- 1Biological Resource Center, Taizhou Hospital of Zhejiang Province, Wenzhou Medical University, Linhai, China
- 2Key Laboratory of Minimally Invasive Techniques & Rapid Rehabilitation of Digestive System Tumor of Zhejiang Province, Taizhou Hospital of Zhejiang Province, Linhai, China
- 3Medical Research Center, Taizhou Hospital of Zhejiang Province, Wenzhou Medical University, Linhai, China
Immune checkpoint inhibitors (ICIs) have become a promising immunotherapy for cancers. Human leukocyte antigen-G (HLA-G), a neoantigen, its biological functions and clinical relevance have been extensively investigated in malignancies, and early clinical trials with “anti-HLA-G strategy” are being launched for advance solid cancer immunotherapy. The mechanism of HLA-G as a new ICI is that HLA-G can bind immune cell bearing inhibitory receptors, the immunoglobulin-like transcript (ILT)-2 and ILT-4. HLA-G/ILT-2/-4 (HLA-G/ILTs) signaling can drive comprehensive immune suppression, promote tumor growth and disease progression. Though clinical benefits could be expected with application of HLA-G antibodies to blockade the HLA-G/ILTs signaling in solid cancer immunotherapy, major challenges with the diversity of HLA-G isoforms, HLA-G/ILTs binding specificity, intra- and inter-tumor heterogeneity of HLA-G, lack of isoform-specific antibodies and validated assay protocols, which could dramatically affect the clinical efficacy. Clinical benefits of HLA-G-targeted solid cancer immunotherapy may be fluctuated or even premature unless major challenges are addressed.
Introduction
Immune checkpoint inhibitors have become a promising immunotherapy for cancers, but durable clinical benefits are limited for existing agents (1). It’s an exciting news released in Cancer Discovery “Gilead Buys into Tizona’s Anti-HLA-G Strategy” that an early clinical trial with human leukocyte antigen-G (HLA-G) inhibitor TTX-080 is being launched for advance solid cancer patients (NCT04485013) (2).
HLA-G, firstly observed on extravillous cytotrophoblast, has been considered to play critical roles in maintaining maternal immune tolerance for the semi-allograft fetus during pregnancy (3, 4). In the context of malignancies, aberrant HLA-G expression in melanoma lesions but not adjacent normal tissues was reported by Paul and co-workers in 1998 for the first time (5). This pioneering investigation has been testified with thousands of samples in more than 30 types of cancers. Ever increasing studies on HLA-G expression by solid tumor lesions have revealed that high levels of HLA-G expression was associated with advanced disease stage, tumor metastasis, poor prognosis, or shorter disease-free survival. However, either among patients with different types of cancers, or among patients with the same type of cancer, intertumor and intratumor heterogeneity of HLA-G expression is evident, such as among patients with breast cancer (6–15), colorectal cancer (16–24), cervical cancer (25–27), endometrial cancer (28–31), esophageal squamous cell carcinoma (32–34), Ewing sarcoma (35), gastric cancer (36–38), glioblastoma (39), hepatocellular carcinoma (40–42), lung cancer (43–45), classical Hodgkin lymphoma (46, 47), diffuse large B-cell lymphoma (48), cutaneous T- and B-cell lymphoma (49), nasopharyngeal carcinoma (50), oral squamous cell carcinoma (51), ovarian cancer (52–55), pancreatic adenocarcinoma (56–59), and thyroid cancer (60, 61) (Table 1). HLA-G expression in solid cancers is now well acknowledged in promoting cancer cell immune escaping and tumor development, and associated with disease progression and poor survival either among cancer patients or pre-clinical murine models (62).
Engagement of HLA-G/ILTs can induce either unresponsive or tolerogenic state of a wide range of immune effector cells. The underlying mechanisms for HLA-G as an immune checkpoint is that HLA-G can either directly bind tyrosine-based inhibitory motifs (ITIMs) containing immune inhibitory receptors, the immunoglobulin-like transcript (ILT)-2/CD85j/LILRB1 and ILT-4/CD85d/LILRB2, which is expressed on various immune competent cells such as T lymphocytes, natural killer cells (NK), dendritic cells (DC) (62), or indirectly by intercellular transfer by the process of trogocytosis and exosomes to drive a comprehensive immune suppression (63). Immune suppression induced by the HLA-G/ILTs signaling pathway includes inhibition of cytotoxicity (64), inflammatory cytokine production (65), chemotaxis and proliferation of T cells and NK cells (66, 67), inhibition antibody production of B cells and maturation of antigen presenting cells (68, 69), and dampen the anti-tumor functions of invariant natural killer T (iNKT) cells and tumor-infiltrating CD8+PD-1−ILT-2+ T cells (70, 71). Also, HLA-G/ILTs engagement can also induce expansion of myeloid derived suppressive cells (MDSCs) and generation of regulatory T cells (72, 73). In addition to immune suppressive functions, HLA-G/ILTs can promote intratumor vascular remodeling by enhancing vascular endothelial growth factor-C (VEGF-C) expression, and increase tumor metastasis by inducing cancer promoting factor matrix metalloproteinases (MMPs) expression (74, 75) (Figure 1). Consequently, both innate and adaptive antitumor immune responses are impaired, thus favoring tumor cell immune evasion and disease progression. In this scenario, restoring antitumor functions of ILT-bearing immune cells with HLA-G inhibitors such as TTX-080 sounds reasonable.
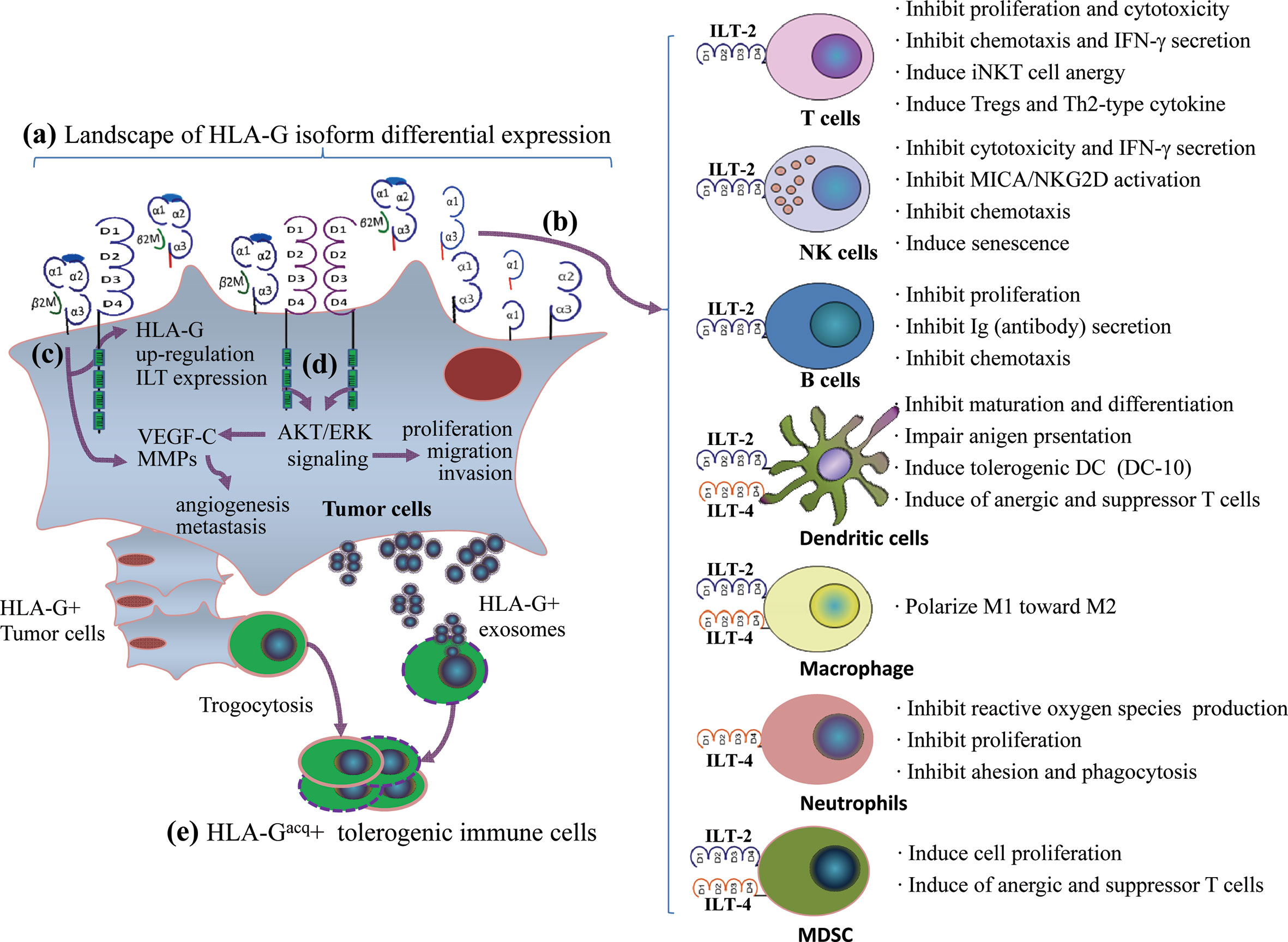
Figure 1 A comprehensive immune suppression mediated by HLA-G/ILTs engagement in cancer development. (A) Distinct profiles of HLA-G isoform expression in an individual cancer patient. The heterogeneous landscape of HLA-G isoform differential expression among cancer patients can be temporal and spatial dependent. (B) Immune inhibitory receptors ILT-2 and ILT-4 are expressed on different immune cell. ILT-2 recognizes HLA-G1 and HLA-G5 while ILT-4 recognizes HLA-G1, -G2, -G5, and HLA-G6 isoforms. HLA-G isoform-dependent ILT-2 and ILT-4 engagement induces a wide spectrum of immune suppression which benefits cancer cell escaping from host immune surveillance and anti-tumor immunity. (C) HLA-G expression up-regulates intratumor ILTs and MMPs expression. (D) ILTs induce VEGF-C expression and enhance cancer cell proliferation, migration and invasion through AKT/ERK signaling, which favors cancer cell angiogenesis and metastasis. (E) In addition to direct binding between HLA-G and ILTs, immune cells acquire HLA-G from neighboring HLA-G+ cancer cells through contact-dependent trogocytosis and from cancer cell derived HLA-G-bearing exosomes in a long-distance. HLA-G acquired immune cells became tolerogenic phenotype and immune functions are impaired.
As HLA-G expression is specifically induced in most types of solid cancer cells, clinical benefits of HLA-G inhibitors could be expected for cancer immunotherapy. However, challenges such as multiple HLA-G isoforms with distinct extracellular domains, different binding sites between HLA-G and ILT-2/ILT-4 interaction, intratumor or intertumor heterogeneity of HLA-G expression, and isoform-specific antibody and validated assay protocol lacking, remain tremendous hurdle in terms of HLA-G/ILTs antibody-based solid cancer immunotherapy.
HLA-G Isoforms Molecular Structure
The HLA-G gene contains eight exons and seven introns. However, most full-length transcripts carry only seven exons because exon seven is usually spliced out. Due to a premature stop codon in E6, the HLA-G full-length protein has 338-amino acids, which is relatively shorter compared with classical HLA class I molecules. Among these exons, E1 generates the signal peptide, E2-E4 generate extracellular α1, α2, and α3 domains, respectively. E5 generates the transmembrane domain, and E6 generates the intracellular cytoplasmic tail of HLA-G (76).
Due to its primary transcript alternative splicing, diverse molecular structures of HLA-G have been observed. Seven HLA-G isoforms including four membrane-bound (HLA-G1–HLA-G4) and three soluble (HLA-G5–HLA-G7) monomers have been identified. With a premature stop codon in E6, membrane-bound (HLA-G1–HLA-G4) isoforms have a unique truncated cytoplasmic tail comparing to other classic HLA class I molecules. Soluble HLA-G5 and HLA-G6 isoforms are resulted from a stop codon in intron 4, and HLA-G7 are generated from a stop codon in intron 2, which prevents the translation of their transmembrane domain (77, 78) (Figure 2).
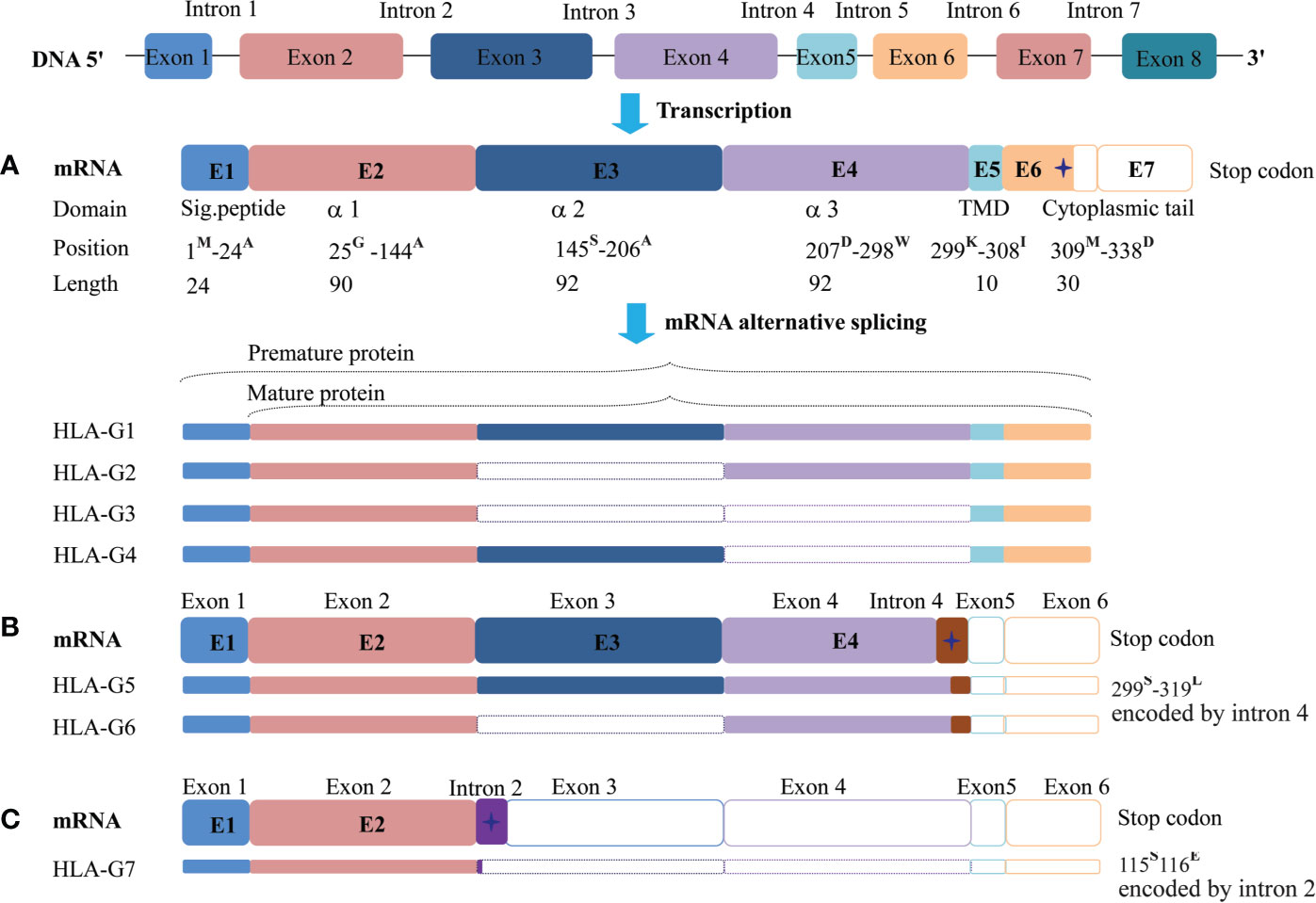
Figure 2 Seven identified HLA-G isoforms generated from its primary transcript alternative splicing. (A) The heavy chain of membrane-bound isoforms HLA-G1, -G2, -G3, -G4 generated by an mRNA containing a stop codon in exon 6. (B) Soluble isoforms HLA-G5 and HLA-G6 generated by an mRNA with a pre-stop codon in intron 4, which terminates transmembrane and cytoplasmic tail transcription. (C) Soluble isoforms HLA-G7 generated by an mRNA with a pre-stop codon in intron 2, which terminates the following domain transcription. Sig.peptide, Signal peptide; TMD, transmembrane domain; , stop codon. The superscript capital letter represents amino acid at the position.
Each HLA-G isoform has its unique extracellular structure. HLA-G1 is the only full-length isoform with extracellular α1, α2, and α3 domains; HLA-G2 has α1 and α3 domains; HLA-G3 has the only α1 domains; HLA-G4 has α1 and α2 domains. Similarly, HLA-G5 has the extracellular α1, α2, and α3 domains; HLA-G6 has α1 and α3 domains, and HLA-G7 has the only α1 domains. α1 and α2 domains form the peptide binding cleft, and α3 domain non-covalently bind to the light chain β2-microglobulin (β2m). Novel HLA-G isoforms such as lacking a transmembrane region and α1 domain have been predicted with RNAseq technology (79) (Figure 3). Moreover, higher molecular weight of HLA-G has been associated with post-translational modifications. Homo- and hetero-HLA-G dimers can be formed through intermolecular disulfide bonds with Cys42 or Cys147 in the extracellular α1 or α2 domain; others such as glycosylated, nitrated, and ubiquitinated HLA-G molecules have also been confirmed (80–83).
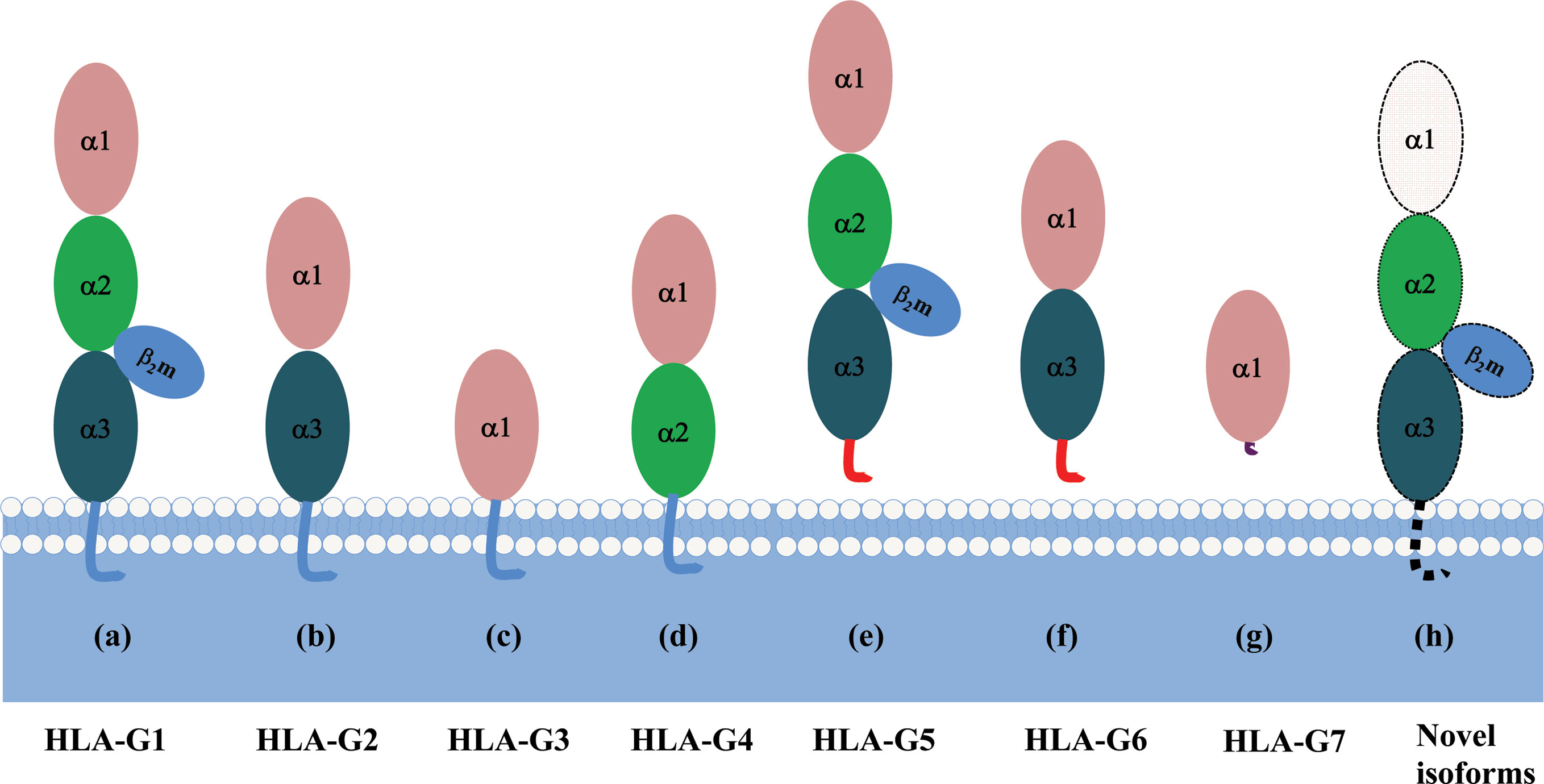
Figure 3 A schematic structure of HLA-G isoforms. (A) HLA-G1 have α1, α2, and α3 extracellular domains; (B) HLA-G2 have α1, and α3 extracellular domains; (C) HLA-G3 have α1 extracellular domains; (D) HLA-G4 have α1, and α2 extracellular domains; (E) HLA-G5 have α1, α2, and α3 extracellular domains; (F) HLA-G6 have α1 and α3 extracellular domains; (G) HLA-G7 has α1 extracellular domain followed by two C- terminal amino-acids encoded by intron 2; (H) Novel HLA-G isoforms such as lacking a transmembrane region and α1 domain have been predicted, but their structure remains confirmed.
HLA-G/ILTs Binding
ILT-2 and ILT-4 belong to the type I transmembrane glycoproteins, which have four extracellular immunoglobulin-like domains (D1-D4), a transmembrane region, and an intracellular tail with four or three immunoreceptor tyrosine-based inhibitory motifs (ITIMs). ILT-2 can be found on a variety of immune cells, such as subpopulations of T cells, B cells, natural killer (NK) cells, myeloid-derived suppressive cells (MDSCs), dendritic cells (DCs), and monocytes/macrophages. ILT-4 is not expressed on lymphocytes, but on monocytes/macrophages, neutrophils, basophils, DCs, and MDSCs (84, 85).
A recent study revealed that ILT-2/-4 extracellular D1D2 are responsible for the interaction with HLA-G binding, while D3D4 act as a scaffold (86). Also, ILT-2/-4 are more accessible to the HLA-G dimer binding than that of HLA-G monomer, leading to much stronger inhibitory signals. ILT-2 and ILT-4 extracellular D1D2 binds to the extracellular α3 domain of HLA-G, but structurally dependents. ILT-2 only binds to the HLA-G heavy chain associated with β2m, while ILT-4 can bind to both β2m free HLA-G heavy chain and HLA-G heavy chain with β2m. Moreover, residues Tyr38 and Tyr76 in ILT-2 are responsible for binding to HLA-G Phe195 in the α3 domain, whereas residues Tyr36 and Arg38 in ILT-4 interact with the Phe195–Tyr197 loop in the HLA-G α3 domain (87). The different binding sites between ILT-2/-4 and HLA-G could be an explanation for the higher affinity of ILT-4 than the affinity of ILT-2 when they interact with HLA-G (88). Based on the different extracellular structure of HLA-G isoforms and ILT binding characteristics, ILT2 can bind to the β2m associated HLA-G1 and HLA-G5 isoforms. However, ILT-4 can bind to both β2m associated or β2m free HLA-G isoforms, which include HLA-G1, -G2, -G4, -G5 and HLA-G6 isoforms (Figure 4).
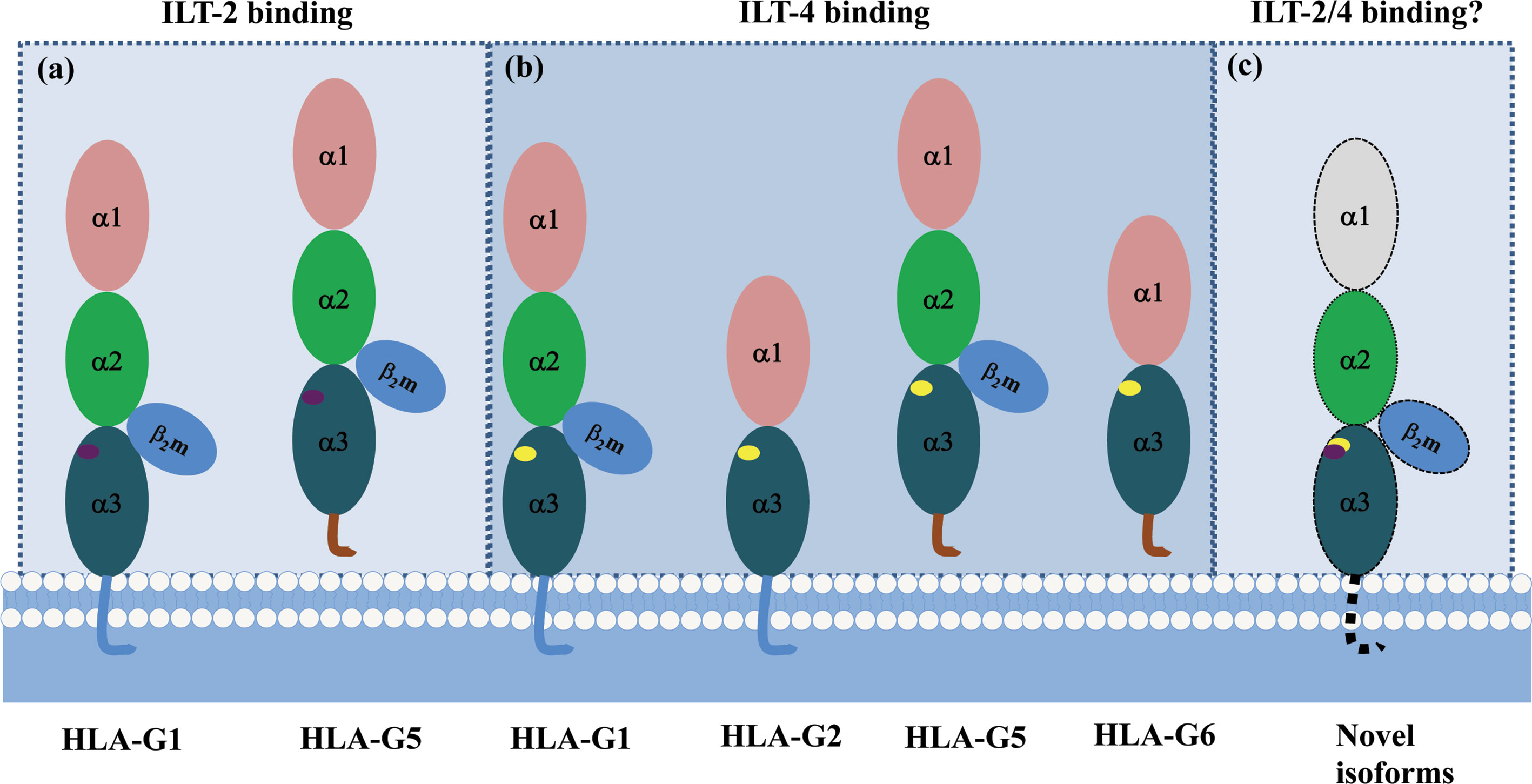
Figure 4 Different binding sites between HLA-G/ILT-2 and HLA-G/ILT-4. ILT-2 residues Tyr38 and Tyr76 bind Phe195 in HLA-G α3 domain, ILT-4 residues Tyr36 and Arg38 bind Phe195–Tyr197 loop in HLA-G α3 domain. (A) ILT-2 binds HLA-G heavy chain associated with β2m (HLA-G1 and HLA-G5). (B) ILT-4 binds both β2m-free (HLA-G2 and HLA-G6) and β2m-associated (HLA-G1 and HLA-G5) HLA-G heavy chain. (C) A panel of novel HLA-G isoforms including isoforms without α1 domain and transmembrane region, or with an extended 5’-region generated by HLA-G mRNA alternative splicing were predicted. However, molecular structure of these novel isoforms and remain to be identified, and interaction with ILTs is unknown yet. and
represent ILT-2 and ILT-4 binding site in HLA-G isoforms.
Taking advantage of the early studies on fetal-maternal immune tolerance, HLA-G-mediated immune inhibition has been well-acknowledged in the broader spectrum of health and disease situations. HLA-G expression favors the acceptance of allograft organ transplantation, whereas it provides an additional strategy for cancer cells to escape from immune surveillance and clearance (89, 90). HLA-G/ILTs engagement activates the phosphorylation of the ITIMs contained in the tyrosine residues, which present docking sites for Src homology 2 (SH2) protein tyrosine phosphates SHP-1 and SHP-2, thus initiating an inhibitory signaling cascade. Meanwhile, ITIM-dependent recruitment of SHP1/SHP2 can markedly suppress the ITAM activated Syk/Src signal cascades in immune cell activation (91).
Challenges in HLA-G/ILTs Targeted Solid Cancer Immunotherapy
One strategy successfully deployed by cancer cells for immune evasion is the impairment of the classical HLA class I and II antigens to hide infected cells from T cell recognition, while aberrant induction of HLA-G expression by cancer cells makes host anti-tumor immune system rather vulnerable (92). Though development of HLA-G/ILTs interaction targeted ICIs is promising for cancer immunotherapy, much real-world information on HLA-G/ILTs status is extremely necessary for both future basic and clinical investigations.
Lack of HLA-G Isoform-Specific Monoclonal Antibodies
The most fundamental task is to develop HLA-G molecule universal or distinct HLA-G isoform-specific mAbs. As high as 80% amino acid sequence identity sharing in the extracellular domain of all HLA class I antigens, only distinct feature of HLA-G is its molecular weight of 39 kDa (HLA-G1 isoform) which is less than 45 kDa of the other classical HLA class I antigens (93), and this is rather similar to other non-classical HLA class I antigens including HLA-E and HLA-F (https://www.uniprot.org/uniprot/P17693; https://www.uniprot.org/uniprot/P13747; https://www.uniprot.org/uniprot/P30511). CLUSTALO sequence alignment results showed that, among HLA-G, HLA-F, and HLA-E molecules, the full-length amino acid sequence are identity round 62%, 75.9% between HLA-G and HLA-F, 71.2% between HLA-G and HLA-E, and 40.1% between HLA-E and HLA-F (Supplementary Figure 1). Thus, the cross-reactivity of anti-HLA-G mAbs to other classical and non-classical HLA class I antigens remains a huge task to be improved (94). Indeed, cross-reactivity of the most widely used such as mAb 4H84 for denatured HLA-G form has been observed to react with other HLA class I antigens (95, 96). Moreover, due to HLA-G primary transcript alternative splicing, in addition to seven ever-identified HLA-G isoforms and more novel isoforms can be expected, lack of HLA-G isoform-specific mAb prevents advances in characterizing their biological functions and clinical significance (97). To be noted, a panel of novel HLA-G isoforms predicted by deep transcriptome analysis have been reported. Among these isoforms, HLA-G1L have extra five amino acids (NKTPR) ahead the beginning residue Methionine at the N-terminal ends, others such as isoforms lack α1 or both α1 and α2 extracellular domains, and novel soluble HLA-G isoforms with distinct C-terminal ends generated by skipping exons 5 and 6.
Among anti-HLA-G antibodies, mAbs 4H84 and 5A6G7 are with known recognizing epitope in HLA-G heavy chain. mAb 4H84 generated by amino acid residues from 61 to 83 (in α1 domain HLA-G, which probes all denatured HLA-G isoforms (98). mAb 5A6G7 generated by a 22-mer C-terminal amino acid sequence in HLA-G5 and HLA-G6, which probes both native and denatured HLA-G5 and HLA-G6 isoforms (99, 100). mAbs 4H84 and 5A6G7 are the most widely used antibodies for evaluating total HLA-G (mAbs 4H84 and MEM-G/1) or HLA-G5/6 isoforms (mAb 5A6G7) respectively in malignant lesions with immunohistochemistry. As a result, novel isoforms without α1 domain can’t be detected by the mAb 4H84 (23, 79). In this scenario, the interpretation of clinical significance of HLA-G in cancers seems rather premature unless more reliable specific anti-HLA-G mAbs are used (Figure 5).
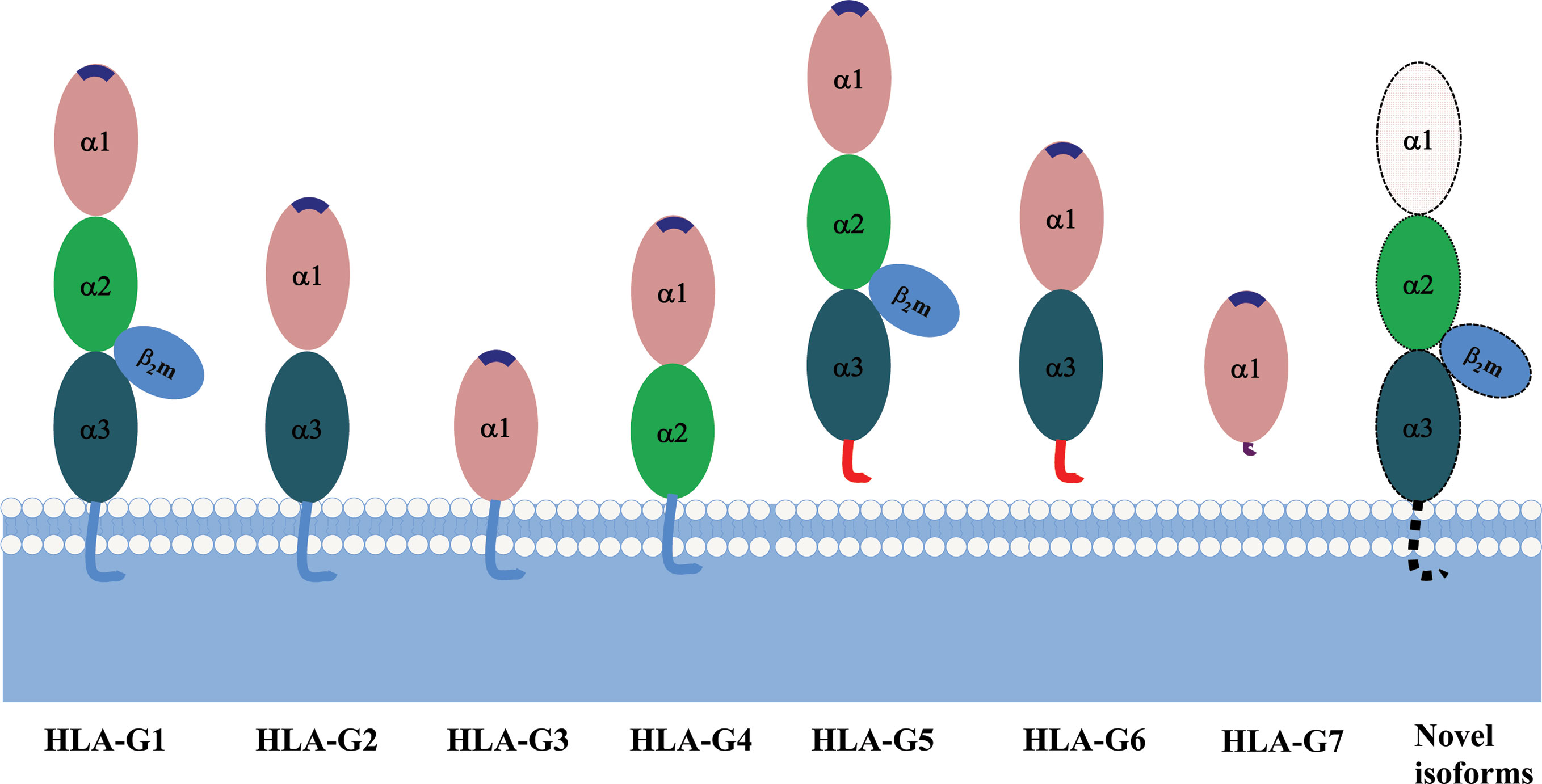
Figure 5 Epitopes in HLA-G recognized by mAbs 4H84 and 5A6G7. Among HLA-G antibodies, only mAbs 4H84 and 5A6G7 were generated with definite immunogen epitopes. mAb 4H84 generated by 61st~83rd amino acids (EEETRNTKAHAQTDRMNLQTLRG) in HLA-G α1 domain which recognizes denatured heavy chain of all seven identified HLA-G isoforms containing α1 domain. mAb 5A6G7 generated by 21-mer C-terminal amino acid (SKEGDGGIMSVRESRSLSEDL) in HLA-G5 and -G6 isoforms, which recognizes both native and denatured heavy chain of HLA-G5 and HLA-G6 isoforms. Novel HLA-G isoforms such as isoforms without α1 domain and transmembrane region, or with an extended 5’-region were predicted. However, no current antibody is available to detect. and
represent mAb 4H84 and 5A6G7 recognizing site in HLA-G isoforms.
Furthermore, evaluation criteria for lesion HLA-G expression including staining protocols, cut-off levels, and cross-assay concordance are far from standardized, which could dramatically affect the definition of HLA-G expression and interpretation of its clinical significance, even with a same mAb to detect HLA-G within a certain type of cancer (62, 96). In this context, a reference criterion should be recommended by international community for HLA-G staining to minimize discrepancies across studies is urgently warranted.
Also, given different binding specificity of HLA-G between ILT-2 and ILT-4 resulted from its extracellular structure, that ILT-2 binds HLA-G1 and HLA-G5 while ILT-4 binds HLA-G1, -G2, -G4, -G5, and HLA-G6 isoforms, the landscape of HLA-G isoforms and their degree of expression in cancers can tremendously affect the benefits of HLA-G/ILTs based cancer immunotherapy.
Heterogeneity of HLA-G Expression in Malignancies
Accumulating evidence solidify the concept that tumor heterogeneity, including inter-patient, inter-tumor, and intra-tumor heterogeneity, is the main cause for variable responses and clinical outcomes to anti-cancer therapy (101). Since the first report HLA-G expressed in tumors, the degree or proportion of HLA-G expression in thousands of malignant lesions among over thirty different types of cancers have been explored. Data revealed that HLA-G expression is restricted to malignant lesions, but not in adjacent non-tumorous tissues, and that neo-HLA-G expression is strongly related to metastasis, advanced disease stage, poor prognosis, and clinical outcome (89). However, inter-patient, inter-tumor, and intra-tumor heterogeneity of the HLA-G expression in each histopathological type of malignancies is also evident (102, 103). In addition to the HLA-G heterogeneity caused by tumor cell itself, such as clonal growth with genetic alterations, epigenetic and post-translational modifications, explanation of HLA-G expression could be biased due to usage of different current available anti-HLA-G monoclonal antibodies, and different assay protocols (62, 96). Very recently, using the method of flow cytometry, our data showed that HLA-G expression in 157 epithelial cell adhesion molecule (EpCAM) positive-gated colorectal tumor lesions is with a median of 14.90% (range: 1.81~79.90%) (24).
For an example, the inter-patient proportion of HLA-G expression in cancers has been observed from 24 to 94.1% in breast cancers (12, 14), and from 22.1 to 70.7% in colorectal cancers (19, 20). Noteworthy, only few studies on the intrapatient inter-tumor and intra-tumor heterogeneity of the HLA-G expression were available. In a cohort of 136 primary cervical cancers, inter-tumor heterogeneity of the HLA-G expression was detected in 25% of these lesions and 11% of case-matched lymph node (LN) metastases. Among pathological subtypes, HLA-G was positive in 22% in squamous cell carcinoma lesions and 20% in LN metastatic tissues, 31% in adenocarcinoma lesions, and 28.0% in their LN metastatic samples. In patients with invasive cervical cancer, positive proportion of HLA-G expression was found in 31.6 and 29.6% of the primary and LN metastatic lesions, respectively (104). Similarly, a study by Swets et al. (16) showed that HLA-G expression was found in 29% of the primary colorectal cancer and 30% of the corresponding liver metastases. Finally, regarding the intra-tumor heterogeneity of the HLA-G, Rouas-Freiss et al. (103) reported that, among 19 clear cell renal-cell carcinoma lesions, the proportion of HLA-G expression varies dramatically on CA9+ clear cell renal-cell carcinoma cells in different zones in each sample, which could be ranged from negative to almost totally positive for HLA-G expression. Also with clear cell renal-cell carcinoma lesions, a study by Tronik-Le Roux et al. (79) released that HLA-G isoform expression including HLA-G1, -G5, and HLA-G6 are extremely heterogeneous among distinct subcellular locations and zones within a same tumor. In a serial section study with colorectal and esophageal cancer lesions, our recent findings further revealed that intratumor heterogeneous expression of HLA-G is a very frequent phenomenon among different zones within a tumor (102).
Native HLA-G Isoform Expression in Cancer Lesions Needs Evaluated
As aforementioned, most currently available information of HLA-G expression in cancer lesions were evaluated by immunohistochemistry with the mAbs 4H84 and/or 5A6G7, which represents all α1 domain containing HLA-G or HLA-G5/6 isoform expression. Whether these data are consistent with the levels of tumor cell surface HLA-G expression remain elusive. Previous evidence showed that no correlation has been established between the degree of cancer lesion HLA-G5/6 expression evaluated with immunohistochemistry and peripheral soluble HLA-G levels (42). Given the fact that HLA-G/ILTs interaction is conformation dependent, tumor cell surface native or conformational HLA-G expression be evaluated with assays such as flow cytometry is necessary (105). To address this issue, more reliable and specific anti-native or -conformational HLA-G mAbs are yet to be explored.
Other HLA-G Receptors
In addition to the ILT-2 and ILT4, receptors including killer inhibitory receptor (KIR) 2DL4/CD158d, CD8, CD160 could also bind HLA-G (106). To be noted, NKG2A/CD94 has been recently reported which could bind to allelic specific products of HLA-G (107).
KIR2DL4 is a member of the killer cell immunoglobulin (Ig)-like receptor (KIR) family, but with an atypical feature owing to a D0 and D2 hybrid extracellular domain, a positively charged arginine residue in the transmembrane region and one ITIM domain in its cytoplasmatic tail. The charged arginine residue enables KIR2DL4 to associate with the Fc fragment receptor γ (FcRγ), which contains two cytoplasmic immunoreceptor tyrosine-based activation motifs (ITAMs). With this unique structure, KIR2DL4 is of both the activation (ITAM) and inhibitory (ITIM) signaling domains (108). The biological function of KIR2DL4 and HLA-G binding is to work as an activating receptor, which induces strong pro-inflammatory cytokine and chemokine immune responses through the endosomal DNA-dependent protein kinase (DNA-PKcs) signaling pathway, but not the NK cell cytotoxicity (109). KIR2DL4 is predominately expressed in decidual NK cells and HLA-G/KIR2DL4 interaction plays critical roles in the regulation of maternal-fetal immune microenvironment, spiralartery remodeling and fetal growth (4).
The heterodimer NKG2A/CD94, a member of the C-type lectin-like receptor family, is a well-known immune inhibitory receptor for HLA-E binding. NKG2A/CD94 is mainly expressed on CD8+ T lymphocytes and subsets of NK cells (110). Additionally, NKG2A/CD94 has recently been found to be an HLA-G allelic product dependent receptor. Hò et al. (107) revealed that a remarkably higher binding affinity was observed for the HLA-G*01:04/NKG2A/CD94 interaction than those for the HLA-G*01:03/NKG2A/CD94 and HLA-G*01:01/NKG2A/CD94 interactions. Moreover, no engagement was observed between the activating receptor NKG2C/CD94 and HLA-G. HLA-G allelic product dependent binding of NKG2A/CD94 could result from the single amino acid residue in the HLA-G*01:01 heavy chain differing from HLA-G*01:04 (p.110L > I) and HLA-G*01:03 (p.31T > S), and these different residues may affect the peptide repertoire and receptor recognition (111). Consequently, different biological function modulation could be expected for the different allelic HLA-G molecules on the immune cells expressing NKG2A/CD94.
Other receptors are the glycosylphosphatidylinositol-anchored receptor CD160, which is expressed on activated endothelial cells, CD16+CD56dim NK cells, and CD8+ T cells (112, 113). CD160 signaling depends on adapter proteins, such as the phosphoinositide-3 kinase, to activate endothelial cell migration and angiogenesis, and immune cells, such as cytokine releasing and target cell lyses (112, 114). Moreover, the cytotoxic T cell surface marker CD8 is reported to interact with the HLA-G protein, which can induce apoptosis of the CD8+ T cells and CD56+CD8+ NKT cells through the Fas/FasL pathway (115).
Conclusions and Perspectives
In most scenarios, HLA-G/ILTs interaction promotes cancer cells to escape immune surveillance and anti-tumor immunity (97, 116). Interference or blockade of HLA-G/ILTs interaction can restore host anti-tumor immune responses, which providing a strong rationale and opportunities to develop HLA-G/ILTs-targeted ICIs for solid cancer immunotherapy. Indeed, early clinical trials based on this immune checkpoint is being launched for different advanced solid cancer treatment2. However, many challenges remain to be addressed.
First, multiple identified HLA-G isoforms and more can be expected, which have distinct extracellular domain(s) for each of them. This information indicates that biological function of different isoforms is diverse. Being lack of isoform-specific antibodies, their tumor tissue expression characteristics and clinical significance is unclear. Currently available mAb 4H84, which probes all isoforms containing α1 domain, can’t distinguish either a distinct isoform or combination of different isoforms expressed on tumor tissues. Novel isoforms without α1 domain can’t be detected with any available mAbs which can be ignored in tumor tissues. These findings sharply compounded the clinical relevance of HLA-G in tumor patients (62). Second, being lack of international validated or recommended assay protocols, both performance and cut-off points are diverse and unconcordance in interpretation of HLA-G expression across studies is rather common (117). Third, being the landscape of HLA-G isoform expression in tumor tissues can’t be specified, application of HLA-G/ILTs-targeted ICIs for cancer treatment can be aimless. Furthermore, HLA-G/ILTs engagement is isoform dependent, where HLA-G1 and HLA-G5 binds ILT-2, and HLA-G1, -G2, -G4, -G5, and HLA-G6 binds ILT-4 (118). Differential expression of HLA-G isoforms does exist in tumor cells whereas the underlying mechanisms are yet to be explored. In this scenario, patient individualized landscape and degree of HLA-G isoform expression should be defined before therapy due to which can tremendously affect clinical benefits of HLA-G-based solid cancer immunotherapy.
In summary, exploration of more reliable and HLA-G isoform-specific antibodies, implementation of international community validated HLA-G detection protocols, deeper insight of patient individualized landscape of HLA-G expression, and development of isoform matched both HLA-G and ILT-2/-4 blocking antibodies, are future directions warranted for the HLA-G/ILTs-targeted solid cancer immunotherapy. Despite these challenges, owing to HLA-G expression is restrict to malignant tissues, and HLA-G/ILTs signaling is involved in a broader spectrum of immune responses than CTLA-4/B7 and PD-1/PD-L1 does, the clinical effects of the immune checkpoint HLA-G/ILTs is optimistic.
Author Contributions
Conceptualization, data curation, funding acquisition, writing —original draft, review and editing: W-HY and AL. Both authors contributed to the article and approved the submitted version.
Funding
This work was supported by grants from Science and Technology Bureau of Taizhou (1901ky01; 1901ky04).
Conflict of Interest
The authors declare that the research was conducted in the absence of any commercial or financial relationships that could be construed as a potential conflict of interest.
Supplementary Material
The Supplementary Material for this article can be found online at: https://www.frontiersin.org/articles/10.3389/fimmu.2021.698677/full#supplementary-material
Supplementary Figure 1 | CLUSTALO sequence alignment either among or between full-length HLA-G, HLA-F, and HLA-E molecules. Results of sequence alignment (A) among full length of HLA-G, HLA-F, and HLA-E molecules. (B) Between full length of HLA-G and HLA-F molecules. (C) Between full length of HLA-E and HLA-F molecules. (D) Between full length of HLA-E and HLA-G molecules.
References
1. Morganti S, Curigliano G. Combinations Using Checkpoint Blockade to Overcome Resistance. Ecancermedicalscience (2020) 14:1148. doi: 10.3332/ecancer.2020.1148
2. Gilead Buys Into Tizona’s Anti-HLA-G Strategy. Cancer Discov (2020) 10:1433. doi: 10.1158/2159-8290.CD-NB2020-077
3. Kovats S, Main EK, Librach C, Stubblebine M, Fisher SJ, DeMars R. A Class I Antigen, HLA-G, Expressed in Human Trophoblasts. Science (1990) 248:220–3. doi: 10.1126/science.2326636
4. Xu X, Zhou Y, Wei H. Roles of HLA-G in the Maternal-Fetal Immune Microenvironment. Front Immunol (2020) 11:592010. doi: 10.3389/fimmu.2020.592010
5. Paul P, Rouas-Freiss N, Khalil-Daher I, Moreau P, Riteau B, Le Gal FA, et al. HLA-G Expression in Melanoma: A Way for Tumor Cells to Escape From Immunosurveillance. Proc Natl Acad Sci USA (1998) 95:4510–5. doi: 10.1073/pnas.95.8.4510
6. Kleinberg L, Flørenes VA, Skrede M, Dong HP, Nielsen S, McMaster MT, et al. Expression of HLA-G in Malignant Mesothelioma and Clinically Aggressive Breast Carcinoma. Virchows Arch (2006) 449:31–9. doi: 10.1007/s00428-005-0144-7
7. Chen HX, Lin A, Shen CJ, Zhen R, Chen BG, Zhang X, et al. Upregulation of Human Leukocyte Antigen-G Expression and Its Clinical Significance in Ductal Breast Cancer. Hum Immunol (2010) 71:892–8. doi: 10.1016/j.humimm.2010.06.009
8. He X, Dong DD, Yie SM, Yang H, Cao M, Ye SR, et al. HLA-G Expression in Human Breast Cancer: Implications for Diagnosis and Prognosis, and Effect on Allocytotoxic Lymphocyte Response After Hormone Treatment In Vitro. Ann Surg Oncol (2010) 17:1459–69. doi: 10.1245/s10434-009-0891-9
9. de Kruijf EM, Sajet A, van Nes JG, Natanov R, Putter H, Smit VT, et al. HLA-E and HLA-G Expression in Classical HLA Class I-Negative Tumors Is of Prognostic Value for Clinical Outcome of Early Breast Cancer Patients. J Immunol (2010) 185:7452–9. doi: 10.4049/jimmunol.1002629
10. da Silva GB, Silva TG, Duarte RA, Neto NL, Carrara HH, Donadi EA, et al. Expression of the Classical and Nonclassical Hla Molecules in Breast Cancer. Int J Breast Cancer (2013) 2013:250435. doi: 10.1155/2013/250435
11. Ramos CS, Gonçalves AS, Marinho LC, Gomes Avelino MA, Saddi VA, Lopes AC, et al. Analysis of HLA-G Gene Polymorphism and Protein Expression in Invasive Breast Ductal Carcinoma. Hum Immunol (2014) 75:667–72. doi: 10.1016/j.humimm.2014.04.005
12. Ishibashi K, Kumai T, Ohkuri T, Kosaka A, Nagato T, Hirata Y, et al. Epigenetic Modification Augments the Immunogenicity of Human Leukocyte Antigen G Serving as a Tumor Antigen for T Cell-Based Immunotherapy. Oncoimmunology (2016) 5:e1169356. doi: 10.1080/2162402X.2016.1169356
13. Jeong S, Park S, Park BW, Park Y, Kwon OJ, Kim HS. Human Leukocyte Antigen-G (HLA-G) Polymorphism and Expression in Breast Cancer Patients. PLoS One (2014) 9:e98284. doi: 10.1371/journal.pone.0098284
14. Engels CC, Charehbili A, van de Velde CJ, Bastiaannet E, Sajet A, Putter H, et al. The Prognostic and Predictive Value of Tregs and Tumor Immune Subtypes in Postmenopausal, Hormone Receptor-Positive Breast Cancer Patients Treated With Adjuvant Endocrine Therapy: A Dutch TEAM Study Analysis. Breast Cancer Res Treat (2015) 149:587–96. doi: 10.1007/s10549-015-3269-7
15. Wuerfel FM, Huebner H, Häberle L, Gass P, Hein A, Jud SM, et al. HLA-G and HLA-F Protein Isoform Expression in Breast Cancer Patients Receiving Neoadjuvant Treatment. Sci Rep (2020) 10:15750. doi: 10.1038/s41598-020-72837-3
16. Swets M, König MH, Zaalberg A, Dekker-Ensink NG, Gelderblom H, van de Velde CJ, et al. HLA-G and Classical HLA Class I Expression in Primary Colorectal Cancer and Associated Liver Metastases. Hum Immunol (2016) 77:773–9. doi: 10.1016/j.humimm.2016.03.001
17. Ye SR, Yang H, Li K, Dong DD, Lin XM, Yie SM. Human Leukocyte Antigen G Expression: As a Significant Prognostic Indicator for Patients With Colorectal Cancer. Mod Pathol (2007) 20:375–83. doi: 10.1038/modpathol.3800751
18. Guo ZY, Lv YG, Wang L, Shi SJ, Yang F, Zheng GX, et al. Predictive Value of HLA-G and HLA-E in the Prognosis of Colorectal Cancer Patients. Cell Immunol (2015) 293:10–6. doi: 10.1016/j.cellimm.2014.10.003
19. Zhang RL, Zhang X, Dong SS, Hu B, Han QY, Zhang JG, et al. Predictive Value of Different Proportion of Lesion HLA-G Expression in Colorectal Cancer. Oncotarget (2017) 8:107441–51. doi: 10.18632/oncotarget.22487
20. Zeestraten EC, Reimers MS, Saadatmand S, Goossens-Beumer IJ, Dekker JW, Liefers GJ, et al. Combined Analysis of HLA-E and HLA-G Predicts Prognosis in Colon Cancer Patients. Br J Cancer (2014) 110:459–68. doi: 10.1038/bjc.2013.696
21. Reimers MS, Engels CC, Putter H, Morreau H, Liefers GJ, van de Velde CJ, et al. Prognostic Value of Hla-E, HLA-G and Tregs in Rectal Cancer: A Retrospective Cohort Study. BMC Cancer (2014) 14:486. doi: 10.1186/1471-2407-14-486
22. Cai Z, Wang L, Han Y, Gao W, Wei X, Gong R, et al. Immunoglobulin-Like Transcript 4 and Human Leukocyte Antigen-G Interaction Promotes the Progression of Human Colorectal Cancer. Int J Oncol (2019) 54:1943–54. doi: 10.3892/ijo.2019.4761
23. Lin A, Zhang X, Zhang RL, Zhang JG, Zhou WJ, Yan WH. Clinical Significance of Potential Unidentified HLA-G Isoforms Without α1 Domain But Containing Intron 4 in Colorectal Cancer Patients. Front Oncol (2018) 8:361. doi: 10.3389/fonc.2018.00361
24. Chen QY, Chen YX, Han QY, Zhang JG, Zhou WJ, Zhang X, et al. Prognositic Significance of Immune Checkpoints HLA-G/ILT-2/4 and PD-L1 in Colorectal Cancer. Front Immunol (2021) 12:679090. doi: 10.3389/fimmu.2021.679090
25. Miranda LN, Reginaldo FP, Souza DM, Soares CP, Silva TG, Rocha KB, et al. Greater Expression of the Human Leukocyte Antigen-G (HLA-G) and interleukin-17 (Il-17) in Cervical Intraepithelial Neoplasia: Analytical Cross-Sectional Study. Sao Paulo Med J (2015) 133:336–42. doi: 10.1590/1516-3180.2013.7170009
26. Li XJ, Zhang X, Lin A, Ruan YY, Yan WH. Human Leukocyte Antigen-G (HLA-G) Expression in Cervical Cancer Lesions Is Associated With Disease Progression. Hum Immunol (2012) 73:946–9. doi: 10.1016/j.humimm.2012.07.041
27. Guimarães MC, Soares CP, Donadi EA, Derchain SF, Andrade LA, Silva TG, et al. Low Expression of Human Histocompatibility Soluble Leukocyte Antigen-G (HLA-G5) in Invasive Cervical Cancer With and Without Metastasis, Associated With Papilloma Virus (HPV). J Histochem Cytochem (2010) 58:405–11. doi: 10.1369/jhc.2009.954131
28. Barrier BF, Kendall BS, Sharpe-Timms KL, Kost ER. Characterization of Human Leukocyte Antigen-G (HLA-G) Expression in Endometrial Adenocarcinoma. Gynecol Oncol (2006) 103:25–30. doi: 10.1016/j.ygyno.2006.01.045
29. Bijen CB, Bantema-Joppe EJ, de Jong RA, Leffers N, Mourits MJ, Eggink HF, et al. The Prognostic Role of Classical and Nonclassical MHC Class I Expression in Endometrial Cancer. Int J Cancer (2010) 126:1417–27. doi: 10.1002/ijc.24852
30. Ben Yahia H, Boujelbene N, Babay W, Ben Safta I, Dhouioui S, Zemni I, et al. Expression Analysis of Immune-Regulatory Molecules HLA-G, HLA-E and IDO in Endometrial Cancer. Hum Immunol (2020) 81:305–13. doi: 10.1016/j.humimm.2020.03.008
31. Walentowicz-Sadlecka M, Dziobek K, Grabiec M, Sadlecki P, Walentowicz P, Mak P, et al. The Analysis of Human Leukocyte Antigen-G Level in Patients With Endometrial Cancer by Western Blot Technique. Am J Reprod Immunol (2019) 81:e13070. doi: 10.1111/aji.13070
32. Yie SM, Yang H, Ye SR, Li K, Dong DD, Lin XM. Expression of HLA-G Is Associated With Prognosis in Esophageal Squamous Cell Carcinoma. Am J Clin Pathol (2007) 128:1002–9. doi: 10.1309/JNCW1QLDFB6AM9WE
33. Lin A, Zhang X, Zhou WJ, Ruan YY, Xu DP, Wang Q, et al. Human Leukocyte Antigen-G Expression Is Associated With a Poor Prognosis in Patients With Esophageal Squamous Cell Carcinoma. Int J Cancer (2011) 129:1382–90. doi: 10.1002/ijc.25807
34. Zheng J, Xu C, Chu D, Zhang X, Li J, Ji G, et al. Human Leukocyte Antigen G Is Associated With Esophageal Squamous Cell Carcinoma Progression and Poor Prognosis. Immunol Lett (2014) 161:13–9. doi: 10.1016/j.imlet.2014.04.007
35. Spurny C, Kailayangiri S, Altvater B, Jamitzky S, Hartmann W, Wardelmann E, et al. T Cell Infiltration Into Ewing Sarcomas Is Associated With Local Expression of Immune-Inhibitory HLA-G. Oncotarget (2017) 9:6536–49. doi: 10.18632/oncotarget.23815
36. Yie SM, Yang H, Ye SR, Li K, Dong DD, Lin XM. Expression of Human Leukocyte Antigen G (Hla-G) Correlates With Poor Prognosis in Gastric Carcinoma. Ann Surg Oncol (2007) 14:2721–9. doi: 10.1245/s10434-007-9464-y
37. Tuncel T, Karagoz B, Haholu A, Ozgun A, Emirzeoglu L, Bilgi O, et al. Immunoregulatory Function of HLA-G in Gastric Cancer. Asian Pac J Cancer Prev (2013) 14:7681–4. doi: 10.7314/apjcp.2013.14.12.7681
38. Du L, Xiao X, Wang C, Zhang X, Zheng N, Wang L, et al. Human Leukocyte Antigen-G Is Closely Associated With Tumor Immune Escape in Gastric Cancer by Increasing Local Regulatory T Cells. Cancer Sci (2011) 102:1272–80. doi: 10.1111/j.1349-7006.2011.01951.x
39. Wastowski IJ, Simões RT, Yaghi L, Donadi EA, Pancoto JT, Poras I, et al. Human Leukocyte Antigen-G Is Frequently Expressed in Glioblastoma and may be Induced In Vitro by Combined 5-Aza-2’-Deoxycytidine and Interferon-γ Treatments: Results From a Multicentric Study. Am J Pathol (2013) 182:540–52. doi: 10.1016/j.ajpath.2012.10.021
40. Cai MY, Xu YF, Qiu SJ, Ju MJ, Gao Q, Li YW, et al. Human Leukocyte Antigen-G Protein Expression Is an Unfavorable Prognostic Predictor of Hepatocellular Carcinoma Following Curative Resection. Clin Cancer Res (2009) 15:4686–93. doi: 10.1158/1078-0432.CCR-09-0463
41. Wang Y, Ye Z, Meng XQ, Zheng SS. Expression of HLA-G in Patients With Hepatocellular Carcinoma. Hepatobiliary Pancreat Dis Int (2011) 10:158–63. doi: 10.1016/s1499-3872(11)60025-8
42. Lin A, Chen HX, Zhu CC, Zhang X, Xu HH, Zhang JG, et al. Aberrant Human Leucocyte Antigen-G Expression and Its Clinical Relevance in Hepatocellular Carcinoma. J Cell Mol Med (2010) 14:2162–71. doi: 10.1111/j.1582-4934.2009.00917.x
43. Yie SM, Yang H, Ye SR, Li K, Dong DD, Lin XM. Expression of Human Leucocyte Antigen G (Hla-G) Is Associated With Prognosis in non-Small Cell Lung Cancer. Lung Cancer (2007) 58:267–74. doi: 10.1016/j.lungcan.2007.06.011
44. Lin A, Zhu CC, Chen HX, Chen BF, Zhang X, Zhang JG, et al. Clinical Relevance and Functional Implications for Human Leucocyte Antigen-G Expression in Non-Small-Cell Lung Cancer. J Cell Mol Med (2010) 14:2318–29. doi: 10.1111/j.1582-4934.2009.00858.x
45. Yan WH, Liu D, Lu HY, Li YY, Zhang X, Lin A. Significance of Tumour Cell HLA-G5/-G6 Isoform Expression in Discrimination for Adenocarcinoma From Squamous Cell Carcinoma in Lung Cancer Patients. J Cell Mol Med (2015) 19:778–85. doi: 10.1111/jcmm.12400
46. Diepstra A, Poppema S, Boot M, Visser L, Nolte IM, Niens M, et al. HLA-G Protein Expression as a Potential Immune Escape Mechanism in Classical Hodgkin’s Lymphoma. Tissue Antigens (2008) 71:219–26. doi: 10.1111/j.1399-0039.2008.01005.x
47. Caocci G, Greco M, Fanni D, Senes G, Littera R, Lai S, et al. HLA-G Expression and Role in Advanced-Stage Classical Hodgkin Lymphoma. Eur J Histochem (2016) 60:2606. doi: 10.4081/ejh.2016.2606
48. Jesionek-Kupnicka D, Bojo M, Prochorec-Sobieszek M, Szumera-Ciećkiewicz A, Jabłońska J, Kalinka-Warzocha E, et al. HLA-G and MHC Class II Protein Expression in Diffuse Large B-Cell Lymphoma. Arch Immunol Ther Exp (Warsz) (2016) 64:225–40. doi: 10.1007/s00005-015-0372-8
49. Urosevic M, Willers J, Mueller B, Kempf W, Burg G, Dummer R. HLA-G Protein Up-Regulation in Primary Cutaneous Lymphomas Is Associated With Interleukin-10 Expression in Large Cell T-Cell Lymphomas and Indolent B-Cell Lymphomas. Blood (2002) 99:609–17. doi: 10.1182/blood.v99.2.609
50. Cai MB, Han HQ, Bei JX, Liu CC, Lei JJ, Cui Q, et al. Expression of Human Leukocyte Antigen G Is Associated With Prognosis in Nasopharyngeal Carcinoma. Int J Biol Sci (2012) 8:891–900. doi: 10.7150/ijbs.4383
51. Gonçalves AS, Wastowski IJ, Capeletti LR, Sacono NT, Cortez AP, Valadares MC, et al. The Clinicopathologic Significance of the Expression of HLA-G in Oral Squamous Cell Carcinoma. Oral Surg Oral Med Oral Pathol Oral Radiol (2014) 117:361–8. doi: 10.1016/j.oooo.2013.12.001
52. Jung YW, Kim YT, Kim SW, Kim S, Kim JH, Cho NH, et al. Correlation of Human Leukocyte Antigen-G (HLA-G) Expression and Disease Progression in Epithelial Ovarian Cancer. Reprod Sci (2009) 16:1103–11. doi: 10.1177/1933719109342131
53. Menier C, Prevot S, Carosella ED, Rouas-Freiss N. Human Leukocyte Antigen-G Is Expressed in Advanced-Stage Ovarian Carcinoma of High-Grade Histology. Hum Immunol (2009) 70:1006–9. doi: 10.1016/j.humimm.2009.07.021
54. Zhang X, Han QY, Li JB, Ruan YY, Yan WH, Lin A. Lesion HLA-G5/-G6 Isoforms Expression in Patients With Ovarian Cancer. Hum Immunol (2016) 77:780–4. doi: 10.1016/j.humimm.2015.12.003
55. Babay W, Ben Yahia H, Boujelbene N, Zidi N, Laaribi AB, Kacem D, et al. Clinicopathologic Significance of HLA-G and HLA-E Molecules in Tunisian Patients With Ovarian Carcinoma. Hum Immunol (2018) 79:463–70. doi: 10.1016/j.humimm.2018.02.012
56. Xu YF, Lu Y, Cheng H, Jiang J, Xu J, Long J, et al. High Expression of Human Leukocyte Antigen-G Is Associated With a Poor Prognosis in Patients With PDAC. Curr Mol Med (2015) 15:360–7. doi: 10.2174/1566524015666150401102218
57. Khodabandeh Shahraki P, Zare Y, Azarpira N, Hosseinzadeh M, Farjadian S. Prognostic Value of HLA-G in Malignant Liver and Pancreas Lesions. Iran J Immunol (2018) 15:28–37.
58. Zhou L, Niu ZY, Liang ZY, Zhou WX, You L, Wang MY, et al. HLA-G Impairs Host Immune Response and Predicts Poor Prognosis in Pancreatic Cancer. Am J Transl Res (2015) 7:2036–44.
59. Hiraoka N, Ino Y, Hori S, Yamazaki-Itoh R, Naito C, Shimasaki M, et al. Expression of Classical Human Leukocyte Antigen Class I Antigens, HLA-E and HLA-G, Is Adversely Prognostic in Pancreatic Cancer Patients. Cancer Sci (2020) 111:3057–70. doi: 10.1111/cas.14514
60. de Figueiredo Feitosa NL, Crispim JC, Zanetti BR, Magalhães PK, Soares CP, Soares EG, et al. HLA-G Is Differentially Expressed in Thyroid Tissues. Thyroid (2014) 24:585–92. doi: 10.1089/thy.2013.0246
61. Nunes LM, Ayres FM, Francescantonio IC, Saddi VA, Avelino MA, Alencar Rde C, et al. Association Between the HLA-G Molecule and Lymph Node Metastasis in Papillary Thyroid Cancer. Hum Immunol (2013) 74:447–51. doi: 10.1016/j.humimm.2012.12.012
62. Lin A, Yan WH. Heterogeneity of HLA-G Expression in Cancers: Facing the Challenges. Front Immunol (2018) 9:2164. doi: 10.3389/fimmu.2018.02164
63. Lin A, Yan WH. Intercellular Transfer of HLA-G: Its Potential in Cancer Immunology. Clin Transl Immunol (2019) 8:e1077. doi: 10.1002/cti2.1077
64. Svajger U, Obermajer N, Jeras M. IFN-γ-Rich Environment Programs Dendritic Cells Toward Silencing of Cytotoxic Immune Responses. J Leukoc Biol (2014) 95:33–46. doi: 10.1189/jlb.1112589
65. Saurabh A, Chakraborty S, Kumar P, Mohan A, Bhatnagar AK, Rishi N, et al. Inhibiting HLA-G Restores IFN-γ and TNF-α Producing T Cell in Pleural Tuberculosis. Tuberculosis (Edinb) (2018) 109:69–79. doi: 10.1016/j.tube.2018.01.008
66. Morandi F, Ferretti E, Bocca P, Prigione I, Raffaghello L, Pistoia V. A Novel Mechanism of Soluble HLA-G Mediated Immune Modulation: Downregulation of T Cell Chemokine Receptor Expression and Impairment of Chemotaxis. PLoS One (2010) 5:e11763. doi: 10.1371/journal.pone.0011763
67. Morandi F, Ferretti E, Castriconi R, Dondero A, Petretto A, Bottino C, et al. Soluble HLA-G Dampens CD94/NKG2A Expression and Function and Differentially Modulates Chemotaxis and Cytokine and Chemokine Secretion in CD56bright and CD56dim NK Cells. Blood (2011) 118:5840–50. doi: 10.1182/blood-2011-05-352393
68. Naji A, Menier C, Morandi F, Agaugué S, Maki G, Ferretti E, et al. Binding of HLA-G to ITIM-Bearing Ig-Like Transcript 2 Receptor Suppresses B Cell Responses. J Immunol (2014) 192:1536–46. doi: 10.4049/jimmunol.1300438
69. Liang S, Ristich V, Arase H, Dausset J, Carosella ED, Horuzsko A. Modulation of Dendritic Cell Differentiation by HLA-G and ILT4 Requires the IL-6–STAT3 Signaling Pathway. Proc Natl Acad Sci USA (2008) 105:8357–62. doi: 10.1073/pnas.0803341105
70. Wu CL, Caumartin J, Amodio G, Anna F, Loustau M, Gregori S, et al. Inhibition of Inkt Cells by the HLA-G-ILT2 Checkpoint and Poor Stimulation by HLA-G-Expressing Tolerogenic Dc. Front Immunol (2021) 11:608614. doi: 10.3389/fimmu.2020.608614
71. Dumont C, Jacquier A, Verine J, Noel F, Goujon A, Wu CL, et al. Cd8+Pd-1-Ilt2+ T Cells Are an Intratumoral Cytotoxic Population Selectively Inhibited by the Immune-Checkpoint Hla-G. Cancer Immunol Res (2019) 7:1619–32. doi: 10.1158/2326-6066.CIR-18-0764
72. Köstlin N, Ostermeir AL, Spring B, Schwarz J, Marmé A, Walter CB, et al. HLA-G Promotes Myeloid-Derived Suppressor Cell Accumulation and Suppressive Activity During Human Pregnancy Through Engagement of the Receptor ILT4. Eur J Immunol (2017) 47:374–84. doi: 10.1002/eji.201646564
73. Gregori S, Tomasoni D, Pacciani V, Scirpoli M, Battaglia M, Magnani CF, et al. Differentiation of Type 1 T Regulatory Cells (Tr1) by Tolerogenic DC-10 Requires the IL-10-Dependent ILT4/HLA-G Pathway. Blood (2010) 116:935–44. doi: 10.1182/blood-2009-07-234872
74. Lin A, Xu HH, Xu DP, Zhang X, Wang Q, Yan WH. Multiple Steps of HLA-G in Ovarian Carcinoma Metastasis: Alter NK Cytotoxicity and Induce Matrix Metalloproteinase-15 (Mmp-15) Expression. Hum Immunol (2013) 74:439–46. doi: 10.1016/j.humimm.2012.11.021
75. Polakova K, Bandzuchova E. Comparison of HLA-G and MMP Transcription in Human Tumor Cell Lines. Neoplasma (2018) 65:943–51. doi: 10.4149/neo_2018_180409N230
76. Ishitani A, Geraghty DE. Alternative Splicing of HLA-G Transcripts Yields Proteins With Primary Structures Resembling Both Class I and Class II Antigens. Proc Natl Acad Sci USA (1992) 89:3947–51. doi: 10.1073/pnas.89.9.3947
77. Fujii T, Ishitani A, Geraghty DE. A Soluble Form of the HLA-G Antigen Is Encoded by a Messenger Ribonucleic Acid Containing Intron 4. J Immunol (1994) 153:5516–24.
78. Paul P, Cabestre FA, Ibrahim EC, Lefebvre S, Khalil-Daher I, Vazeux G, et al. Identification of HLA-G7 as a New Splice Variant of the HLA-G mRNA and Expression of Soluble HLA-G5, -G6, and -G7 Transcripts in Human Transfected Cells. Hum Immunol (2000) 61:1138–49. doi: 10.1016/s0198-8859(00)00197-x
79. Tronik-Le Roux D, Renard J, Vérine J, Renault V, Tubacher E, LeMaoult J, et al. Novel Landscape of HLA-G Isoforms Expressed in Clear Cell Renal Cell Carcinoma Patients. Mol Oncol (2017) 11:1561–78. doi: 10.1002/1878-0261.12119
80. Nardi Fda S, König L, Wagner B, Giebel B, Santos Manvailer LF, Rebmann V. Soluble Monomers, Dimers and HLA-G-Expressing Extracellular Vesicles: The Three Dimensions of Structural Complexity to Use HLA-G as a Clinical Biomarker. HLA (2016) 88:77–86. doi: 10.1111/tan.12844
81. McMaster M, Zhou Y, Shorter S, Kapasi K, Geraghty D, Lim KH, et al. HLA-G Isoforms Produced by Placental Cytotrophoblasts and Found in Amniotic Fluid Are Due to Unusual Glycosylation. J Immunol (1998) 160:5922–8.
82. Díaz-Lagares A, Alegre E, Gonzalez A. Detection of 3-Nitrotyrosine-Modified Human Leukocyte Antigen-G in Biological Fluids. Hum Immunol (2009) 70:976–80. doi: 10.1016/j.humimm.2009.07.018
83. Alegre E, Rebmann V, Lemaoult J, Rodriguez C, Horn PA, Díaz-Lagares A, et al. In Vivo Identification of an HLA-G Complex as Ubiquitinated Protein Circulating in Exosomes. Eur J Immunol (2013) 43:1933–9. doi: 10.1002/eji.201343318
84. Kang X, Kim J, Deng M, John S, Chen H, Wu G, et al. Inhibitory Leukocyte Immunoglobulin-Like Receptors: Immune Checkpoint Proteins and Tumor Sustaining Factors. Cell Cycle (2016) 15:25–40. doi: 10.1080/15384101.2015.1121324
85. Borrego F, Kabat J, Kim DK, Lieto L, Maasho K, Peña J, et al. Structure and Function of Major Histocompatibility Complex (MHC) Class I Specific Receptors Expressed on Human Natural Killer (NK) Cells. Mol Immunol (2002) 38:637–60. doi: 10.1016/s0161-5890(01)00107-9
86. Wang Q, Song H, Cheng H, Qi J, Nam G, Tan S, et al. Structures of the Four Ig-Like Domain LILRB2 and the Four-Domain LILRB1 and HLA-G1 Complex. Cell Mol Immunol (2020) 17:966–75. doi: 10.1038/s41423-019-0258-5
87. Shiroishi M, Kuroki K, Rasubala L, Tsumoto K, Kumagai I, Kurimoto E, et al. Structural Basis for Recognition of the Nonclassical MHC Molecule HLA-G by the Leukocyte Ig-Like Receptor B2 (Lilrb2/Lir2/Ilt4/Cd85d). Proc Natl Acad Sci USA (2006) 103:16412–7. doi: 10.1073/pnas.0605228103
88. Shiroishi M, Tsumoto K, Amano K, Shirakihara Y, Colonna M, Braud VM, et al. Human Inhibitory Receptors Ig-Like Transcript 2 (ILT2) and ILT4 Compete With CD8 for MHC Class I Binding and Bind Preferentially to HLA-G. Proc Natl Acad Sci USA (2003) 100:8856–61. doi: 10.1073/pnas.1431057100
89. Carosella ED, Rouas-Freiss N, Tronik-Le Roux D, Moreau P, LeMaoult J. Hla-G: An Immune Checkpoint Molecule. Adv Immunol (2015) 127:33–144. doi: 10.1016/bs.ai.2015.04.001
90. Würfel FM, Winterhalter C, Trenkwalder P, Wirtz RM, Würfel W. European Patent in Immunoncology: From Immunological Principles of Implantation to Cancer Treatment. Int J Mol Sci (2019) 20:1830. doi: 10.3390/ijms20081830
91. Hofmeister V, Weiss EH. HLA-G Modulates Immune Responses by Diverse Receptor Interactions. Semin Cancer Biol (2003) 13:317–23. doi: 10.1016/s1044-579x(03)00022-1
92. Seliger B. Molecular Mechanisms of HLA Class I-Mediated Immune Evasion of Human Tumors and Their Role in Resistance to Immunotherapies. HLA (2016) 88:213–20. doi: 10.1111/tan.12898
93. Apps R, Gardner L, Moffett A. A Critical Look at HLA-G. Trends Immunol (2008) 29:313–21. doi: 10.1016/j.it.2008.02.012
94. Sernee MF, Ploegh HL, Schust DJ. Why Certain Antibodies Cross-React With HLA-A and HLA-G: Epitope Mapping of Two Common MHC Class I Reagents. Mol Immunol (1998) 35:177–88. doi: 10.1016/s0161-5890(98)00026-1
95. Poláková K, Kuba D, Russ G. The 4H84 Monoclonal Antibody Detecting Beta2m Free Nonclassical HLA-G Molecules Also Binds to Free Heavy Chains of Classical HLA Class I Antigens Present on Activated Lymphocytes. Hum Immunol (2004) 65:157–62. doi: 10.1016/j.humimm.2003.10.005
96. Swets M, Wouters A, Krijgsman D, van Vlierberghe RLP, Boot A, van Eendenburg JD, et al. HLA-G Protein Expression in Colorectal Cancer Evaluated by Immunohistochemistry and Western Blot Analysis: Its Expression Characteristics Remain Enigmatic. Clin Immunol (2018) 194:80–6. doi: 10.1016/j.clim.2018.07.005
97. Loustau M, Anna F, Dréan R, Lecomte M, Langlade-Demoyen P, Caumartin J. Hla-G Neo-Expression on Tumors. Front Immunol (2020) 11:1685. . doi: 10.3389/fimmu.2020.01685
98. McMaster MT, Librach CL, Zhou Y, Lim KH, Janatpour MJ, DeMars R, et al. Human Placental HLA-G Expression Is Restricted to Differentiated Cytotrophoblasts. J Immunol (1995) 154:3771–8.
99. Emmer PM, Steegers EA, van Lierop MJ, Steegers-Theunissen RP, Loke YW, Joosten I. Amniotic Fluid Soluble Human Leukocyte Antigen G Is Markedly Decreased in Offspring With Neural Tube Defects. Early Hum Dev (2002) 66:101–5. doi: 10.1016/s0378-3782(01)00236-5
100. Rebmann V, Lemaoult J, Rouas-Freiss N, Carosella ED, Grosse-Wilde H. Report of the Wet Workshop for Quantification of Soluble HLA-G in Essen, 2004. Hum Immunol (2005) 66:853–63. doi: 10.1016/j.humimm.2005.05.003
101. Smyth EC, Gambardella V, Cervantes A, Fleitas T. Checkpoint Inhibitors for Gastroesophageal Cancers: Dissecting Heterogeneity to Better Understand Their Role in First Line and Adjuvant Therapy. Ann Oncol (2021) 32:590–9. doi: 10.1016/j.annonc.2021.02.004
102. Zhang X, Lin A, Han QY, Zhang JG, Chen QY, Ye YH, et al. Intratumor Heterogeneity of HLA-G Expression in Cancer Lesions. Front Immunol (2020) 11:565759. doi: 10.3389/fimmu.2020.565759
103. Rouas-Freiss N, LeMaoult J, Verine J, Tronik-Le Roux D, Culine S, Hennequin C, et al. Intratumor Heterogeneity of Immune Checkpoints in Primary Renal Cell Cancer: Focus on HLA-G/ILT2/ILT4. Oncoimmunology (2017) 6:e1342023. doi: 10.1080/2162402X.2017.1342023
104. Ferns DM, Heeren AM, Samuels S, Bleeker MCG, de Gruijl TD, Kenter GG, et al. Classical and Non-Classical HLA Class I Aberrations in Primary Cervical Squamous- and Adenocarcinomas and Paired Lymph Node Metastases. J Immunother Cancer (2016) 4:78. doi: 10.1186/s40425-016-0184-3
105. Lin A, Yan WH. Letter to the Editors: Growth Hormone Stops Excessive Inflammation After Partial Hepatectomy Allowing Liver Regeneration and Survival by Induction of H2-Bl/HLA-G. Hepatology (2021) 73:1238. doi: 10.1002/hep.31532
106. Morandi F, Rizzo R, Fainardi E, Rouas-Freiss N, Pistoia V. Recent Advances in Our Understanding of HLA-G Biology: Lessons From a Wide Spectrum of Human Diseases. J Immunol Res (2016) 2016:4326495. doi: 10.1155/2016/4326495
107. Hò GT, Celik AA, Huyton T, Hiemisch W, Blasczyk R, Simper GS, et al. Nkg2a/Cd94 Is a New Immune Receptor for HLA-G and Distinguishes Amino Acid Differences in the HLA-G Heavy Chain. Int J Mol Sci (2020) 21:4362. doi: 10.3390/ijms21124362
108. Rajagopalan S. Endosomal Signaling and a Novel Pathway Defined by the Natural Killer Receptor KIR2DL4 (Cd158d). Traffic (2010) 11:1381–90. doi: 10.1111/j.1600-0854.2010.01112.x
109. Rajagopalan S, Moyle MW, Joosten I, Long EO. Dna-PKcs Controls an Endosomal Signaling Pathway for a Proinflammatory Response by Natural Killer Cells. Sci Signal (2010) 3:ra14. doi: 10.1126/scisignal.2000467
110. Borrego F, Masilamani M, Kabat J, Sanni TB, Coligan JE. The Cell Biology of the Human Natural Killer Cell CD94/NKG2A Inhibitory Receptor. Mol Immunol (2005) 42:485–8. doi: 10.1016/j.molimm.2004.07.031
111. Celik AA, Simper GS, Huyton T, Blasczyk R, Bade-Döding C. HLA-G Mediated Immune Regulation Is Impaired by a Single Amino Acid Exchange in the Alpha 2 Domain. Hum Immunol (2018) 79:453–62. doi: 10.1016/j.humimm.2018.03.010
112. Fons P, Chabot S, Cartwright JE, Lenfant F, L’Faqihi F, Giustiniani J, et al. Soluble HLA-G1 Inhibits Angiogenesis Through an Apoptotic Pathway and by Direct Binding to CD160 Receptor Expressed by Endothelial Cells. Blood (2006) 108:2608–15. doi: 10.1182/blood-2005-12-019919
113. Contini P, Ghio M, Poggi A, Filaci G, Indiveri F, Ferrone S, et al. Soluble HLA-A,-B,-C and -G Molecules Induce Apoptosis in T and NK CD8+ Cells and Inhibit Cytotoxic T Cell Activity Through CD8 Ligation. Eur J Immunol (2003) 33:125–34. doi: 10.1002/immu.200390015
114. Le Bouteiller P, Tabiasco J, Polgar B, Kozma N, Giustiniani J, Siewiera J, et al. CD160: A Unique Activating NK Cell Receptor. Immunol Lett (2011) 138:93–6. doi: 10.1016/j.imlet.2011.02.003
115. Puppo F, Contini P, Ghio M, Indiveri F. Soluble HLA Class I Molecules/CD8 Ligation Trigger Apoptosis of CD8+ Cells by Fas/Fas-Ligand Interaction. ScientificWorldJournal (2002) 2:421–3. doi: 10.1100/tsw.2002.122
116. Carosella ED, Gregori S, Tronik-Le Roux D. HLA-G/Lilrbs: A Cancer Immunotherapy Challenge. Trends Cancer (2021) 7:389–92. doi: 10.1016/j.trecan.2021.01.004 S2405-8033(21)00017-0
117. Krijgsman D, Roelands J, Hendrickx W, Bedognetti D, Kuppen PJK. Hla-G: A New Immune Checkpoint in Cancer? Int J Mol Sci (2020) 21:4528. doi: 10.3390/ijms21124528
Keywords: HLA-G, immune checkpoint, immune checkpoint inhibitor, immunoglobulin-like transcript, cancer immunotherapy
Citation: Lin A and Yan W-H (2021) HLA-G/ILTs Targeted Solid Cancer Immunotherapy: Opportunities and Challenges. Front. Immunol. 12:698677. doi: 10.3389/fimmu.2021.698677
Received: 21 April 2021; Accepted: 14 June 2021;
Published: 30 June 2021.
Edited by:
Geraldo Aleixo Passos, University of São Paulo, BrazilReviewed by:
Erick C. Castelli, São Paulo State University, BrazilRusan Ali Catar, Charité–Universitätsmedizin Berlin, Germany
Copyright © 2021 Lin and Yan. This is an open-access article distributed under the terms of the Creative Commons Attribution License (CC BY). The use, distribution or reproduction in other forums is permitted, provided the original author(s) and the copyright owner(s) are credited and that the original publication in this journal is cited, in accordance with accepted academic practice. No use, distribution or reproduction is permitted which does not comply with these terms.
*Correspondence: Wei-Hua Yan, yanwhcom@yahoo.com