administration in EAE inhibits Th1 and Th17 responses via ER-α binding to suppress disease (103, 104, 190). Oestrogen also promotes B cell migration away from the target organ by upregulating the expression of chemokine CXCL13 (191), which acts through its receptor CXCR5 (widely expressed on mature B cells (192)). Further beneficial effects of estrogen in MS include expansion of PD-L1-expressing Bregs as well as upregulation of PD-1 on Tregs (117, 191), which leads to further downregulation of Th17 responses (117, 191, 193, 194).
Women with MS display a reduction in the incidence of relapses during pregnancy, followed by an increased risk of relapse post-partum (195). In the third trimester, high levels of estrogen modulate CD4 T cell function by promoting a decrease in Th17 cells mirrored by an expansion of Tregs (196). This protective effect is further enhanced by ER-α signaling in Bregs, potentiating Treg activity (197, 198). Additionally, progesterone has also been shown to suppress EAE by promoting IL-10 production by B cells (144, 198). In the post-partum period, B cells from MS patients preferentially develop into plasma cells (199). Furthermore, activated memory T cells from relapsing MS patients produce more pro-inflammatory cytokines when compared to non-relapsing patients and healthy controls postpartum (200).
To summarize, MS is more common in females that males, due to genetic effects such as increased expression of Kdm6a. However, in contrast to its role in SLE, estrogen has immunosuppressive effects on T and B lymphocytes and ameliorates disease.
Rheumatoid arthritis
RA is a chronic inflammatory disease that results in pain, swelling, stiffness and deformity of multiple joints (5). RA is around three times more common in females than males and has a peak age of onset around the time of menopause in women, but occurs towards later life in men (6). The onset of RA is associated with the post-menopausal and postpartum periods, suggesting links with falling levels of female sex hormones (6, 10, 201). Of note, men with RA have lower androgen concentrations and higher estrogen levels than controls (149).
Nearly all human autoimmune diseases have been linked to specific human leukocyte antigen (HLA) genes, mainly haplotypes in major histocompatibility complex (MHC) class II (202). It is suggested that these haplotypes predispose to autoimmunity by presenting many peptides, with specific haplotypes linked to differing cytokine profiles (203). HLA-DR4 is associated with RA and supports a female sex bias (134, 204). Female HLA-DR4-transgenic mice display more robust antigen presentation by B cells than males, and increased IL-4 and IL-13 production, which further promotes B cell responses (202, 204). In contrast, HLA-DR4 males produce more anti-inflammatory IL-10 and have higher numbers of Tregs and Bregs (204). Treg frequencies decrease following castration of these male mice supporting immunosuppressive effects of androgens (204).
Oestrogen supplementation to mice with collage-induced arthritis limits disease development and severity by reducing IFN-γ and TNF-α production, as well as by shifting autoantibody isotype from IgG2a to IgG1 (205–207). In addition, estrogen decreases Th17 cells in the joints during established arthritis by promoting their migration to lymph nodes, via ER-α-mediated upregulation of CCR6 on Th17 cells and CCL20 in lymph nodes (206). Androgens also suppress RA, in part due to inhibition of NF-κB and subsequent reduction in IL-6 (208, 209).
The T cell co-stimulatory molecule CD2 is involved in T cell activation, as well as Th17 and Treg differentiation (202). CD2 is associated with RA and is expressed at higher levels in the RA synovium and healthy PBMCs of women than men (210). In addition, it has recently been demonstrated that a polymorphic ER-binding site regulates expression of surrounding genes, including CD2, in a sex-specific manner, resulting in sexually dimorphic T cell responses (202).
Altogether, these studies implicate a significant role for genetic factors, such as HLA type, along with sex hormones for the sex-specific lymphocyte differences in RA.
Myasthenia gravis
In MG pathogenic autoantibodies cause neuromuscular junction transmission failure and muscle weakness, which can be life-threatening (19). Interestingly, the age of onset is a critical determinant of sex bias in MG. In female predominant early-onset MG (EOMG; <50 years), there is lymphoid follicular hyperplasia (LFH) and germinal centers containing numerous B cell clones within the thymus (150, 211). Thymocytes and circulating T cells from patients with MG express more ER-α and -β than controls (66). The upregulation of ERs has been shown to be a result of increased pro-inflammatory cytokines IFN-γ, IL-1 and TNFα (66), that likely enhance reactivity to estrogens, and promote B cell responses. Furthermore, estrogen exposure in experimental autoimmune MG has been shown to enhance Th1 responses, and production of IgG2a and IgG2b autoantibodies, as well as exacerbate disease (212).
In male predominant late-onset MG (LOMG), the thymus is atrophied, and autoimmunity is thought to result from a lack of AIRE, along with a loss of muscle-like myoid cells, resulting in a failure of negative T cell selection to muscle-specific antibodies (213–215). Thymic involution is known to progress at a faster rate in males than females, and in LOMG there is a decrease in naive cell export from the thymus, with a trend to lower export in males, perhaps in part explaining the male bias to disease (213, 216).
Genetic studies have also demonstrated sex-specific HLA alleles to associate with the different subtypes of MG. HLA-B*08 and HLA-DR3 associate with EOMG, with a stronger association in females (217, 218). In contrast, LOMG is associated with HLA-B7 and/or HLA-DR2 alleles in males but not females (218, 219).
Factors that influence sex bias in lymphocytes
Understanding and acknowledging sex bias needs to be at the forefront of future immunological research. There are several additional factors detailed below that should be taken into consideration, due to their potential influence on sex differences in lymphocytes.
Hormonal influences
Hormonal effects vary between autoimmune diseases, as detailed in the previous sections. As such, differences in exposures to hormonal therapies between the sexes also needs to be considered in the context of specific autoimmune diseases. For instance, high-dose estrogen supplementation in female MS patients reduces IFN-γ expression by PBMCs, and results in an improvement in disease (185). In contrast, in SLE, use of hormonal contraceptives may increase risk of developing disease, and hormone replacement therapy use is linked to an increase in mild-moderate disease flares (220, 221).
Male patients with MS treated with testosterone displayed reduced CD4 T cells, increased NK cells, reduced IL-2 production from PBMCs, and reduced brain atrophy (222, 223). Gonadotrophin-releasing hormone (GnRH) antagonists, used in prostate cancer treatment, reduce testosterone levels. GnRH antagonists block thymocyte proliferative responses and reduce numbers of CD4 T cells, Tregs and B cells (224–226). GnRH agonists have also been shown to exert sexually dimorphic actions in an SLE mouse model, with worsening of disease seen only in females, potentially due to gender differences in the expression of G proteins on immune cells (227). Worsening of MS in females has also been reported with their use (228–230).
Ageing
Immunosenescence that occurs with age results in both a reduced functional capacity of the immune system to protect against infection as well as enhanced immune-mediated inflammation, commonly known an “inflammaging” (231, 232). This decline in immune function occurs earlier in men than women, but the rate of decline is faster in women upon onset, paralleling changes in sex hormone levels, and making the sex difference in lymphocytes more divergent (233–236). In addition to hormonal changes, sex-based differences in the aging of lymphocytes have been attributed to increased skewing of XCI in females (237), differences in mitochondrial dysfunction (238), telomere shortening (239), chromatin accessibility (233) and demethylation (240).
As T cells age, there is a sex difference in the upregulation of genes related to inflammaging. Older females display higher proinflammatory responses and weaker T cell mediated defenses compared to age-matched males (236, 238). There is an increase in memory/activated T cells, and an increased CD4:CD8 T cell ratio, with a greater rate of increase in women (52, 241–243). Women also demonstrate a later but stronger rise in IL-6 than men (244). IFN-γ transiently increases in the early postmenopausal period in women (237, 245, 246), before falling to its lowest level in late menopause (247). In contrast, IFN-γ levels have been seen to either decline or not change with age in healthy men (232, 246). In older men with inflammatory or autoimmune diseases however, IFN-γ and IL-17 production by T cells is higher than younger men, which increases with disease severity, a pattern not seen in older women with the same diseases (232).
Although females have more antibody-producing plasma cells than males, this trend has been shown to weaken with age (235). Further, a subset of dysfunctional B cells known as age-associated B cells (ABCs), are associated with autoantibody production and are expanded in elderly females (248–250). It is proposed that overexpression of TLR7 in females may lead to accumulation of this autoantibody-producing B cell subset (251). In patients with SLE, IgD-CD27- double negative (DN) B cells, which are similar to ABCs, are expanded and display hyper-responsiveness to TLR7 (252, 253).
Overall, sex-specific changes in lymphocytes with age are complex and not yet fully understood, however, provide some explanation as to why autoimmune disease susceptibility differs between men and women with age.
Interaction with the target organ
Sex differences have been demonstrated in lymphocyte interaction with target organs of various autoimmune diseases (189, 242, 243, 254, 255). These are influenced by sex differences in target organ structure and cell death pathways, as well as sex hormone interaction with the target tissue. Despite increased prevalence of autoimmune disease in women, in many conditions males appear to be prone to greater target organ damage. This is demonstrated by increased apoptosis of thymocytes and thymic atrophy in male rodents than in females given lipopolysaccharide, which binds to TLR4 on macrophages to trigger a pro-inflammatory cascade promoting a Th1 response (256, 257).
In MS, male sex is a risk factor for poor outcome and increased brain atrophy (183). This is likely to be, in part, due to sex differences in miRNA expression within the brain (258, 259), differences in blood-brain barrier permeability (254), and the ability of estrogen to exhibit neuroprotective effects (260). Studies in hypoxic brain injury and stroke have demonstrated differences in Treg responses, and their effects upon cerebral infiltration and brain injury between men and women, resulting in Treg-mediated neuroprotection in females but increased neurodegeneration in males (255, 261).
In contrast to MS, female patients with RA on TNF-α inhibitors display lower remission rates in comparison to males (34, 262). This may be explained by the higher expression of CD2 in the synovium of females; in vitro deletion of CD2 has been shown to reduce the damaging effects of TNF-α on synovial cells (210, 263). In addition, there is thought to be a male-biased benefit of anti-TNF-α-driven reduction in the peripheral conversion of steroid precursors to estrogen in synovial tissues in RA (264–266), levels of which correlate with inflammation (149). Finally, synovial secretion of IL-6 and IL-8 positively correlate with the expression of ER-α and ER-β, but not AR (267), suggesting that estrogen signaling might contribute to synovial inflammation in females.
Differences in the innate immune system
Differences in the innate immune system perpetuate the more robust immune responses seen in females. Neutrophils and macrophages have higher phagocytic activity in females than males (268), and female antigen presenting cells are more efficient at presenting peptides (269). Testosterone reduces expression of TLR4, reduces synthesis of TNF, and increases BAFF, IL-10, IL-12 and TGF-β production from macrophages (88, 129, 270, 271). Low dose estrogen activates dendritic cells (DCs) (272, 273), whereas (272, 273)high dose estrogen increases DC tolerance, reduces proinflammatory cytokine production and increases IL-10 and TGF-β mRNA expression (274). Sex differences in the complement system have also been demonstrated, including increased activity of the alternative pathway in males (275, 276), contrasting to a greater dependence on the lectin pathway in females (277). Female mice have been shown to display reduced susceptibility to complement-mediated damage, possibly due to differences in terminal pathway components (278). It is therefore unclear why complement-mediated diseases are more common in women, but this may contribute towards the increased male-susceptibility to target organ damage.
Gut microbiome
Mouse studies have shown that differences in the gut microbiome, with influence from sex hormones, contribute to sex differences in the immune response. This has been well summarized in a recent review (279). Conflicting data exists in human studies, in which it is harder to account for the multiple confounding factors. However, sex differences have been shown in the gut microbiome in numerous studies, with influence from sex hormones (280–282). Thus, its influence upon autoimmune disease and mechanisms to modulate this requires further investigation.
Other factors
In addition to genetic and hormonal factors, sex differences in exposure and response to environmental factors including sociological differences, psychological stress, diet, obesity, vitamin D, smoking, and viral infections may also impact upon the sex differences in lymphocytes (283–288). It is likely that these factors contribute towards the increasing incidence seen in autoimmune diseases by 3 to 9% each year (1).
Women are more likely to develop cross-reactive T and B cells to foreign stimuli such as cigarette smoking, viruses, and dietary peptides, which can act as triggers for autoimmune disease (289–291). Females are also more likely to be obese, with higher amounts of leptin produced by their adipocytes. This promotes pro-inflammatory Th1 responses and inhibits Tregs (292–294). In patients with MS, the immunomodulatory effects of vitamin D were found to be greater in females than in males including inhibition of T cell proliferation, reduction in IFN-γ and IL-17, and an increase in Tregs and IL-10-secreting cells (295). Additionally, an independent study also showed that vitamin D promotes Treg differentiation and can ameliorate EAE in an estrogen-dependent manner (296).
Conclusion
The influence of sex chromosomes, modulated by direct and indirect effects of sex hormones, contributes to sex differences in lymphocyte populations and their functions. Although the contribution of these differences to specific autoimmune diseases remains to be fully understood, it is apparent that they contribute to the disparities in disease susceptibility, severity, and response to treatment between men and women. It is therefore important to acknowledge these differences in lymphocytes when evaluating treatment strategies, or disease biomarkers, as a step towards personalized medicine for individuals with autoimmune diseases. Future research aimed to further elaborate the sex bias in the immune response could provide novel tailored treatment strategies for improved management of autoimmune diseases.
Author contributions
KD and MM have been involved in the drafting of the manuscript and approved the final version.
Funding
MM is funded by the Academy of Medical Sciences Springboard Award (SBF006\1165), Asthma & Lung UK (RP22F\6) and University of Manchester Presidential Fellowship. KD is undertaking a PhD funded by the Manchester Myasthenia Gravis Research Fund (NorthCare Charity Fund CS2041), with additional grants from Myaware and the Neuromuscular Study Group, and a salary from the Northern Care Alliance NHS Foundation Trust.
Acknowledgments
We thank P. A. Blair and B. Potts for their comments on the manuscript.
Conflict of interest
The authors declare that the research was conducted in the absence of any commercial or financial relationships that could be construed as a potential conflict of interest.
Publisher’s note
All claims expressed in this article are solely those of the authors and do not necessarily represent those of their affiliated organizations, or those of the publisher, the editors and the reviewers. Any product that may be evaluated in this article, or claim that may be made by its manufacturer, is not guaranteed or endorsed by the publisher.
References
1. Connect Immune Research. Are you autimmune aware? report for parlimentarians into autoimmune conditions (2018). Available at: https://www.immunology.org/sites/default/files/connect-immune-research-are-you-autoimmune-report.pdf.
2. Manji N, Carr-Smith JD, Boelaert K, Allahabadia A, Armitage M, Chatterjee VK, et al. Influences of age, gender, smoking, and family history on autoimmune thyroid disease phenotype. J Clin Endocrinol Metab (2006) 91:4873–80. doi: 10.1210/jc.2006-1402
3. Tingi E, Syed AA, Kyriacou A, Mastorakos G, Kyriacou A. Benign thyroid disease in pregnancy: A state of the art review. J Clin Transl Endocrinol (2016) 6:37–49. doi: 10.1016/j.jcte.2016.11.001
4. Hughes GC, Choubey D. Modulation of autoimmune rheumatic diseases by oestrogen and progesterone. Nat Rev Rheumatol (2014) 10:740–51. doi: 10.1038/nrrheum.2014.144
5. Rindfleisch JA, Muller D. Diagnosis and management of rheumatoid arthritis. Am Fam Phys (2005) 72:1037–47.
6. Da Silva JA, Hall GM. The effects of gender and sex hormones on outcome in rheumatoid arthritis. Baillieres Clin Rheumatol (1992) 6:196–219. doi: 10.1016/S0950-3579(05)80344-9
7. Fernanda Romo-García M, Zapata-Zuñiga M, Antonio Enciso-Moreno J, Enrique Castañeda-Delgado J. The role of estrogens in rheumatoid arthritis physiopathology. In: Rheumatoid arthritis - other perspectives towards a better practice. (London: IntechOpen) (2020).
8. Magyari M. Gender differences in multiple sclerosis epidemiol-ogy and treatment response. Mult Scler (2014) 20:1244–51. doi: 10.1177/1352458514521515
9. Goodin DS. Chapter 11 - the epidemiology of multiple sclerosis: insights to disease pathogenesis. In: Goodin DS, editor. Handbook of clinical neurology. (London: Elsevier) (2014). p. 231–66.
10. . Varytė G, Zakarevičienė J, Ramašauskaitė D, Laužikienė D, Arlauskienė A. Pregnancy and multiple sclerosis: An update on the disease modifying treatment strategy and a review of pregnancy’s impact on disease activity. Medicina (2020) 56(2):49. doi: 10.3390/medicina56020049
11. Greuter T, Manser C, Pittet V, Vavricka SR, Biedermann L. On behalf of Swiss IBDnet, an official working group of the Swiss society of gastroenterology. gender differences in inflammatory bowel disease. Digestion (2020) 101 Suppl 1:98–104. doi: 10.1159/000504701
12. Restellini S, Biedermann L, Hruz P, Mottet C, Moens A, Ferrante M, et al. On behalf of Swiss IBDnet, an official working group of the Swiss society of gastroenterology. update on the management of inflammatory bowel disease during pregnancy and breastfeeding. Digestion (2020) 101 Suppl 1:27–42. doi: 10.1159/000502886
13. Molinié F, Gower-Rousseau C, Yzet T, Merle V, Grandbastien B, Marti R, et al. Opposite evolution in incidence of crohn’s disease and ulcerative colitis in northern France (1988-1999). Gut (2004) 53:843–8. doi: 10.1136/gut.2003.025346
14. Sardu C, Cocco E, Mereu A, Massa R, Cuccu A, Marrosu MG, et al. Population based study of 12 autoimmune diseases in Sardinia, Italy: prevalence and comorbidity. PloS One (2012) 7:e32487. doi: 10.1371/journal.pone.0032487
15. Jansson-Knodell CL, King KS, Larson JJ, Van Dyke CT, Murray JA, Rubio-Tapia A. Gender-based differences in a population-based cohort with celiac disease: More alike than unalike. Dig Dis Sci (2018) 63:184–92. doi: 10.1007/s10620-017-4835-0
16. Wasef SZY, Yacoub Wasef SZ. Gender differences in systemic lupus erythematosus. Gender Med (2004) 1:12–7. doi: 10.1016/s1550-8579(04)80006-8
17. Smyth A, Oliveira GHM, Lahr BD, Bailey KR, Norby SM, Garovic VD. A systematic review and meta-analysis of pregnancy outcomes in patients with systemic lupus erythematosus and lupus nephritis. Clin J Am Soc Nephrol (2010) 5:2060–8. doi: 10.2215/CJN.00240110
18. Dao KH, Bermas BL. Systemic lupus erythematosus management in pregnancy. Int J Womens Health (2022) 14:199–211. doi: 10.2147/IJWH.S282604
19. Dresser L, Wlodarski R, Rezania K, Soliven B. Myasthenia gravis: Epidemiology, pathophysiology and clinical manifestations. J Clin Med Res (2021) 10(11):2235. doi: 10.3390/jcm10112235
20. Asmail A, Kesler A, Kolb H, Drory VE, Karni A. A tri-modal distribution of age-of-onset in female patients with myasthenia gravis is associated with the gender-related clinical differences. Int J Neurosci (2019) 129:313–9. doi: 10.1080/00207454.2018.1529669
21. Queiro R, Tejón P, Coto P, Alonso S, Alperi M, Sarasqueta C, et al. Clinical differences between men and women with psoriatic arthritis: relevance of the analysis of genes and polymorphisms in the major histocompatibility complex region and of the age at onset of psoriasis. Clin Dev Immunol (2013) 2013:482691. doi: 10.1155/2013/482691
22. Queiro R, Tejón P, Alonso S, Coto P. Age at disease onset: a key factor for understanding psoriatic disease. Rheumatology (2014) 53:1178–85. doi: 10.1093/rheumatology/ket363
23. Hägg D, Eriksson M, Sundström A, Schmitt-Egenolf M. The higher proportion of men with psoriasis treated with biologics may be explained by more severe disease in men. PloS One (2013) 8:e63619. doi: 10.1371/journal.pone.0063619
24. Alazwari A, Abdollahian M, Tafakori L, Johnstone A, Alshumrani RA, Alhelal MT, et al. Predicting age at onset of type 1 diabetes in children using regression, artificial neural network and random forest: A case study in Saudi Arabia. PloS One (2022) 17(2):e0264118. doi: 10.1371/journal.pone.0264118
25. Manicardi V, Russo G, Napoli A, Torlone E, Li Volsi P, Giorda CB, et al. Gender-disparities in adults with type 1 diabetes: More than a quality of care issue. a cross-sectional observational study from the AMD annals initiative. PloS One (2016) 11:e0162960. doi: 10.1371/journal.pone.0162960
26. Rusman T, van Vollenhoven RF, van der Horst-Bruinsma IE. Gender differences in axial spondyloarthritis: Women are not so lucky. Curr Rheumatol Rep (2018) 20:35. doi: 10.1007/s11926-018-0744-2
27. Ortolan A, van Lunteren M, Ramiro S, Ramonda R, Landewé RBM, Dagfinrud H, et al. Are gender-specific approaches needed in diagnosing early axial spondyloarthritis? data from the SPondyloArthritis caught early cohort. Arthritis Res Ther (2018) 20:218. doi: 10.1186/s13075-018-1705-x
28. Mokbel A, Lawson DO, Farrokhyar F. Pregnancy outcomes in women with ankylosing spondylitis: a scoping literature and methodological review. Clin Rheumatol (2021) 40:3465–80. doi: 10.1007/s10067-021-05588-9
29. Shotan A, Keren A. Myocarditis and pregnancy. Cardiac problems in pregnancy. (2019) (New Jersey: Wiley Online Books), 107–15. doi: 10.1002/9781119409861
30. Kytö V, Sipilä J, Rautava P. The effects of gender and age on occurrence of clinically suspected myocarditis in adulthood. Heart (2013) 99:1681–4. doi: 10.1136/heartjnl-2013-304449
31. Cooper LT, Mather PJ, Alexis JD, Pauly DF, Torre-Amione G, Wittstein IS, et al. Myocardial recovery in peripartum cardiomyopathy: prospective comparison with recent onset cardiomyopathy in men and nonperipartum women. J Card Fail (2012) 18:28–33. doi: 10.1016/j.cardfail.2011.09.009
32. Klein SL. Sex influences immune responses to viruses, and efficacy of prophylaxis and treatments for viral diseases. Bioessays (2012) 34:1050–9. doi: 10.1002/bies.201200099
33. Kronzer VL, Bridges SL Jr, Davis JM 3rd. Why women have more autoimmune diseases than men: An evolutionary perspective. Evol Appl (2021) 14:629–33. doi: 10.1111/eva.13167
34. Klein SL, Morgan R. The impact of sex and gender on immunotherapy outcomes. Biol Sex Dif (2020) 11. doi: 10.1186/s13293-020-00301-y
35. Beery AK, Zucker I. Sex bias in neuroscience and biomedical research. Neurosci Biobehav Rev (2011) 35:565–72. doi: 10.1016/j.neubiorev.2010.07.002
36. Mackay IR. Topics in review: Tolerance and autoimmunity. West J Med (2001) 174:118. doi: 10.1136/ewjm.174.2.118
37. Fan H, Dong G, Zhao G, Liu F, Yao G, Zhu Y, et al. Gender differences of b cell signature in healthy subjects underlie disparities in incidence and course of SLE related to estrogen. J Immunol Res (2014) 2014:814598. doi: 10.1155/2014/814598
38. Libert C, Dejager L, Pinheiro I. The X chromosome in immune functions: when a chromosome makes the difference. Nat Rev Immunol (2010) 10:594–604. doi: 10.1038/nri2815
39. Klein SL, Flanagan KL. Sex differences in immune responses. Nat Rev Immunol (2016) 16:626–38. doi: 10.1038/nri.2016.90
40. Berletch JB, Yang F, Xu J, Carrel L, Disteche CM. Genes that escape from X inactivation. Hum Genet (2011) 130:237–45. doi: 10.1007/s00439-011-1011-z
41. Carrel L, Willard HF. X-Inactivation profile reveals extensive variability in X-linked gene expression in females. Nature (2005) 434:400–4. doi: 10.1038/nature03479
42. Chabchoub G, Uz E, Maalej A, Mustafa CA, Rebai A, Mnif M, et al. Analysis of skewed X-chromosome inactivation in females with rheumatoid arthritis and autoimmune thyroid diseases. Arthritis Res Ther (2009) 11:R106 doi: 10.1186/ar2759.
43. Wang J, Syrett CM, Kramer MC, Basu A, Atchison ML, Anguera MC. Unusual maintenance of X chromosome inactivation predisposes female lymphocytes for increased expression from the inactive X. Proc Natl Acad Sci U.S.A. (2016) 113:E2029–38. doi: 10.1073/pnas.1520113113
44. Kanaan SB, Onat OE, Balandraud N, Martin GV, Nelson JL, Azzouz DF, et al. Evaluation of X chromosome inactivation with respect to HLA genetic susceptibility in rheumatoid arthritis and systemic sclerosis. PloS One (2016) 11:e0158550. doi: 10.1371/journal.pone.0158550
45. Pinheiro I, Dejager L, Libert C. X-Chromosome-located microRNAs in immunity: might they explain male/female differences? the X chromosome-genomic context may affect X-located miRNAs and downstream signaling, thereby contributing to the enhanced immune response of females. Bioessays (2011) 33:791–802. doi: 10.1002/bies.201100047
46. Taneja V. Sex hormones determine immune response. Front Immunol (2018) 9:1931. doi: 10.3389/fimmu.2018.01931
47. Jiwrajka N, Anguera MC. The X in seX-biased immunity and autoimmune rheumatic disease. J Exp Med (2022) 219(6):e20211487. doi: 10.1084/jem.20211487
48. Kovats S. Estrogen receptors regulate innate immune cells and signaling pathways. Cell Immunol (2015) 294:63–9. doi: 10.1016/j.cellimm.2015.01.018
49. Bereshchenko O, Bruscoli S, Riccardi C. Glucocorticoids, sex hormones, and immunity. Front Immunol (2018) 9:1332. doi: 10.3389/fimmu.2018.01332
50. Buskiewicz IA, Huber SA, Fairweather D. Chapter 4 - sex hormone receptor expression in the immune system. In: Neigh GN, Mitzelfelt MM, editors. Sex differences in physiology. Boston: Academic Press (2016). p. 45–60.
51. Brown MA, Su MA. An inconvenient variable: Sex hormones and their impact on T cell responses. J Immunol (2019) 202:1927–33. doi: 10.4049/jimmunol.1801403
52. Lee BW, Yap HK, Chew FT, Quah TC, Prabhakaran K, Chan GS, et al. Age- and sex-related changes in lymphocyte subpopulations of healthy Asian subjects: from birth to adulthood. Cytometry (1996) 26:8–15. doi: 10.1002/(SICI)1097-0320(19960315)26:1<8::AID-CYTO2>3.0.CO;2-E
53. Uppal SS, Verma S, Dhot PS. Normal values of CD4 and CD8 lymphocyte subsets in healthy indian adults and the effects of sex, age, ethnicity, and smoking. Cytomet B Clin Cytom (2003) 52:32–6. doi: 10.1002/cyto.b.10011
54. Wikby A, Månsson IA, Johansson B, Strindhall J, Nilsson SE. The immune risk profile is associated with age and gender: findings from three Swedish population studies of individuals 20-100 years of age. Biogerontology (2008) 9:299–308. doi: 10.1007/s10522-008-9138-6
55. Abdullah M, Chai P-S, Chong M-Y, Tohit ERM, Ramasamy R, Pei CP, et al. Gender effect on in vitro lymphocyte subset levels of healthy individuals. Cell Immunol (2012) 272:214–9. doi: 10.1016/j.cellimm.2011.10.009
56. Lai J-J, Lai K-P, Zeng W, Chuang K-H, Altuwaijri S, Chang C. Androgen receptor influences on body defense system via modulation of innate and adaptive immune systems: lessons from conditional AR knockout mice. Am J Pathol (2012) 181:1504–12. doi: 10.1016/j.ajpath.2012.07.008
57. Roden AC, Moser MT, Tri SD, Mercader M, Kuntz SM, Dong H, et al. Augmentation of T cell levels and responses induced by androgen deprivation. J Immunol (2004) 173:6098–108. doi: 10.4049/jimmunol.173.10.6098
58. Olsen NJ, Kovacs WJ. Effects of androgens on T and b lymphocyte development. Immunol Res (2001) 23:281–8. doi: 10.1385/IR:23:2-3:281
59. Lee S, Kim J, Jang B, Hur S, Jung U, Kil K, et al. Fluctuation of peripheral blood T, b, and NK cells during a menstrual cycle of normal healthy women. J Immunol (2010) 185:756–62. doi: 10.4049/jimmunol.0904192
60. Maret A, Coudert JD, Garidou L, Foucras G, Gourdy P, Krust A, et al. Estradiol enhances primary antigen-specific CD4 T cell responses and Th1 development in vivo. essential role of estrogen receptor alpha expression in hematopoietic cells. Eur J Immunol (2003) 33:512–21. doi: 10.1002/immu.200310027
61. Chatzileontiadou DSM, Sloane H, Nguyen AT, Gras S, Grant EJ. The many faces of CD4+ T cells: Immunological and structural characteristics. Int J Mol Sci (2020) 22(1):73. doi: 10.3390/ijms22010073
62. Sankaran-Walters S, Macal M, Grishina I, Nagy L, Goulart L, Coolidge K, et al. Sex differences matter in the gut: effect on mucosal immune activation and inflammation. Biol Sex Differ (2013) 4:10. doi: 10.1186/2042-6410-4-10
63. Li J, McMurray RW. Effects of estrogen receptor subtype-selective agonists on immune functions in ovariectomized mice. Int Immunopharmacol (2006) 6:1413–23. doi: 10.1016/j.intimp.2006.04.019
64. Ruddy SC, Lau R, Cabrita MA, McGregor C, McKay BC, Murphy LC, et al. Preferential estrogen receptor β ligands reduce bcl-2 expression in hormone-resistant breast cancer cells to increase autophagy. Mol Cancer Ther (2014) 13:1882–93. doi: 10.1158/1535-7163.MCT-13-1066
65. Rosenzweig R, Gupta S, Kumar V, Gumina RJ, Bansal SS. Estrogenic bias in T-lymphocyte biology: Implications for cardiovascular disease. Pharmacol Res (2021) 170:105606. doi: 10.1016/j.phrs.2021.105606
66. Nancy P, Berrih-Aknin S. Differential estrogen receptor expression in autoimmune myasthenia gravis. Endocrinology (2005) 146:2345–53. doi: 10.1210/en.2004-1003
67. Fox HS, Bond BL, Parslow TG. Estrogen regulates the IFN-gamma promoter. J Immunol (1991) 146:4362–7.
68. Shen H, Panchanathan R, Rajavelu P, Duan X, Gould KA, Choubey D. Gender-dependent expression of murine Irf5 gene: implications for sex bias in autoimmunity. J Mol Cell Biol (2010) 2:284–90. doi: 10.1093/jmcb/mjq023
69. Eames HL, Corbin AL, Udalova IA. Interferon regulatory factor 5 in human autoimmunity and murine models of autoimmune disease. Transl Res (2016) 167:167–82. doi: 10.1016/j.trsl.2015.06.018
70. Panchanathan R, Liu H, Choubey D. Expression of murine Unc93b1 is up-regulated by interferon and estrogen signaling: implications for sex bias in the development of autoimmunity. Int Immunol (2013) 25:521–9. doi: 10.1093/intimm/dxt015
71. Karpuzoglu-Sahin E, Hissong BD, Ansar Ahmed S. Interferon-gamma levels are upregulated by 17-beta-estradiol and diethylstilbestrol. J Reprod Immunol (2001) 52:113–27. doi: 10.1016/s0165-0378(01)00117-6
72. Karpuzoglu E, Phillips RA, Gogal RM Jr, Ansar Ahmed S. IFN-gamma-inducing transcription factor, T-bet is upregulated by estrogen in murine splenocytes: role of IL-27 but not IL-12. Mol Immunol (2007) 44:1808–14. doi: 10.1016/j.molimm.2006.08.005
73. Navarro FC, Watkins SK. Estrogen stimulation differentially impacts human male and female antigen-specific T cell anti-tumor function and polyfunctionality. Gend Genome (2017) 1:1–13. doi: 10.1089/gg.2017.0014
74. Faas M, Bouman A, Moesa H, Heineman MJ, de Leij L, Schuiling G. The immune response during the luteal phase of the ovarian cycle: a Th2-type response? Fertil Steril (2000) 74:1008–13. doi: 10.1016/s0015-0282(00)01553-3
75. Agarwal SK, Marshall GD Jr. Perimenstrual alterations in type-1/type-2 cytokine balance of normal women. Ann Allergy Asthma Immunol (1999) 83:222–8. doi: 10.1016/S1081-1206(10)62644-0
76. Lambert KC, Curran EM, Judy BM, Milligan GN, Lubahn DB, Estes DM. Estrogen receptor alpha (ERalpha) deficiency in macrophages results in increased stimulation of CD4+ T cells while 17beta-estradiol acts through ERalpha to increase IL-4 and GATA-3 expression in CD4+ T cells independent of antigen presentation. J Immunol (2005) 175:5716–23. doi: 10.4049/jimmunol.175.9.5716
77. Matalka KZ. The effect of estradiol, but not progesterone, on the production of cytokines in stimulated whole blood, is concentration-dependent. Neuro Endocrinol Lett (2003) 24:185–91.
78. Moulton VR, Holcomb DR, Zajdel MC, Tsokos GC. Estrogen upregulates cyclic AMP response element modulator α expression and downregulates interleukin-2 production by human T lymphocytes. Mol Med (2012) 18:370–8. doi: 10.2119/molmed.2011.00506
79. Piccinni MP, Giudizi MG, Biagiotti R, Beloni L, Giannarini L, Sampognaro S, et al. Progesterone favors the development of human T helper cells producing Th2-type cytokines and promotes both IL-4 production and membrane CD30 expression in established Th1 cell clones. J Immunol (1995) 155:128–33.
80. Sabahi F, Rola-Plesczcynski M, O’Connell S, Frenkel LD. Qualitative and quantitative analysis of T lymphocytes during normal human pregnancy. Am J Reprod Immunol (1995) 33:381–93. doi: 10.1111/j.1600-0897.1995.tb00907.x
81. Dosiou C, Hamilton AE, Pang Y, Overgaard MT, Tulac S, Dong J, et al. Expression of membrane progesterone receptors on human T lymphocytes and jurkat cells and activation of G-proteins by progesterone. J Endocrinol (2008) 196:67–77. doi: 10.1677/JOE-07-0317
82. Girón-González JA, Moral FJ, Elvira J, García-Gil D, Guerrero F, Gavilán I, et al. Consistent production of a higher TH1:TH2 cytokine ratio by stimulated T cells in men compared with women. Eur J Endocrinol (2000) 143:31–6. doi: 10.1530/eje.0.1430031
83. Zhang MA, Rego D, Moshkova M, Kebir H, Chruscinski A, Nguyen H, et al. Peroxisome proliferator-activated receptor (PPAR) α and-γ regulate IFNγ and IL-17A production by human T cells in a sex-specific way. Proc Natl Acad Sci (2012) 109:9505–10. doi: 10.1073/pnas.1118458109
84. Bouman A, Schipper M, Heineman MJ, Faas MM. Gender difference in the non-specific and specific immune response in humans. Am J Reprod Immunol (2004) 52:19–26. doi: 10.1111/j.1600-0897.2004.00177.x
85. Huber SA, Pfaeffle B. Differential Th1 and Th2 cell responses in male and female BALB/c mice infected with coxsackievirus group b type 3. J Virol (1994) 68:5126–32. doi: 10.1128/jvi.68.8.5126-5132.1994
86. Dalal M, Kim S, Voskuhl RR. Testosterone therapy ameliorates experimental autoimmune encephalomyelitis and induces a T helper 2 bias in the autoantigen-specific T lymphocyte response. J Immunol (1997) 159:3–6.
87. Bebo BF Jr, Schuster JC, Vandenbark AA, Offner H. Androgens alter the cytokine profile and reduce encephalitogenicity of myelin-reactive T cells. J Immunol (1999) 162:35–40.
88. Liva SM, Voskuhl RR. Testosterone acts directly on CD4+ T lymphocytes to increase IL-10 production. J Immunol (2001) 167:2060–7. doi: 10.4049/jimmunol.167.4.2060
89. Namazi MR. The Th1-promoting effects of dehydroepiandrosterone can provide an explanation for the stronger Th1-immune response of women. Iran J Allergy Asthma Immunol (2009) 8:65–9.
90. Dunn SE, Ousman SS, Sobel RA, Zuniga L, Baranzini SE, Youssef S, et al. Peroxisome proliferator-activated receptor (PPAR)alpha expression in T cells mediates gender differences in development of T cell-mediated autoimmunity. J Exp Med (2007) 204:321–30. doi: 10.1084/jem.20061839
91. Kissick HT, Sanda MG, Dunn LK, Pellegrini KL, On ST, Noel JK, et al. Androgens alter T-cell immunity by inhibiting T-helper 1 differentiation. Proc Natl Acad Sci U.S.A. (2014) 111:9887–92. doi: 10.1073/pnas.1402468111
92. Massa MG, David C, Jörg S, Berg J, Gisevius B, Hirschberg S, et al. Testosterone differentially affects T cells and neurons in murine and human models of neuroinflammation and neurodegeneration. Am J Pathol (2017) 187:1613–22. doi: 10.1016/j.ajpath.2017.03.006
93. Lang TJ. Estrogen as an immunomodulator. Clin Immunol (2004) 113:224–30. doi: 10.1016/j.clim.2004.05.011
94. Hepworth MR, Hardman MJ, Grencis RK. The role of sex hormones in the development of Th2 immunity in a gender-biased model of trichuris muris infection. Eur J Immunol (2010) 40:406–16. doi: 10.1002/eji.200939589
95. Araneo BA, Dowell T, Diegel M, Daynes RA. Dihydrotestosterone exerts a depressive influence on the production of interleukin-4 (IL-4), IL-5, and gamma-interferon, but not IL-2 by activated murine T cells. Blood (1991) 78:688–99. doi: 10.1182/blood.V78.3.688.688
96. Freeman BM, Mountain DJH, Brock TC, Chapman JR, Kirkpatrick SS, Freeman MB, et al. Low testosterone elevates interleukin family cytokines in a rodent model: a possible mechanism for the potentiation of vascular disease in androgen-deficient males. J Surg Res (2014) 190:319–27. doi: 10.1016/j.jss.2014.03.017
97. Mohamad N-V, Wong SK, Wan Hasan WN, Jolly JJ, Nur-Farhana MF, Ima-Nirwana S, et al. The relationship between circulating testosterone and inflammatory cytokines in men. Aging Male (2019) 22:129–40. doi: 10.1080/13685538.2018.1482487
98. Ben-Batalla I, Vargas-Delgado ME, von Amsberg G, Janning M, Loges S. Influence of androgens on immunity to self and foreign: Effects on immunity and cancer. Front Immunol (2020) 11:1184. doi: 10.3389/fimmu.2020.01184
99. Malkin CJ, Pugh PJ, Jones RD, Kapoor D, Channer KS, Jones TH. The effect of testosterone replacement on endogenous inflammatory cytokines and lipid profiles in hypogonadal men. J Clin Endocrinol Metab (2004) 89:3313–8. doi: 10.1210/jc.2003-031069
100. Blanco LP, Plegue M, Fung-Leung W-P, Holoshitz J. Gender-biased regulation of human IL-17-producing cells in vitro by peptides corresponding to distinct HLA-DRB1 allele-coded sequences. J Immune Based Ther Vaccines Antimicrob (2013) 2:29–38. doi: 10.4236/jibtva.2013.23004
101. Mohammad I, Starskaia I, Nagy T, Guo J, Yatkin E, Väänänen K, et al. Estrogen receptor α contributes to T cell–mediated autoimmune inflammation by promoting T cell activation and proliferation. Sci Signal (2018) 11(526):eaap941. doi: 10.1126/scisignal.aap9415
102. Fuseini H, Cephus J-Y, Wu P, Davis JB, Contreras DC, Gandhi VD, et al. ERα signaling increased IL-17A production in Th17 cells by upregulating IL-23R expression, mitochondrial respiration, and proliferation. Front Immunol (2019) 10:2740. doi: 10.3389/fimmu.2019.02740
103. Bebo BF Jr, Fyfe-Johnson A, Adlard K, Beam AG, Vandenbark AA, Offner H. Low-dose estrogen therapy ameliorates experimental autoimmune encephalomyelitis in two different inbred mouse strains. J Immunol (2001) 166:2080–9. doi: 10.4049/jimmunol.166.3.2080
104. Jansson L, Olsson T, Holmdahl R. Estrogen induces a potent suppression of experimental autoimmune encephalomyelitis and collagen-induced arthritis in mice. J Neuroimmunol (1994) 53:203–7. doi: 10.1016/0165-5728(94)90030-2
105. Afshan G, Afzal N, Qureshi S. CD4+CD25(hi) regulatory T cells in healthy males and females mediate gender difference in the prevalence of autoimmune diseases. Clin Lab (2012) 58:567–71.
106. Voskuhl RR, Sawalha AH, Itoh Y. Sex chromosome contributions to sex differences in multiple sclerosis susceptibility and progression. Mult Scler (2018) 24:22–31. doi: 10.1177/1352458517737394
107. Robinson GA, Peng J, Peckham H, Butler G, Pineda-Torra I, Ciurtin C, et al. Investigating sex differences in T regulatory cells from cisgender and transgender healthy individuals and patients with autoimmune inflammatory disease: a cross-sectional study. Lancet Rheumatol (2022) 4:e710–24. doi: 10.1016/S2665-9913(22)00198-9
108. Zhu M-L, Bakhru P, Conley B, Nelson JS, Free M, Martin A, et al. Sex bias in CNS autoimmune disease mediated by androgen control of autoimmune regulator. Nat Commun (2016) 7:11350. doi: 10.1038/ncomms11350
109. Walecki M, Eisel F, Klug J, Baal N, Paradowska-Dogan A, Wahle E, et al. Androgen receptor modulates Foxp3 expression in CD4+CD25+Foxp3+ regulatory T-cells. Mol Biol Cell (2015) 26:2845–57. doi: 10.1091/mbc.E14-08-1323
110. Arruvito L, Sanz M, Banham AH, Fainboim L. Expansion of CD4+ CD25+ and FOXP3+ regulatory T cells during the follicular phase of the menstrual cycle: implications for human reproduction. J Immunol (2007) 178:2572–8. doi: 10.4049/jimmunol.178.4.2572
111. Mao G, Wang J, Kang Y, Tai P, Wen J, Zou Q, et al. Progesterone increases systemic and local uterine proportions of CD4+CD25+ treg cells during midterm pregnancy in mice. Endocrinology (2010) 151:5477–88. doi: 10.1210/en.2010-0426
112. Lee JH, Lydon JP, Kim CH. Progesterone suppresses the mTOR pathway and promotes generation of induced regulatory T cells with increased stability. Eur J Immunol (2012) 42:2683–96. doi: 10.1002/eji.201142317
113. Huang N, Chi H, Qiao J. Role of regulatory T cells in regulating fetal-maternal immune tolerance in healthy pregnancies and reproductive diseases. Front Immunol (2020) 11:1023. doi: 10.3389/fimmu.2020.01023
114. Polanczyk MJ, Carson BD, Subramanian S, Afentoulis M, Vandenbark AA, Ziegler SF, et al. Cutting edge: Estrogen drives expansion of the CD4 CD25 regulatory T cell compartment. J Immunol (2004) 173:2227–30. doi: 10.4049/jimmunol.173.4.2227
115. Prieto GA, Rosenstein Y. Oestradiol potentiates the suppressive function of human CD4 CD25 regulatory T cells by promoting their proliferation. Immunology (2006) 118:58–65. doi: 10.1111/j.1365-2567.2006.02339.x
116. Rowe JH, Ertelt JM, Xin L, Way SS. Pregnancy imprints regulatory memory that sustains anergy to fetal antigen. Nature (2012) 490:102–6. doi: 10.1038/nature11462
117. Wang C, Dehghani B, Li Y, Kaler LJ, Vandenbark AA, Offner H. Oestrogen modulates experimental autoimmune encephalomyelitis and interleukin-17 production via programmed death 1. Immunology (2009) 126:329–35. doi: 10.1111/j.1365-2567.2008.03051.x
118. Bynoe MS, Grimaldi CM, Diamond B. Estrogen up-regulates bcl-2 and blocks tolerance induction of naive b cells. Proc Natl Acad Sci U.S.A. (2000) 97:2703–8. doi: 10.1073/pnas.040577497
119. Asaba J, Bandyopadhyay M, Kindy M, Dasgupta S. Estrogen receptor signal in regulation of b cell activation during diverse immune responses. Int J Biochem Cell Biol (2015) 68:42–7. doi: 10.1016/j.biocel.2015.08.012
120. Straub RH. The complex role of estrogens in inflammation. Endocr Rev (2007) 28:521–74. doi: 10.1210/er.2007-0001
121. Herblot S, Aplan PD, Hoang T. Gradient of E2A activity in b-cell development. Mol Cell Biol (2002) 22:886–900. doi: 10.1128/MCB.22.3.886-900.2002
122. Sakiani S, Olsen NJ, Kovacs WJ. Gonadal steroids and humoral immunity. Nat Rev Endocrinol (2013) 9:56–62. doi: 10.1038/nrendo.2012.206
123. Verthelyi D. Sex hormones as immunomodulators in health and disease. Int Immunopharmacol (2001) 1:983–93. doi: 10.1016/S1567-5769(01)00044-3
124. Grimaldi CM, Michael DJ, Diamond B. Cutting edge: expansion and activation of a population of autoreactive marginal zone b cells in a model of estrogen-induced lupus. J Immunol (2001) 167:1886–90. doi: 10.4049/jimmunol.167.4.1886
125. Grimaldi CM, Jeganathan V, Diamond B. Hormonal regulation of b cell development: 17 beta-estradiol impairs negative selection of high-affinity DNA-reactive b cells at more than one developmental checkpoint. J Immunol (2006) 176:2703–10. doi: 10.4049/jimmunol.176.5.2703
126. Hill L, Jeganathan V, Chinnasamy P, Grimaldi C, Diamond B. Differential roles of estrogen receptors α and β in control of b-cell maturation and selection. Mol Med (2011) 17:211–20. doi: 10.2119/molmed.2010.00172
127. Phiel KL, Henderson RA, Adelman SJ, Elloso MM. Differential estrogen receptor gene expression in human peripheral blood mononuclear cell populations. Immunol Lett (2005) 97:107–13. doi: 10.1016/j.imlet.2004.10.007
128. Panchanathan R, Shen H, Zhang X, Ho S-M, Choubey D. Mutually positive regulatory feedback loop between interferons and estrogen receptor-alpha in mice: implications for sex bias in autoimmunity. PloS One (2010) 5:e10868. doi: 10.1371/journal.pone.0010868
129. Wilhelmson AS, Lantero Rodriguez M, Stubelius A, Fogelstrand P, Johansson I, Buechler MB, et al. Testosterone is an endogenous regulator of BAFF and splenic b cell number. Nat Commun (2018) 9:2067. doi: 10.1038/s41467-018-04408-0
130. Altuwaijri S, Chuang K-H, Lai K-P, Lai J-J, Lin H-Y, Young FM, et al. Susceptibility to autoimmunity and b cell resistance to apoptosis in mice lacking androgen receptor in b cells. Mol Endocrinol (2009) 23:444–53. doi: 10.1210/me.2008-0106
131. Grimaldi CM, Cleary J, Dagtas AS, Moussai D, Diamond B. Estrogen alters thresholds for b cell apoptosis and activation. J Clin Invest (2002) 109:1625–33. doi: 10.1172/JCI0214873
132. Panchanathan R, Choubey D. Murine BAFF expression is up-regulated by estrogen and interferons: implications for sex bias in the development of autoimmunity. Mol Immunol (2013) 53:15–23. doi: 10.1016/j.molimm.2012.06.013
133. Grimaldi CM, Hill L, Xu X, Peeva E, Diamond B. Hormonal modulation of b cell development and repertoire selection. Mol Immunol (2005) 42:811–20. doi: 10.1016/j.molimm.2004.05.014
134. Luckey D, Medina K, Taneja V. B cells as effectors and regulators of sex-biased arthritis. Autoimmunity (2012) 45:364–76. doi: 10.3109/08916934.2012.665528
135. Furman D, Hejblum BP, Simon N, Jojic V, Dekker CL, Thiébaut R, et al. Systems analysis of sex differences reveals an immunosuppressive role for testosterone in the response to influenza vaccination. Proc Natl Acad Sci U.S.A. (2014) 111:869–74. doi: 10.1073/pnas.1321060111
136. Giltay EJ, Fonk JC, von Blomberg BM, Drexhage HA, Schalkwijk C, Gooren LJ. In vivo effects of sex steroids on lymphocyte responsiveness and immunoglobulin levels in humans. J Clin Endocrinol Metab (2000) 85:1648–57. doi: 10.1210/jcem.85.4.6562
137. Eidinger D, Garrett TJ. Studies of the regulatory effects of the sex hormones on antibody formation and stem cell differentiation. J Exp Med (1972) 136:1098–116. doi: 10.1084/jem.136.5.1098
138. Jones BG, Sealy RE, Penkert RR, Surman SL, Maul RW, Neale G, et al. Complex sex-biased antibody responses: estrogen receptors bind estrogen response elements centered within immunoglobulin heavy chain gene enhancers. Int Immunol (2019) 31:141–56. doi: 10.1093/intimm/dxy074
139. Rhodes K, Markham RL, Maxwell PM, Monk-Jones ME. Immunoglobulins and the X-chromosome. Br Med J (1969) 3:439–41. doi: 10.1136/bmj.3.5668.439
140. Souyris M, Cenac C, Azar P, Daviaud D, Canivet A, Grunenwald S, et al. TLR7escapes X chromosome inactivation in immune cells. Sci Immunol (2018) 3:eaap8855. doi: 10.1126/sciimmunol.aap8855
141. Souyris M, Mejía JE, Chaumeil J, Guéry J-C. Female predisposition to TLR7-driven autoimmunity: gene dosage and the escape from X chromosome inactivation. Semin Immunopathol (2019) 41:153–64. doi: 10.1007/s00281-018-0712-y
142. Lü FX, Abel K, Ma Z, Rourke T, Lu D, Torten J, et al. The strength of b cell immunity in female rhesus macaques is controlled by CD8+ T cells under the influence of ovarian steroid hormones. Clin Exp Immunol (2002) 128:10–20. doi: 10.1046/j.1365-2249.2002.01780.x
143. Sthoeger ZM, Chiorazzi N, Lahita RG. Regulation of the immune response by sex hormones. i. In vitro effects of estradiol and testosterone on pokeweed mitogen-induced human b cell differentiation. J Immunol (1988) 141:91–8.
144. Yates MA, Li Y, Chlebeck P, Proctor T, Vandenbark AA, Offner H. Progesterone treatment reduces disease severity and increases IL-10 in experimental autoimmune encephalomyelitis. J Neuroimmunol (2010) 220:136–9. doi: 10.1016/j.jneuroim.2010.01.013
145. Park S-R, Zan H, Pal Z, Zhang J, Al-Qahtani A, Pone EJ, et al. HoxC4 binds to the promoter of the cytidine deaminase AID gene to induce AID expression, class-switch DNA recombination and somatic hypermutation. Nat Immunol (2009) 10:540–50. doi: 10.1038/ni.1725
146. Pauklin S, Petersen-Mahrt SK. Progesterone inhibits activation-induced deaminase by binding to the promoter. J Immunol (2009) 183:1238–44. doi: 10.4049/jimmunol.0803915
147. Cutolo M, Capellino S, Sulli A, Serioli B, Secchi ME, Villaggio B, et al. Estrogens and autoimmune diseases. Ann N Y Acad Sci (2006) 1089:538–47. doi: 10.1196/annals.1386.043
148. Singh RP, Bischoff DS. Sex hormones and gender influence the expression of markers of regulatory T cells in SLE patients. Front Immunol (2021) 12:619268. doi: 10.3389/fimmu.2021.619268
149. Tengstrand B, Carlström K, Felländer-Tsai L, Hafström I. Abnormal levels of serum dehydroepiandrosterone, estrone, and estradiol in men with rheumatoid arthritis: high correlation between serum estradiol and current degree of inflammation. J Rheumatol (2003) 30:2338–43.
150. Marx A, Ströbel P, Weis C-A. The pathology of the thymus in myasthenia gravis. Mediastinum (2018) 2:66–6. doi: 10.21037/med.2018.12.04
151. Dema B, Charles N. Autoantibodies in SLE: Specificities, isotypes and receptors. Antibodies (Basel) (2016) 5(1):2. doi: 10.3390/antib5010002
152. Rahman A, Isenberg DA. Systemic lupus erythematosus. N Engl J Med 358(9):929–39. doi: 10.1056/NEJMra071297
153. Hewagama A, Patel D, Yarlagadda S, Strickland FM, Richardson BC. Stronger inflammatory/cytotoxic T-cell response in women identified by microarray analysis. Genes Immun (2009) 10:509–16. doi: 10.1038/gene.2009.12
154. Hewagama A. Role of X-chromosome encoded miRNAs in autoimmunity: suppressing the suppressor and female predisposition. Rheumatol Curr Res (2013) 3:118. doi: 10.4172/2161-1149.1000118
155. Khan D, Dai R, Ansar Ahmed S. Sex differences and estrogen regulation of miRNAs in lupus, a prototypical autoimmune disease. Cell Immunol (2015) 294:70–9. doi: 10.1016/j.cellimm.2015.01.004
156. Lu M-C, Lai N-S, Chen H-C, Yu H-C, Huang K-Y, Tung C-H, et al. Decreased microRNA(miR)-145 and increased miR-224 expression in T cells from patients with systemic lupus erythematosus involved in lupus immunopathogenesis. Clin Exp Immunol (2012) 171:91–9. doi: 10.1111/j.1365-2249.2012.04676.x
157. Steinmetz OM, Turner J-E, Paust H-J, Lindner M, Peters A, Heiss K, et al. CXCR3 mediates renal Th1 and Th17 immune response in murine lupus nephritis. J Immunol (2009) 183:4693–704. doi: 10.4049/jimmunol.0802626
158. Enghard P, Humrich JY, Rudolph B, Rosenberger S, Biesen R, Kuhn A, et al. CXCR3+CD4+ T cells are enriched in inflamed kidneys and urine and provide a new biomarker for acute nephritis flares in systemic lupus erythematosus patients. Arthritis Rheum (2009) 60:199–206. doi: 10.1002/art.24136
159. Lu Q, Wu A, Tesmer L, Ray D, Yousif N, Richardson B. Demethylation of CD40LG on the inactive X in T cells from women with lupus. J Immunol (2007) 179:6352–8. doi: 10.4049/jimmunol.179.9.6352
160. Zhou Y, Yuan J, Pan Y, Fei Y, Qiu X, Hu N, et al. T Cell CD40LG gene expression and the production of IgG by autologous b cells in systemic lupus erythematosus. Clin Immunol (2009) 132:362–70. doi: 10.1016/j.clim.2009.05.011
161. Meier A, Chang JJ, Chan ES, Pollard RB, Sidhu HK, Kulkarni S, et al. Sex differences in the toll-like receptor-mediated response of plasmacytoid dendritic cells to HIV-1. Nat Med (2009) 15:955–9. doi: 10.1038/nm.2004
162. Griesbeck M, Ziegler S, Laffont S, Smith N, Chauveau L, Tomezsko P, et al. Sex differences in plasmacytoid dendritic cell levels of IRF5 drive higher IFN-α production in women. J Immunol (2015) 195:5327–36. doi: 10.4049/jimmunol.1501684
163. Berghöfer B, Frommer T, Haley G, Fink L, Bein G, Hackstein H. TLR7 ligands induce higher IFN-alpha production in females. J Immunol (2006) 177:2088–96. doi: 10.4049/jimmunol.177.4.2088
164. Rönnblom L, Eloranta M-L, Alm GV. The type I interferon system in systemic lupus erythematosus. Arthritis Rheum (2006) 54:408–20. doi: 10.1002/art.21571
165. Elkon KB, Stone VV. Type I interferon and systemic lupus erythematosus. J Interferon Cytokine Res (2011) 31:803–12. doi: 10.1089/jir.2011.0045
166. Nakashima H, Akahoshi M, Masutani K. Th1/Th2 balance of SLE patients with lupus nephritis. Rinsho Byori (2006) 54:706–13.
167. Shaukat F, Russinova L, Seizhanova B, Rakhmetova A. Lupus nephritis in males; updates to current knowledge. J Nephropharmacol (2019) 8:9–9. doi: 10.15171/npj.2019.09
168. Haddadi N-S, Mande P, Brodeur TY, Hao K, Ryan GE, Moses S, et al. Th2 to Th1 transition is required for induction of skin lesions in an inducible and recurrent murine model of cutaneous lupus-like inflammation. Front Immunol (2022) 13:883375. doi: 10.3389/fimmu.2022.883375
169. Ramírez Sepúlveda JI, Bolin K, Mofors J, Leonard D, Svenungsson E, Jönsen A, et al. Sex differences in clinical presentation of systemic lupus erythematosus. Biol Sex Differ (2019) 10:60. doi: 10.1186/s13293-019-0274-2
170. Feng F, Nyland J, Banyai M, Tatum A, Silverstone AE, Gavalchin J. The induction of the lupus phenotype by estrogen is via an estrogen receptor-alpha-dependent pathway. Clin Immunol (2010) 134:226–36. doi: 10.1016/j.clim.2009.10.004
171. Bynoté KK, Hackenberg JM, Korach KS, Lubahn DB, Lane PH, Gould KA. Estrogen receptor-alpha deficiency attenuates autoimmune disease in (NZB x NZW)F1 mice. Genes Immun (2008) 9:137–52. doi: 10.1038/sj.gene.6364458
172. Svenson JL, EuDaly J, Ruiz P, Korach KS, Gilkeson GS. Impact of estrogen receptor deficiency on disease expression in the NZM2410 lupus prone mouse. Clin Immunol (2008) 128:259–68. doi: 10.1016/j.clim.2008.03.508
173. Young NA, Wu L-C, Burd CJ, Friedman AK, Kaffenberger BH, Rajaram MVS, et al. Estrogen modulation of endosome-associated toll-like receptor 8: an IFNα-independent mechanism of sex-bias in systemic lupus erythematosus. Clin Immunol (2014) 151:66–77. doi: 10.1016/j.clim.2014.01.006
174. Li J, McMurray RW. Effects of estrogen receptor subtype-selective agonists on autoimmune disease in lupus-prone NZB/NZW F1 mouse model. Clin Immunol (2007) 123:219–26. doi: 10.1016/j.clim.2007.01.008
175. Lin H-L, Yen J-H, Chiou S-S, Tsai W-C, Ou T-T, Wu C-C, et al. Estradiol upregulates calcineurin expression via overexpression of estrogen receptor alpha gene in systemic lupus erythematosus. Kaohsiung J Med Sci (2011) 27:125–31. doi: 10.1016/j.kjms.2010.12.005
176. Rider V, Keltner S, Abdou NI. Increased estrogen-dependent expression of calcineurin in female SLE T cells is regulated by multiple mechanisms. J Gend Specif Med (2003) 6:14–21.
177. Maselli A, Conti F, Alessandri C, Colasanti T, Barbati C, Vomero M, et al. Low expression of estrogen receptor β in T lymphocytes and high serum levels of anti-estrogen receptor α antibodies impact disease activity in female patients with systemic lupus erythematosus. Biol Sex Differ (2016) 7:3. doi: 10.1186/s13293-016-0057-y
178. Roubinian JR, Talal N, Greenspan JS, Goodman JR, Siiteri PK. Effect of castration and sex hormone treatment on survival, anti-nucleic acid antibodies, and glomerulonephritis in NZB/NZW F1 mice. J Exp Med (1978) 147:1568–83. doi: 10.1084/jem.147.6.1568
179. Elbourne KB, Keisler D, McMurray RW. Differential effects of estrogen and prolactin on autoimmune disease in the NZB/NZW F1 mouse model of systemic lupus erythematosus. Lupus (1998) 7:420–7. doi: 10.1191/096120398678920352
180. Steward MW, Hay FC. Changes in immunoglobulin class and subclass of anti-DNA antibodies with increasing age in N/ZBW F1 hybrid mice. Clin Exp Immunol (1976) 26:363–70.
181. Roubinian JR, Papoian R, Talal N. Androgenic hormones modulate autoantibody responses and improve survival in murine lupus. J Clin Invest (1977) 59:1066–70. doi: 10.1172/JCI108729
182. Tower C, Crocker I, Chirico D, Baker P, Bruce I. SLE and pregnancy: the potential role for regulatory T cells. Nat Rev Rheumatol (2011) 7:124–8. doi: 10.1038/nrrheum.2010.124
183. Voskuhl RR, Patel K, Paul F, Gold SM, Scheel M, Kuchling J, et al. Sex differences in brain atrophy in multiple sclerosis. Biol Sex Differ (2020) 11:49. doi: 10.1186/s13293-020-00326-3
184. Wang K, Song F, Fernandez-Escobar A, Luo G, Wang J-H, Sun Y. The properties of cytokines in multiple sclerosis: Pros and cons. Am J Med Sci (2018) 356:552–60. doi: 10.1016/j.amjms.2018.08.018
185. Sicotte NL, Liva SM, Klutch R, Pfeiffer P, Bouvier S, Odesa S, et al. Treatment of multiple sclerosis with the pregnancy hormone estriol. Ann Neurol (2002) 52:421–8. doi: 10.1002/ana.10301
186. Cua DJ, Hinton DR, Stohlman SA. Self-antigen-induced Th2 responses in experimental allergic encephalomyelitis (EAE)-resistant mice. Th2-mediated suppression of autoimmune disease. J Immunol (1995) 155:4052–9.
187. Kim S, Voskuhl RR. Decreased IL-12 production underlies the decreased ability of male lymph node cells to induce experimental autoimmune encephalomyelitis. J Immunol (1999) 162:5561–8.
188. Itoh Y, Golden LC, Itoh N, Matsukawa MA, Ren E, Tse V, et al. The X-linked histone demethylase Kdm6a in CD4+ T lymphocytes modulates autoimmunity. J Clin Invest (2019) 129:3852–63. doi: 10.1172/JCI126250
189. Palaszynski KM, Loo KK, Ashouri JF, Liu H-B, Voskuhl RR. Androgens are protective in experimental autoimmune encephalomyelitis: implications for multiple sclerosis. J Neuroimmunol (2004) 146:144–52. doi: 10.1016/j.jneuroim.2003.11.004
190. Lélu K, Laffont S, Delpy L, Paulet P-E, Périnat T, Tschanz SA, et al. Estrogen receptor α signaling in T lymphocytes is required for estradiol-mediated inhibition of Th1 and Th17 cell differentiation and protection against experimental autoimmune encephalomyelitis. J Immunol (2011) 187:2386–93. doi: 10.4049/jimmunol.1101578
191. Subramanian S, Yates M, Vandenbark AA, Offner H. Oestrogen-mediated protection of experimental autoimmune encephalomyelitis in the absence of Foxp3+ regulatory T cells implicates compensatory pathways including regulatory b cells. Immunology (2011) 132:340–7. doi: 10.1111/j.1365-2567.2010.03380.x
192. Loetscher P, Moser B. Homing chemokines in rheumatoid arthritis. Arthritis Res (2002) 4:233–6. doi: 10.1186/ar412
193. Aggelakopoulou M, Kourepini E, Paschalidis N, Panoutsakopoulou V. ERβ in CD4+ T cells is crucial for ligand-mediated suppression of central nervous system autoimmunity. J Immunol (2016) 196:4947–56. doi: 10.4049/jimmunol.1600246
194. Garnier L, Laffont S, Lélu K, Yogev N, Waisman A, Guéry J-C. Estrogen signaling in bystander Foxp3neg CD4+ T cells suppresses cognate Th17 differentiation in trans and protects from central nervous system autoimmunity. J Immunol (2018) 201:3218–28. doi: 10.4049/jimmunol.1800417
195. Confavreux C, Hutchinson M, Hours MM, Cortinovis-Tourniaire P, Moreau T. Rate of pregnancy-related relapse in multiple sclerosis. N Engl J Med (1998) 339:285–91. doi: 10.1056/NEJM199807303390501
196. Iannello A, Rolla S, Maglione A, Ferrero G, Bardina V, Inaudi I, et al. Pregnancy epigenetic signature in T helper 17 and T regulatory cells in multiple sclerosis. Front Immunol (2018) 9:3075. doi: 10.3389/fimmu.2018.03075
197. Bodhankar S, Vandenbark AA, Offner H. Oestrogen treatment of experimental autoimmune encephalomyelitis requires 17β-oestradiol-receptor-positive b cells that up-regulate PD-1 on CD4+ Foxp3+ regulatory T cells. Immunology (2012) 137:282–93. doi: 10.1111/imm.12013
198. Zhang J, Benedek G, Bodhankar S, Lapato A, Vandenbark AA, Offner H. IL-10 producing b cells partially restore E2-mediated protection against EAE in PD-L1 deficient mice. J Neuroimmunol (2015) 285:129–36. doi: 10.1016/j.jneuroim.2015.06.002
199. Janssen M, Rijvers L, Koetzier SC, Wierenga-Wolf AF, Melief M-J, van Langelaar J, et al. Pregnancy-induced effects on memory b-cell development in multiple sclerosis. Sci Rep (2021) 11:12126. doi: 10.1038/s41598-021-91655-9
200. Koetzier SC, Neuteboom RF, Wierenga-Wolf AF, Melief M-J, de Mol CL, van Rijswijk A, et al. Effector T helper cells are selectively controlled during pregnancy and related to a postpartum relapse in multiple sclerosis. Front Immunol (2021) 12:642038. doi: 10.3389/fimmu.2021.642038
201. Raine C, Giles I. What is the impact of sex hormones on the pathogenesis of rheumatoid arthritis? Front Med (2022) 9:909879. doi: 10.3389/fmed.2022.909879
202. Mangalam AK, Rajagopalan G, Taneja V, David CS. HLA class II transgenic mice mimic human inflammatory diseases. In: Advances in immunology. (Orlando: Academic Press) (2008). p. 65–147.
203. Taneja V. Cytokines pre-determined by genetic factors are involved in pathogenesis of rheumatoid arthritis. Cytokine (2015) 75:216–21. doi: 10.1016/j.cyto.2014.11.028
204. Behrens M, Trejo T, Luthra H, Griffiths M, David CS, Taneja V. Mechanism by which HLA-DR4 regulates sex-bias of arthritis in humanized mice. J Autoimmun (2010) 35:1–9. doi: 10.1016/j.jaut.2009.12.007
205. Latham KA, Zamora A, Drought H, Subramanian S, Matejuk A, Offner H, et al. Estradiol treatment redirects the isotype of the autoantibody response and prevents the development of autoimmune arthritis. J Immunol (2003) 171:5820–7. doi: 10.4049/jimmunol.171.11.5820
206. Andersson A, Stubelius A, Karlsson MN, Engdahl C, Erlandsson M, Grahnemo L, et al. Estrogen regulates T helper 17 phenotype and localization in experimental autoimmune arthritis. Arthritis Res Ther (2015) 17:32. doi: 10.1186/s13075-015-0548-y
207. Ganesan K, Tiwari M, Balachandran C, Manohar BM, Puvanakrishnan R. Estrogen and testosterone attenuate extracellular matrix loss in collagen-induced arthritis in rats. Calcif Tissue Int (2008) 83:354–64. doi: 10.1007/s00223-008-9183-9
208. Keller ET, Chang C, Ershler WB. Inhibition of NFκB activity through maintenance of IκBα levels contributes to dihydrotestosterone-mediated repression of the interleukin-6 promoter. J Biol Chem (1996) 271:26267–75. doi: 10.1074/jbc.271.42.26267
209. Gubbels Bupp MR, Jorgensen TN. Androgen-induced immunosuppression. Front Immunol (2018) 9:794. doi: 10.3389/fimmu.2018.00794
210. Fernandez Lahore G, Förster M, Johannesson M, Sabatier P, Lönnblom E, Aoun M, et al. Polymorphic estrogen receptor binding site causes Cd2-dependent sex bias in the susceptibility to autoimmune diseases. Nat Commun (2021) 12:5565. doi: 10.1038/s41467-021-25828-5
211. Sims GP, Shiono H, Willcox N, Stott DI. Somatic hypermutation and selection of b cells in thymic germinal centers responding to acetylcholine receptor in myasthenia gravis. J Immunol (2001) 167:1935–44. doi: 10.4049/jimmunol.167.4.1935
212. Delpy L, Douin-Echinard V, Garidou L, Bruand C, Saoudi A, Guéry J-C. Estrogen enhances susceptibility to experimental autoimmune myasthenia gravis by promoting type 1-polarized immune responses. J Immunol (2005) 175:5050–7. doi: 10.4049/jimmunol.175.8.5050
213. Chuang W-Y, Ströbel P, Bohlender-Willke A-L, Rieckmann P, Nix W, Schalke B, et al. Late-onset myasthenia gravis - CTLA4(low) genotype association and low-for-age thymic output of naïve T cells. J Autoimmun (2014) 52:122–9. doi: 10.1016/j.jaut.2013.12.006
214. Marx A, Hohenberger P, Hoffmann H, Pfannschmidt J, Schnabel P, Hofmann H-S, et al. The autoimmune regulator AIRE in thymoma biology: autoimmunity and beyond. J Thorac Oncol (2010) 5:S266–72. doi: 10.1097/JTO.0b013e3181f1f63f
215. Aarli JA. Late-onset myasthenia gravis: a changing scene. Arch Neurol (1999) 56:25–7. doi: 10.1001/archneur.56.1.25
216. Rezzani R, Nardo L, Favero G, Peroni M, Rodella LF. Thymus and aging: morphological, radiological, and functional overview. Age (2014) 36:313–51. doi: 10.1007/s11357-013-9564-5
217. Gregersen PK, Kosoy R, Lee AT, Lamb J, Sussman J, McKee D, et al. Risk for myasthenia gravis maps to a (151) Pro→Ala change in TNIP1 and to human leukocyte antigen-B*08. Ann Neurol (2012) 72:927–35. doi: 10.1002/ana.23691
218. Janer M, Cowland A, Picard J, Campbell D, Pontarotti P, Newsom-Davis J, et al. A susceptibility region for myasthenia gravis extending into the HLA-class I sector telomeric to HLA-c. Hum Immunol (1999) 60:909–17. doi: 10.1016/S0198-8859(99)00062-2
219. Newsom-Davis J, Willcox N, Schluep M, Harcourt G, Vincent A, Mossman S, et al. Immunological heterogeneity and cellular mechanisms in myasthenia gravis. Ann N Y Acad Sci (1987) 505:12–26. doi: 10.1111/j.1749-6632.1987.tb51279.x
220. Holroyd CR, Edwards CJ. The effects of hormone replacement therapy on autoimmune disease: rheumatoid arthritis and systemic lupus erythematosus. Climacteric (2009) 12:378–86. doi: 10.1080/13697130903025449
221. Sanchez-Guerrero J, Karlson EW, Liang MH, Hunter DJ, Speizer FE, Colditz GA. Past use of oral contraceptives and the risk of developing systemic lupus erythematosus. Arthritis Rheum (1997) 40:804–8. doi: 10.1002/art.1780400505
222. Sicotte NL, Giesser BS, Tandon V, Klutch R, Steiner B, Drain AE, et al. Testosterone treatment in multiple sclerosis. Arch Neurol (2007) 64:683. doi: 10.1001/archneur.64.5.683
223. Gold SM, Chalifoux S, Giesser BS, Voskuhl RR. Immune modulation and increased neurotrophic factor production in multiple sclerosis patients treated with testosterone. J Neuroinflamm (2008) 5:32. doi: 10.1186/1742-2094-5-32
224. Morale MC, Batticane N, Bartoloni G, Guarcello V, Farinella Z, Galasso MG, et al. Blockade of central and peripheral luteinizing hormone-releasing hormone (LHRH) receptors in neonatal rats with a potent LHRH-antagonist inhibits the morphofunctional development of the thymus and maturation of the cell-mediated and humoral immune responses. Endocrinology (1991) 128:1073–85. doi: 10.1210/endo-128-2-1073
225. Jacobson JD, Nisula BC, Steinberg AD. Modulation of the expression of murine lupus by gonadotropin-releasing hormone analogs. Endocrinology (1994) 134:2516–23. doi: 10.1210/endo.134.6.8194477
226. Page ST, Plymate SR, Bremner WJ, Matsumoto AM, Hess DL, Lin DW, et al. Effect of medical castration on CD4+ CD25+ T cells, CD8+ T cell IFN-gamma expression, and NK cells: a physiological role for testosterone and/or its metabolites. Am J Physiol Endocrinol Metab (2006) 290:E856–63. doi: 10.1152/ajpendo.00484.2005
227. Jacobson JD. Gonadotropin-releasing hormone and G proteins: Potential roles in autoimmunity. Ann New York Acad Sci (2006) 917:809–18. doi: 10.1111/j.1749-6632.2000.tb05446.x
228. Sakurai K, Shinohara K, Imai T, Yamano Y, Hasegawa Y. Severe multiple sclerosis manifesting upon GnRH agonist therapy for uterine fibroids. Intern Med (2020) 59:3093–6. doi: 10.2169/internalmedicine.4839-20
229. Confavreux C, de Sèze J, Brassat D. Increased risk of multiple sclerosis relapse after in vitro fertilisation. JNNP (2012) 83(8):796–802. doi: 10.1136/jnnp-2012-302235
230. Torkildsen Ø, Holmøy T, Myhr K-M. Severe multiple sclerosis reactivation after gonadotropin treatment. Mult Scler Relat Disord (2018) 22:38–40. doi: 10.1016/j.msard.2018.02.031
231. Giefing-Kröll C, Berger P, Lepperdinger G, Grubeck-Loebenstein B. How sex and age affect immune responses, susceptibility to infections, and response to vaccination. Aging Cell (2015) 14:309–21. doi: 10.1111/acel.12326
232. Goetzl EJ, Huang M-C, Kon J, Patel K, Schwartz JB, Fast K, et al. Gender specificity of altered human immune cytokine profiles in aging. FASEB J (2010) 24:3580–9. doi: 10.1096/fj.10-160911
233. Márquez EJ, Chung C-H, Marches R, Rossi RJ, Nehar-Belaid D, Eroglu A, et al. Sexual-dimorphism in human immune system aging. Nat Commun (2020) 11:751. doi: 10.1038/s41467-020-14396-9
234. Anderson CL, Beever CL, Penaherrera MS. The dynamics of X-inactivation skewing as women age. Genetics (2004) 66(4):327–32. doi: 10.1111/j.1399-0004.2004.00310.x
235. Huang Z, Chen B, Liu X, Li H, Xie L, Gao Y, et al. Effects of sex and aging on the immune cell landscape as assessed by single-cell transcriptomic analysis. Proc Natl Acad Sci U.S.A. (2021) 118(33):e2023216118. doi: 10.1073/pnas.2023216118
236. Marttila S, Jylhävä J, Nevalainen T, Nykter M, Jylhä M, Hervonen A, et al. Transcriptional analysis reveals gender-specific changes in the aging of the human immune system. PloS One (2013) 8:e66229. doi: 10.1371/journal.pone.0066229
237. Yasui T, Maegawa M, Tomita J, Miyatani Y, Yamada M, Uemura H, et al. Changes in serum cytokine concentrations during the menopausal transition. Maturitas (2007) 56:396–403. doi: 10.1016/j.maturitas.2006.11.002
238. Desdín-Micó G, Soto-Heredero G, Aranda JF, Oller J, Carrasco E, Gabandé-Rodríguez E, et al. T Cells with dysfunctional mitochondria induce multimorbidity and premature senescence. Science (2020) 368:1371–6. doi: 10.1126/science.aax0860
239. Barrett ELB, Richardson DS. Sex differences in telomeres and lifespan. Aging Cell (2011) 10:913–21. doi: 10.1111/j.1474-9726.2011.00741.x
240. Yusipov I, Bacalini MG, Kalyakulina A, Krivonosov M, Pirazzini C, Gensous N, et al. Age-related DNA methylation changes are sex-specific: a comprehensive assessment. Aging (2020) 12:24057–80. doi: 10.18632/aging.202251
241. Hirokawa K, Utsuyama M, Hayashi Y, Kitagawa M, Makinodan T, Fulop T. Slower immune system aging in women versus men in the Japanese population. Immun Ageing (2013) 10:19. doi: 10.1186/1742-4933-10-19
242. Kamada M, Irahara M, Maegawa M, Yasui T, Takeji T, Yamada M, et al. Effect of hormone replacement therapy on post-menopausal changes of lymphocytes and T cell subsets. J Endocrinol Invest (2000) 23:376–82. doi: 10.1007/BF03343741
243. Jentsch-Ullrich K, Koenigsmann M, Mohren M, Franke A. Lymphocyte subsets’ reference ranges in an age- and gender-balanced population of 100 healthy adults–a monocentric German study. Clin Immunol (2005) 116:192–7. doi: 10.1016/j.clim.2005.03.020
244. Milan-Mattos JC, Anibal FF, Perseguini NM, Minatel V, Rehder-Santos P, Castro CA, et al. Effects of natural aging and gender on pro-inflammatory markers. Braz J Med Biol Res (2019) 52:e8392. doi: 10.1590/1414-431X20198392.
245. Vural P, Akgul C, Canbaz M. Effects of hormone replacement therapy on plasma pro-inflammatory and anti-inflammatory cytokines and some bone turnover markers in postmenopausal women. Pharmacol Res (2006) 54:298–302. doi: 10.1016/j.phrs.2006.06.006
246. Pietschmann P, Gollob E, Brosch S, Hahn P, Kudlacek S, Willheim M, et al. The effect of age and gender on cytokine production by human peripheral blood mononuclear cells and markers of bone metabolism. Exp Gerontol (2003) 38:1119–27. doi: 10.1016/s0531-5565(03)00189-x
247. Deguchi K, Kamada M, Irahara M, Maegawa M, Yamamoto S, Ohmoto Y, et al. Postmenopausal changes in production of type 1 and type 2 cytokines and the effects of hormone replacement therapy. Menopause (2001) 8:266–73. doi: 10.1097/00042192-200107000-00008
248. Rubtsova K, Marrack P, Rubtsov AV. Age-associated b cells: are they the key to understanding why autoimmune diseases are more prevalent in women? Expert Rev Clin (2012) 8(1):5–7. doi: 10.1586/eci.11.83
249. Rubtsova K, Rubtsov AV, Cancro MP, Marrack P. Age-associated b cells: A t-bet–dependent effector with roles in protective and pathogenic immunity. J Immunol (2015) 195:1933–7. doi: 10.4049/jimmunol.1501209
250. Ma S, Wang C, Mao X, Hao Y. B cell dysfunction associated with aging and autoimmune diseases. Front Immunol (2019) 10:318. doi: 10.3389/fimmu.2019.00318
251. Rubtsova K, Marrack P, Rubtsov AV. Sexual dimorphism in autoimmunity. J Clin Invest (2015) 125:2187–93. doi: 10.1172/JCI78082
252. Sachinidis A, Xanthopoulos K, Garyfallos A. Age-associated b cells (ABCs) in the prognosis, diagnosis and therapy of systemic lupus erythematosus (SLE). Mediterr J Rheumatol (2020) 31:311–8. doi: 10.31138/mjr.31.3.311
253. Jenks SA, Cashman KS, Zumaquero E, Marigorta UM, Patel AV, Wang X, et al. Distinct effector b cells induced by unregulated toll-like receptor 7 contribute to pathogenic responses in systemic lupus erythematosus. Immunity (2020) 52:203. doi: 10.1016/j.immuni.2019.12.005
254. Churov AV, Mamashov KY, Novitskaia AV. Homeostasis and the functional roles of CD4+ treg cells in aging. Immunol Lett (2020) 226:83–9. doi: 10.1016/j.imlet.2020.07.004
255. Herz J, Köster C, Crasmöller M, Abberger H, Hansen W, Felderhoff-Müser U, et al. Peripheral T cell depletion by FTY720 exacerbates hypoxic-ischemic brain injury in neonatal mice. Front Immunol (2018) 9:1696. doi: 10.3389/fimmu.2018.01696
256. Fairweather D, Frisancho-Kiss S, Rose NR. Sex differences in autoimmune disease from a pathological perspective. Am J Pathol (2008) 173:600–9. doi: 10.2353/ajpath.2008.071008
257. Kosyreva AM, Makarova OV, Kakturskiy LV, Mikhailova LP, Boltovskaya MN, Rogov KA. Sex differences of inflammation in target organs, induced by intraperitoneal injection of lipopolysaccharide, depend on its dose. J Inflammation Res (2018) 11:431–45. doi: 10.2147/JIR.S178288
258. Morgan CP, Bale TL. Sex differences in microRNA regulation of gene expression: no smoke, just miRs. Biol Sex Differ (2012) 3:22. doi: 10.1186/2042-6410-3-22
259. Morgan CP, Bale TL. Early prenatal stress epigenetically programs dysmasculinization in second-generation offspring via the paternal lineage. J Neurosci (2011) 31:11748–55. doi: 10.1523/JNEUROSCI.1887-11.2011
260. Voskuhl R. Sex differences in autoimmune diseases. Biol Sex Differ (2011) 2:1. doi: 10.1186/2042-6410-2-1
261. Beckmann L, Obst S, Labusek N, Abberger H, Köster C, Klein-Hitpass L, et al. Regulatory T cells contribute to sexual dimorphism in neonatal hypoxic-ischemic brain injury. Stroke (2022) 53(2):381–90. STROKEAHA121037537. doi: 10.1161/STROKEAHA.121.037537
262. Maranini B, Bortoluzzi A, Silvagni E, Govoni M. Focus on sex and gender: What we need to know in the management of rheumatoid arthritis. J Pers Med (2022) 12(3):499. doi: 10.3390/jpm12030499
263. Li Y, Qi W, Yan L, Wang M, Zhao L. Tripterygium wilfordii derivative LLDT-8 targets CD2 in the treatment of rheumatoid arthritis. BioMed Rep (2021) 15:81. doi: 10.3892/br.2021.1457
264. Castagnetta LA, Carruba G, Granata OM, Stefano R, Miele M, Schmidt M, et al. Increased estrogen formation and estrogen to androgen ratio in the synovial fluid of patients with rheumatoid arthritis. J Rheumatol (2003) 30:2597–605.
265. Straub RH, Härle P, Sarzi-Puttini P, Cutolo M. Tumor necrosis factor-neutralizing therapies improve altered hormone axes: an alternative mode of antiinflammatory action. Arthritis Rheum (2006) 54:2039–46. doi: 10.1002/art.21946
266. Cutolo M, Sulli A, Capellino S, Villaggio B, Montagna P, Pizzorni C, et al. Anti-TNF and sex hormones. Ann N Y Acad Sci (2006) 1069:391–400. doi: 10.1196/annals.1351.037
267. Capellino S, Riepl B, Rauch L, Angele P, Cutolo M, Straub RH. Quantitative determination of steroid hormone receptor positive cells in the synovium of patients with rheumatoid arthritis and osteoarthritis: is there a link to inflammation? Ann Rheum Dis (2007) 66:53–8. doi: 10.1136/ard.2006.055483
268. Spitzer JA. Gender differences in some host defense mechanisms. Lupus (1999) 8:380–3. doi: 10.1177/096120339900800510
269. Weinstein Y, Ran S, Segal S. Sex-associated differences in the regulation of immune responses controlled by the MHC of the mouse. J Immunol (1984) 132:656–61.
270. Rettew JA, Huet-Hudson YM, Marriott I. Testosterone reduces macrophage expression in the mouse of toll-like receptor 4, a trigger for inflammation and innate immunity. Biol Reprod (2008) 78:432–7. doi: 10.1095/biolreprod.107.063545
271. D’agostino P, Milano S, Barbera C, Bella G, Rosa M, Ferlazzo V, et al. Sex hormones modulate inflammatory mediators produced by macrophagesa. Ann N Y Acad Sci (1999) 876:426–9. doi: 10.1111/j.1749-6632.1999.tb07667.x
272. Hughes GC, Clark EA. Regulation of dendritic cells by female sex steroids: relevance to immunity and autoimmunity. Autoimmunity (2007) 40:470–81. doi: 10.1080/08916930701464764
273. Seillet C, Rouquié N, Foulon E, Douin-Echinard V, Krust A, Chambon P, et al. Estradiol promotes functional responses in inflammatory and steady-state dendritic cells through differential requirement for activation function-1 of estrogen receptor α. J Immunol (2013) 190:5459–70. doi: 10.4049/jimmunol.1203312
274. Papenfuss TL, Powell ND, McClain MA, Bedarf A, Singh A, Gienapp IE, et al. Estriol generates tolerogenic dendritic cells in vivo that protect against autoimmunity. J Immunol (2011) 186:3346–55. doi: 10.4049/jimmunol.1001322
275. Gaya da Costa M, Poppelaars F, van Kooten C, Mollnes TE, Tedesco F, Würzner R, et al. Age and sex-associated changes of complement activity and complement levels in a healthy Caucasian population. Front Immunol (2018) 9:2664. doi: 10.3389/fimmu.2018.02664
276. Kamitaki N, Sekar A, Handsaker RE, de Rivera H, Tooley K, Morris DL, et al. Complement genes contribute sex-biased vulnerability in diverse disorders. Nature (2020) 582:577–81. doi: 10.1038/s41586-020-2277-x
277. Wu M, Rowe JM, Fleming SD. Complement initiation varies by sex in intestinal ischemia reperfusion injury. Front Immunol (2021) 12:649882. doi: 10.3389/fimmu.2021.649882
278. Kotimaa J, Klar-Mohammad N, Gueler F, Schilders G, Jansen A, Rutjes H, et al. Sex matters: Systemic complement activity of female C57BL/6J and BALB/cJ mice is limited by serum terminal pathway components. Mol Immunol (2016) 76:13–21. doi: 10.1016/j.molimm.2016.06.004
279. Rosser EC, de Gruijter NM, Matei DE. Mini-review: Gut-microbiota and the sex-bias in autoimmunity - lessons learnt from animal models. Front Med (2022) 9:910561. doi: 10.3389/fmed.2022.910561
280. McGee JS, Huttenhower C. Of mice and men and women: Sexual dimorphism of the gut microbiome. Int J Womens Dermatol (2021) 7:533–8. doi: 10.1016/j.ijwd.2021.10.007
281. Kim YS, Unno T, Kim BY, Park MS. Sex differences in gut microbiota. World J Mens Health (2020) 38:48–60. doi: 10.5534/wjmh.190009
282. Elderman M, de Vos P, Faas M. Role of microbiota in sexually dimorphic immunity. Front Immunol (2018) 9:1018. doi: 10.3389/fimmu.2018.01018
283. Borchers AT, Gershwin ME. Sociological differences between women and men: implications for autoimmunity. Autoimmun Rev (2012) 11:A413–21. doi: 10.1016/j.autrev.2011.11.016
284. Rubtsov AV, Rubtsova K, Kappler JW, Marrack P. Genetic and hormonal factors in female-biased autoimmunity. Autoimmun Rev (2010) 9:494–8. doi: 10.1016/j.autrev.2010.02.008
285. Fransen F, van Beek AA, Borghuis T, Meijer B, Hugenholtz F, van der Gaast-de Jongh C, et al. The impact of gut microbiota on gender-specific differences in immunity. Front Immunol (2017) 8:754. doi: 10.3389/fimmu.2017.00754
286. Yurkovetskiy L, Burrows M, Khan AA, Graham L, Volchkov P, Becker L, et al. Gender bias in autoimmunity is influenced by microbiota. Immunity (2013) 39:400–12. doi: 10.1016/j.immuni.2013.08.013
287. Markle JGM, Frank DN, Mortin-Toth S, Robertson CE, Feazel LM, Rolle-Kampczyk U, et al. Sex differences in the gut microbiome drive hormone-dependent regulation of autoimmunity. Science (2013) 339:1084–8. doi: 10.1126/science.1233521
288. Edwards M, Dai R, Ahmed SA. Our environment shapes us: The importance of environment and sex differences in regulation of autoantibody production. Front Immunol (2018) 9:478. doi: 10.3389/fimmu.2018.00478
289. Angeloni B, Bigi R, Bellucci G, Mechelli R, Ballerini C, Romano C, et al. A case of double standard: Sex differences in multiple sclerosis risk factors. Int J Mol Sci (2021) 22(7):3696. doi: 10.3390/ijms22073696
290. Mooney LA, Perera FP, Van Bennekum AM, Blaner WS, Karkoszka J, Covey L, et al. Gender differences in autoantibodies to oxidative DNA base damage in cigarette smokers. Cancer Epidemiol Biomarkers Prev (2001) 10:641–8.
291. Guggenmos J, Schubart AS, Ogg S, Andersson M, Olsson T, Mather IH, et al. Antibody cross-reactivity between myelin oligodendrocyte glycoprotein and the milk protein butyrophilin in multiple sclerosis. J Immunol (2004) 172:661–8. doi: 10.4049/jimmunol.172.1.661
292. Versini M, Jeandel P-Y, Rosenthal E, Shoenfeld Y. Obesity in autoimmune diseases: not a passive bystander. Autoimmun Rev (2014) 13:981–1000. doi: 10.1016/j.autrev.2014.07.001
293. Nicot A. Gender and sex hormones in multiple sclerosis pathology and therapy. Front Biosci (2009) 14:4477–515. doi: 10.2741/3543
294. Elbers JM, Asscheman H, Seidell JC, Frölich M, Meinders AE, Gooren LJ. Reversal of the sex difference in serum leptin levels upon cross-sex hormone administration in transsexuals. J Clin Endocrinol Metab (1997) 82:3267–70. doi: 10.1210/jcem.82.10.4284
295. Correale J, Ysrraelit MC, Gaitán MI. Gender differences in 1,25 dihydroxyvitamin D3 immunomodulatory effects in multiple sclerosis patients and healthy subjects. J Immunol (2010) 185:4948–58. doi: 10.4049/jimmunol.1000588
Keywords: lymphocytes, sex, sex-bias, T cells, B cells, estrogen, testosterone
Citation: Dodd KC and Menon M (2022) Sex bias in lymphocytes: Implications for autoimmune diseases. Front. Immunol. 13:945762. doi: 10.3389/fimmu.2022.945762
Received: 24 May 2022; Accepted: 03 November 2022;
Published: 24 November 2022.
Edited by:
Trine N. Jorgensen, Case Western Reserve University, United StatesReviewed by:
Dolores Njoku, Washington University in St. Louis, United StatesDorothee Schwinge, University Medical Center Hamburg-Eppendorf, Germany
Copyright © 2022 Dodd and Menon. This is an open-access article distributed under the terms of the Creative Commons Attribution License (CC BY). The use, distribution or reproduction in other forums is permitted, provided the original author(s) and the copyright owner(s) are credited and that the original publication in this journal is cited, in accordance with accepted academic practice. No use, distribution or reproduction is permitted which does not comply with these terms.
*Correspondence: Madhvi Menon, madhvi.menon@manchester.ac.uk