- 1Department of Laboratory Medicine, Binzhou Medical University, Binzhou, China
- 2Department of Oncology, Xiangya Cancer Center, Xiangya Hospital, Central South University, Changsha, China
- 3Key Laboratory of Molecular Radiation Oncology Hunan Province, Xiangya Hospital, Central South University, Changsha, China
- 4Hunan International Science and Technology Collaboration Base of Precision Medicine for Cancer, Xiangya Hospital, Central South University, Changsha, China
- 5Hunan Provincial Clinical Research Center for Respiratory Diseases, Xiangya Hospital, Central South University, Changsha, China
- 6Institute of Gerontological Cancer Research, National Clinical Research Center for Gerontology, Changsha, China
- 7Center for Molecular Imaging of Central South University, Xiangya Hospital, Changsha, China
- 8Department of Clinical Laboratory, Binzhou Medical University Hospital, Binzhou, China
- 9Biomedical Pioneering Innovation Center (BIOPIC), Beijing Advanced Innovation Center for Genomics, Peking-Tsinghua Center for Life Sciences, Peking University Genome Editing Research Center, State Key Laboratory of Protein and Plant Gene Research, School of Life Sciences, Peking University, Beijing, China
The transcription factor Snail1, a key inducer of epithelial-mesenchymal transition (EMT), plays a critical role in tumor metastasis. Its stability is strictly controlled by multiple intracellular signal transduction pathways and the ubiquitin-proteasome system (UPS). Increasing evidence indicates that methylation and acetylation of Snail1 also affects tumor metastasis. More importantly, Snail1 is involved in tumor immunosuppression by inducing chemokines and immunosuppressive cells into the tumor microenvironment (TME). In addition, some immune checkpoints potentiate Snail1 expression, such as programmed death ligand 1 (PD-L1) and T cell immunoglobulin 3 (TIM-3). This mini review highlights the pathways and molecules involved in maintenance of Snail1 level and the significance of Snail1 in tumor immune evasion. Due to the crucial role of EMT in tumor metastasis and tumor immunosuppression, comprehensive understanding of Snail1 function may contribute to the development of novel therapeutics for cancer.
Introduction
Metastasis is one of the most prominent features of malignant tumors and is the leading cause of death in tumor patients (1). Tumor metastasis is a multi-step process in which EMT has a crucial regulatory role. During the process of EMT, epithelial cells lose their cell polarity and cell–cell adhesion, and transit to quasi-mesenchymal cell states, thus increasing their migration and invasion properties (2). Recent studies indicated that tumor progression and metastasis are closely related to epigenetic modifications and the immune system. It was reported that immune checkpoint molecules such as PD-L1 are involved in EMT regulation, while EMT can also induce immunosuppression and immune evasion in tumors (3).
The Snail family of zinc finger transcription factors comprises three members in vertebrates, Snail1 (Snail), Snail2 (Slug), Snail3 (Smuc) (4, 5). Snail1 and Snail2 down-regulate the expression of many target proteins associated with EMT. Among them, the most significant one is E-cadherin (6). Due to the critical role of Snail1 in EMT, this mini review focuses on how appropriate Snail1 levels are maintained in cells, with emphasis on the role of epigenetic and UPS in the regulation of Snail1. Furthermore, we also discuss the involvement of Snail1 in tumor immune evasion, a role which has made it a promising therapeutics target in tumor treatment.
Structural and Functional Characteristics of Snail1
In all Snail family members, the amino terminal end contains a highly conserved SNAG domain, which functions as a transcriptional repressor domain (7). The fingers correspond to the C2H2 type and bind to the upstream regulatory region of target genes for gene specific transcriptional inhibition (8). The central region of Snail2 includes the Slug domain, while Snail1 has two defined functional domains in this region: a regulatory domain containing an Xpo1/CRM1 mediated nuclear export signal (NES) (9) and a serine-rich domain involved in the regulation of its stability (10) (Figure 1A).
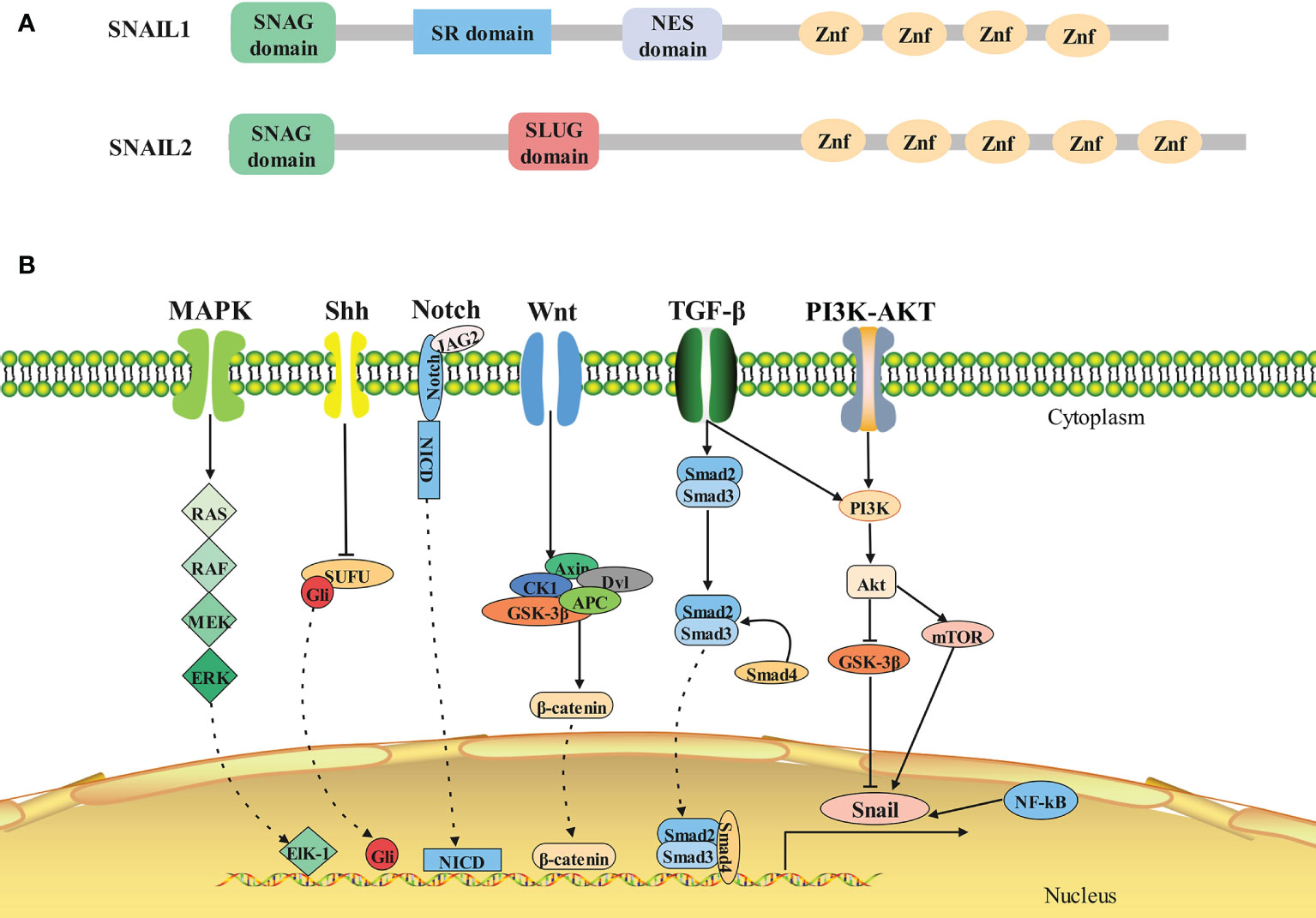
Figure 1 Structure and signaling pathways of SNAIL1. (A)Architecture of SNAIL1 in human. Composite of the overall structure of Snail1 and Snail2, which shows the relative positions of the SNAG domain, the zinc fingers (I–V), and the Slug-specific boxes, NES domain and serine-rich domain. (B)The molecular signaling pathways of SNAIL1. Snail1 is regulated by several signaling pathways that promote its expression. From left to right: MAPK, Shh, Notch, Wnt, TGF-β, PI3K-AKT, and NF-κB signaling pathway.
Snail1, as a transcriptional repressor, is implicated in the regulation of other tumor metastasis suppressors, such as the epithelial marker E-cadherin (11). Previous studies reported that SNAG domain of Snail1 couples on the CDH1 (which encodes E-cadherin) promoter (12), and recruits histone deacetylase (HDAC). Subsequently, Snail1, HDAC1, HDAC2 and mSin3A conjointly form a multi-molecular complex that further inhibits the expression of E-cadherin (13). Furthermore, Snail1 interacts with the H3K9 methyltransferase G9A or SUV39H1 and recruits it to the CDH1 promoter for transcriptional inhibition in breast cancer (14, 15), collectively resulting in the occurrence of EMT.
The Signaling Pathways Involved in Snail1 Expression
The expression of Snail1 is regulated by many signaling pathways both at the transcriptional and protein level (16, 17) (Figure 1B). Physiologically, these signaling pathways control normal cell morphology, proliferation, differentiation, and apoptosis. However, abnormal activation of these signaling pathways contribute to the initiation and progression of tumors activated (18, 19).
TGF-β Pathway
The transforming growth factor-β (TGF-β) was described as an inducer of EMT during the development of tumor (20). Mechanistically, TGF-β binds to its receptor TβRII and TβRI, which subsequently phosphorylates its downstream targets, including members of the SMAD family of signal transducers, SMAD2 and SMAD3 (21), forming a heterooligomeric complex with SMAD4 (22). Then this SMAD complex translocates to the nucleus and functions as a transcription factor to regulate the transcription of target genes such as Snail1 in human tumors (16, 21, 23–25).
PI3K-Akt Pathway
The phosphatidylinositol 3-kinase (PI3K)-Akt signaling pathway is hyperactivated or altered in many cancer types (26–28) and regulates a broad range of cellular processes (29, 30). It is well known that Akt can phosphorylate and inhibit GSK-3β activity, subsequently suppressing the GSK-3β-mediated phosphorylation of Snail1 and facilitating its stabilization and nuclear localization, which ultimately promotes EMT widely presenting in a variety of tumors (31–36). In addition to GSK-3β, PI3K-Akt also activates mTOR, thereby potentiating Snail1 expression in gastric, breast, pancreatic and ovarian cancer (37–40). Furthermore, some studies also indicate TGF-β regulates Snail1 expression via the Akt/GSK-3β signaling pathway in osteosarcoma and ovarian clear cell carcinoma (41, 42).
Wnt Pathway
In the presence of Wnt signaling, the destruction complex (APC, Dvl, Axin, GSK-3β, CK1) reduces the phosphorylation and ubiquitination of β-catenin (43).Thus, levels of cytoplasmic β-catenin rise, which translocates to the nucleus and induces transcription of pro-invasive factors (44–46). Moreover, β-catenin/T-cell factor (TCF) transcriptional complex regulates Snail1 via Axin2-mediated nuclear export of GSK-3β in breast cancer (47).
Notch Pathway
Notch signaling is generated through the interaction between Notch receptors and ligands such as Jagged-2 (JAG2) (48). Notch is released into the cytoplasm by intracellular segment (NICD) after being sheared three times (49), and then enters the nucleus to bind to the Snail1 promoter, directly stimulating transcription (50).
Shh Pathway
Sonic Hedgehog (Shh) is a lipid-modified secreted protein that couples to Patched receptor (51). In the presence of Hedgehog signaling, Smoothened is relieved from Patched-mediated suppression due to the Hedgehog-dependent internalization of Patched, which leads to inactivation of SUFU for the stabilization and nuclear accumulation of Gli family members (51, 52). So far, Shh-mediated Gli1 activation was reported to induce the expression of Snail1 in a variety of cancers, such as breast, skin, ovarian, pancreatic, neuroendocrine cancer and basal cell carcinoma (45, 53–57).
MAPK Pathway
The Ras/Raf/MEK/ERK pathway is the most important signaling cascade among all MAPK signal (58). Once activated, ERK translocates to the nucleus, binds to and regulates the activity of the transcription factor Elk-1 through phosphorylation (58). Of note, activation of Elk1 facilitates recruitment of phosphorylated mitogen and stress activated protein kinase 1 (MSK1), which in turn enhances histone H3 acetylation and phosphorylation (serine 10) of Snail1 promoter, ultimately promoting the transcription of Snail1 (59). Furthermore hepatocyte growth factor (HGF) could induce transcription of Snail1 by activating MAPK signaling pathway in liver cancer (60).
NF-κB Pathway
The over-activation of nuclear factor-κB (NF-κB) with a role in the inflammatory response, immune response and cell apoptosis, is associated with multifarious tumors (61). Previous studies have demonstrated that the activation of the NF-κB pathway blocked the degradation and promoted the transcription of Snail1 (62), subsequently facilitating the migration and invasion in breast, colorectal, gastric cancers, cholangiocarcinoma and malignant human keratinocyte (63–66).
Regulation of Snail1 Expression by Ubiquitin-Proteasome Ststem
Ubiquitin mediates protein degradation via binding to lysine residues of the substrate proteins (67). It is highly conserved in eukaryotic cells and can also function as a signaling molecule to modulate protein function (68). Its eight residues including M1, K6, K11, K27, K29, K33, K48, and K63 are used as attachment sites to form polyubiquitin chains (69). The most abundant chain types are K48, which are usually degraded by the 26S proteasome (68).
Degradation of SNAIL1 by UPS
Snail1 is an extremely unstable protein, β-TrCP1 was first reported to be involved in Snail1 ubiquitination via GSK-3β mediated phosphorylation of S96 and S100 residues on Snail1 (10). In contrast, Snail1 is ubiquitinated independently of GSK-3β phosphorylation by FBXL14 through K98, 137, and 146 residues (70). Interestingly, miR-27a can directly down-regulate the expression of FBXO45, resulting in reduced Snail1 degradation (71). In breast cancer, the S11 residue of Snail1 is phosphorylated by PKD1, which promotes the ubiquitination and degradation of Snail1 by FBXO11 (72, 73), while FBXO22 depends on GSK-3β (74). In addition, it has been reported that PPIL2, SPSB3 and TRIM21 are involved in ubiquitination and degradation of Snail1 (75–77). In gastric cancer, phosphorylation of Snail1 is required for the F-box domain of FBXO31 to function (78), FBXW7 inhibits metastasis in part by binding to Snail1 (79) and FBXL5 promotes poly-ubiquitination of Snail1 at K85, K146 and K234 residues (80, 81). In Non-small cell lung cancer, both β-TrCP2 and FBXW7 are absolutely implicated in ubiquitination and degradation of Snail1 (82, 83). In cervical cancer, HECTD1-mediated degradation of Snail1 occurs in the cytoplasm rather than in the nucleus (84). Finally, other E3 ligases such as TRIM50 and CHIP, are also involved in regulation of Snail1 in hepatocarcinoma and ovarian cancer, respectively (85, 86).
So far, some molecular targets have been found based on the above E3 ligases, which are potential therapeutic targets. LINC00511 and EBV-miR-Bart10-3p both inhibit β-TrCP1 and prevent Snail1 degradation in triple negative breast cancer and nasopharyngeal carcinoma, respectively (87, 88). In non-small cell lung cancer, the expression of the β-TrCP2 is inhibited by miR-106b-25 (83), while FBXW7 agonist (Oridonin) contributes to the degradation of Snail1 (89). In hepatocellular carcinoma, miR-1306-3p directly targets FBXL5 to suppress Snail1 degradation (90). Likewise, miR-27a immediately down-regulate the expression of FBXO45 (91). Particularly, BRD4 identifies acetylated K146 and K187 on Snail1 in an acetylation-dependent manner to prevent its degradation by FBXL14 and β-TrCP1 in gastric cancer (92). Inversely, Metformin is beneficial to the expression of LKB1, thereby strengthening the capacity of FBXL14 in pancreatic cancer (93) (Figure 2A).
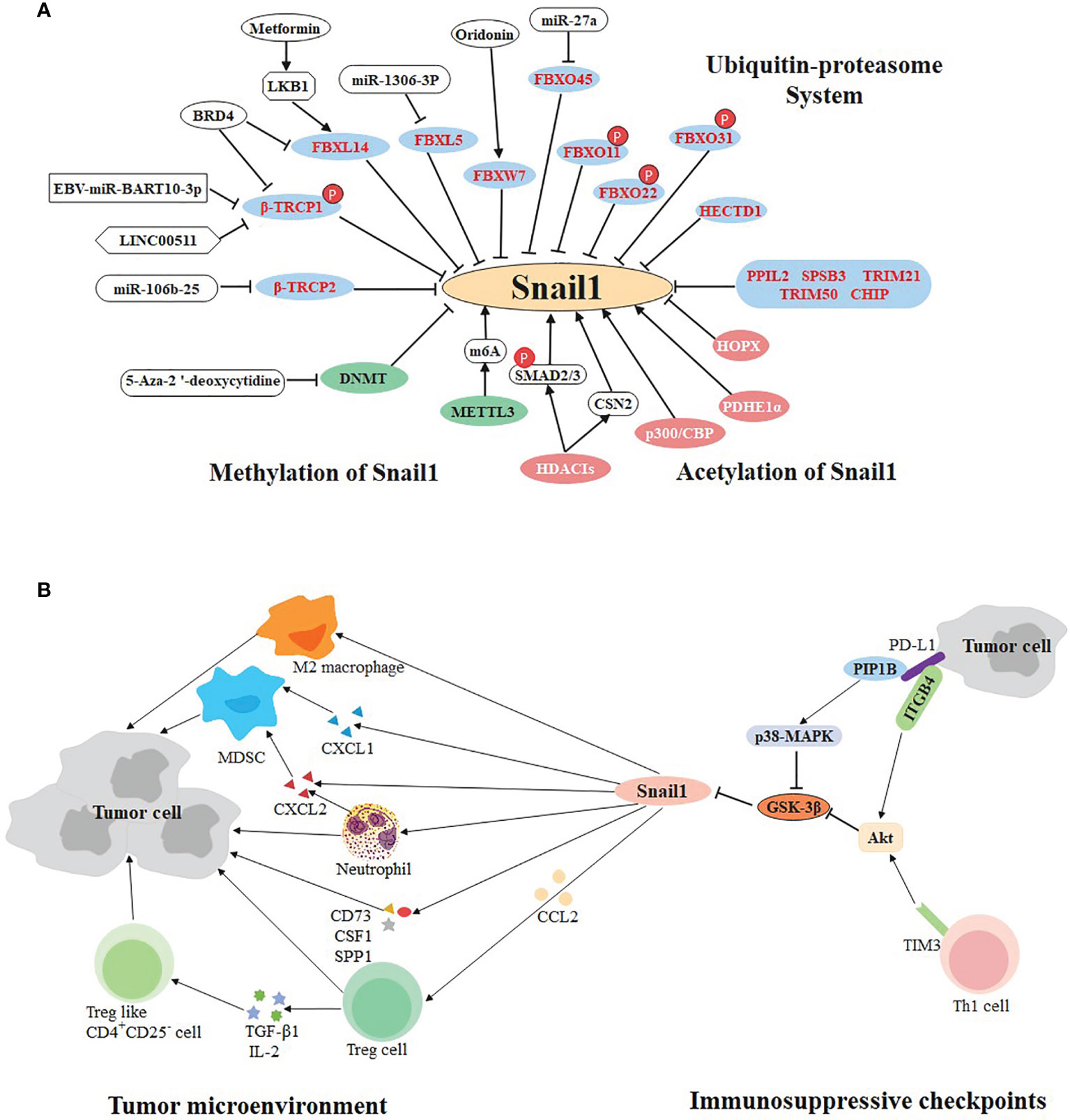
Figure 2 Multifaceted regulation of SNAIL1. (A) Ubiquitination, methylation, and acetylation regulate the expression of SNAIL1. The ubiquitin E3 ligases in the blue circle negatively regulate the expression of Snail1. Small molecule compounds or drugs directly or indirectly act on E3 ligase to promote or inhibit the expression of Snail1. Molecules in the green and pink circles participate in methylation and acetylation of Snail1, respectively. (B) Bidirectional regulation of SNAIL1 and tumor immune environment. Snail1 recruits immunosuppressive cells (including Treg, MDSCs, M2 macrophages, neutrophils, and Treg-like CD4+CD25- cells) to participate in the formation of tumor microenvironment via cytokines, chemokines and their receptors. In addition, immunosuppressive checkpoints PD-L1 and TIM-3 can regulate the expression of Snail1 through different molecular pathways.
Stabilizing the Expression of SNAIL1 by DUBs
Ubiquitination is a reversible process and ubiquitin moieties are removed from polypeptides by deubiquitinases (DUBs) (94). Currently, plentiful DUBs are involved in the occurrence, progression, and drug resistance of cancer (95–97). In esophageal squamous cell carcinoma, OTUB1 inhibits the ubiquitination and degradation of SMAD2/3, leading to strengthen TGF-β signaling and stabilization of Snail1 expression (98, 99). Interestingly, USP26 is a specific deubiquitinase of Snail1 and significantly increases its stability by combining with the zinc finger domain at the Snail1, an essential region for its stability and nuclear localization (100, 101). In addition, EIF3H and PSMD14 have also been found to be involved in Snail1 deubiquitination (102, 103). In breast cancer, DUB3 couples on SNAG domain of Snail1 and inhibits ubiquitination of Snail1 mediated by FBXL14 and β-TrCP1 (104). Analogously, CSN2 removes the ubiquitination of Snail1 via disrupting its binding to GSK-3β and β-TrCP (62). In lung cancer, CSN5 and USP37 significantly stabilize the expression of Snail1. More importantly, USP37 is closely associated with increased mortality and metastasis rates (105, 106). In glioblastoma, USP3 also hydrolyzes FBXO11 or FBXW1-induced polyubiquitination chain on Snail1, resulting in increased aggressiveness and tumorigenicity (107). Similarly, high expression of OTUB1 in gliomas is associated with poor prognosis (108). In colorectal cancer, up-regulation of USP47 is mediated by SOX9, leading to an increase in Snail1 deubiquitination under hypoxia condition (109). In gastric cancer, USP29 enhanced the interaction between Snail1 and SCP1, causing both dephosphorylation and deubiquitination of Snail1 (110).
Epigenetic Modification in Snail1 Regulation
Epigenetic abnormalities have been linked to many human diseases, including cancer (111, 112). Particularly, methylation and acetylation are involved in Snail1-mediated tumor metastasis (Figure 2A).
Methylation of SNAIL1
DNA methylation is an important mechanism of epigenetic gene regulation, which primarily occurs at CpG dinucleotide within gene promoters by a covalent modification of cytosine residues via DNA methyltransferase (DNMT) enzymes (113). It was previously reported that DNA methylation in the first intron region of Snail1 was negatively correlated with its transcription level, but its expression was increased when treated with DNMT inhibitor 5-Aza-2 ‘-deoxycytidine in trophoblast cells (114). Uniformly, the chromatin remodeling factor ARID2 represses EMT of hepatocellular carcinoma by recruiting DNMT1 to Snail1 promoter, which increases promoter methylation and inhibits its transcription (115). Recently, m6A RNA methylation is an emerging epigenetic modification, which has been associated with the progression of several cancers (116, 117). Interestingly, m6A is methylated by Methyltransferase-like 3 (METTL3) to accelerate Snail1 expression in HeLa cells (118), which is equivalent to indirect regulation of Snail1 by methylation.
Acetylation of SNAIL1
Protein acetylation was originally discovered on histones in the nucleus and involved in gene transcription (119). Subsequently, non-histone proteins were increasingly found to also undergo acetylation (120). In nasopharyngeal carcinoma, the glucose metabolizing enzyme PDHE1α facilitates H3K9 acetylation on the Snail1 promoter to enhance cell motility and thereby drive cancer metastasis (121). Inversely, HOPX mediates epigenetic silencing of Snail1 transcription through the enhancement of histone H3K9 deacetylation in the Snail1 promoter (122). In lung cancer cells, p300 acetylates Snail1 at K187 (123), and CREB-binding protein (CBP) interacts with and acetylates Snail1 at K146 and K187, which prevents formation of the repressor complex (124). As mentioned above, BRD4 recognizes acetylated K146 and K187 on Snail1 to prevent it from being degraded by E3 ligases in gastric cancer (92).
At present, histone deacetylase inhibitors (HDACIs) are now emerging as a new class of anticancer agents (125, 126). However, HDACIs stabilize surprisingly Snail1 expression through several mechanisms in hepatocellular carcinoma: HDACIs up-regulate Snail1 at the transcriptional level by promoting SMAD2/3 phosphorylation and nuclear translocation (127). Posteriorly, HDACIs regulate the stabilization of Snail1 via up-regulating the expression of CSN2, which interacts with Snail1 to expose its acetylation site, leading to inhibit degradation of Snail1 via preventing its phosphorylation and ubiquitination (127). Coincidentally, this phenomenon was also observed in CNE2 cells (128). Accordingly, more cautions should be exercised in the usage of medicines such as HDACIs, as they may increase the risk of tumor metastasis.
Bidirectional Regulation of Snail1 and Tumor Immune Environment in Tumor Progression
Tumorigenesis and progression are influenced by tumor microenvironment and controlled by the host immune system (129). In addition to malignant cells, adipocytes, fibroblasts, tumor vasculature, lymphocytes, dendritic cells, and cancer-associated fibroblasts are present in the tumor microenvironment (130). The last decade has witnessed dramatic advances in cancer treatment through immunotherapy such as immune checkpoints inhibitors, which are the most popular and promising treatment at present (131). Recently, the bidirectional regulation of immune checkpoints and EMT was uncovered via Snail1 (Figure 2B).
Immunosuppressive Checkpoints Regulate SNAIL1 Expression
So far, two immune checkpoint proteins PD-L1 and TIM-3 have been found to regulate Snail1 expression. PD-L1, which accumulates to high level on the surface of some tumor cells, can bind to PD-1 and induce T cells exhaustion, thereby mediating tumor immune escape and potentiating tumor progression (132, 133). Histochemical staining of 477 lung adenocarcinoma specimens showed a positive correlation between the expression of PD-L1 and Snail1 (134). Two studies showed that PD-L1 can inhibit GSK3β activity via binding to tyrosine phosphatase PTP1B or integrin β4 to activate p38-MAPK or Akt activity, respectively. Through this mechanism, PD-L1 can inhibit GSK3β-mediated phosphorylation, ubiquitination, and degradation of Snail1, thereby promoting EMT and the metastatic potential of breast cancer and cervical cancer (135, 136).
TIM-3 contains an immunoglobulin and a mucin-like domain and was originally identified as a receptor expressed on Th1 cells (137). The silencing of TIM-3 was accompanied by a decrease in Snail1 expression, indicating that TIM-3 may be involved in metastasis of osteosarcoma and hepatocellular carcinoma (138–140). Due to the lack of research in this aspect, it is only known that TIM-3 induces EMT to stimulate the metastasis of esophageal squamous cell carcinoma at least partly through the Akt/GSK-3β/Snail1 signaling pathway (141).
The role of SNAIL1 in Tumor Immune Evasion
Increasing evidence suggests that Snail1 is also involved in immune escape from tumors, which can accelerate cancer metastasis. Previous research has reported the quantity of tumor-specific infiltrating lymphocytes and the systemic immune response increased via silencing Snail1 in melanoma (142), suggesting that Snail1 is visibly involved in tumor immunity. Firstly, Snail1 recruits CD4+FOXP3+Treg cells into the tumor microenvironment through C-C motif chemokine ligand 2 (CCL2) (143). In a mouse model of lung cancer, Snail1 was also found to increase intratumoral C-X-C chemokine ligand 2 (CXCL2) secretion and neutrophil infiltration (144). In ovarian cancer, Snail1 accelerates cancer progression via up-regulation of CXCL1 and CXCL2 as well as recruitment of myeloid-derived suppressor cells (MDSCs) (145), which plays a vital role in cancer immunosuppression, tumor angiogenesis, drug resistance and promotion of tumor metastasis (146, 147). In cholangiocarcinoma, Snail1 appears to produce immunosuppressive natural T-regulatory like CD4+CD25- cells, in part by mediating the T regulatory-inducible cytokines such as TGF-β1 and IL-2 (148). In addition, Snail1 induces M2 polarization of tumor-associated macrophages and facilitates tumor growth in head and neck cancer (149). A recent study showed that the high expression of Snail1 in mesenchymal tumor cell induces the expression of several cytokines (CD73, CSF1, SPP1), which collectively expedites the assembly of tumor immunosuppressive microenvironments (2). All these lines of evidence strongly confirm that Snail1 effectively promotes tumor cells to secrete chemokines or cytokines, which recruits various immunosuppressive cells to the tumor microenvironment and provides an appropriate environment for tumor metastasis.
Summary and Future Perspectives
Physiologically, Snail1 participates in embryo implantation and initiation, wound healing, and cell survival (8, 150, 151). In addition, as we discussed above, Snail1 is a crucial target involved in tumor metastasis and immune escape, and can endow tumor cells with the characteristics of stem cells (5). What’s more, Snail1 overexpression was found to be a potential risk factor of neoplasm recurrence in various cancers, such as cutaneous squamous cell carcinoma, clear cell renal cell carcinoma, ameloblastic carcinoma, non-muscle-invasive bladder, colon and non-small-cell lung cancer (152–157). Consistent with tumor relapse, Snail1 overexpression also indicates poor prognosis in several types of cancers (158–161). Taken together, Snail1 can function as a biomarker to predict tumor relapse and patient prognosis.
Snail1 also hold critical role in cancer treatment, increasing evidence suggested that Snail1 is implicated in chemotherapy and radiotherapy resistance. For instance, silencing Snail1 was found to be beneficial in enhancing the sensitivity of gemcitabine therapy in pancreatic ductal carcinoma (162, 163) and increasing radiosensitivity in hypopharyngeal carcinoma (164). Furthermore, Snail1 contributes to the resistance of glioblastoma cells to temozolomide via the IL-6-STAT3-Snail1 pathway (165) and colorectal cancer cells to 5-fluorouracil by facilitating the expression of the ABCB1 resistance gene (166). In addition, Snail1 overexpression could induce tumor stem cell-like phenotype and generate chemotherapy resistance to oxaliplatin in colorectal cancer (167). Collectively, chemotherapy or radiotherapy combined with Snail1 inhibitors such as CYD19 (168), GN-25 (169) and Co (III)-Ebox (170) may be a promising therapeutic approach to combat tumors. At present, it has not yet been reported whether Snail1 is involved in immune checkpoint blockade. Due to knockdown of Snail1 decreases the infiltration of immunosuppressive cells in the tumor microenvironment, it is possible targeting Snail1 could enhance the anti-tumor effect. Accordingly, further development of novel Snail1 inhibitors and investigation of the safety of these compounds is urgently need for conquering cancer in future.
Author Contributions
XT and XS were responsible for the primary review of literature, consolidation of information, and writing. LW and YL guided and supervised this study. All authors contributed to the article and approved the submitted version.
Funding
This work was supported by National Natural Science Foundations of China (81802400 to YL, 81900199 and 81974465 to LW), China Postdoctoral Science Foundation (2020M670053 to YL), Hunan province natural science funds for Excellent Young Scholars (2019JJ30043 to LW) and the recruitment program for Huxiang talents (2019RS1009 to LW).
Conflict of Interest
The authors declare that the research was conducted in the absence of any commercial or financial relationships that could be construed as a potential conflict of interest.
Publisher’s Note
All claims expressed in this article are solely those of the authors and do not necessarily represent those of their affiliated organizations, or those of the publisher, the editors and the reviewers. Any product that may be evaluated in this article, or claim that may be made by its manufacturer, is not guaranteed or endorsed by the publisher.
References
1. Lin Y, Xu J, Lan H. Tumor-Associated Macrophages in Tumor Metastasis: Biological Roles and Clinical Therapeutic Applications. J Hematol Oncol (2019) 12:76. doi: 10.1186/s13045-019-0760-3
2. Dongre A, Rashidian M, Eaton EN, Reinhardt F, Thiru P, Zagorulya M, et al. Direct and Indirect Regulators of Epithelial-Mesenchymal Transition-Mediated Immunosuppression in Breast Carcinomas. Cancer Discov (2021) 11:1286–305. doi: 10.1158/2159-8290.CD-20-0603
3. Messeha SS, Zarmouh NO, Soliman K. Polyphenols Modulating Effects of PD-L1/PD-1 Checkpoint and EMT-Mediated PD-L1 Overexpression in Breast Cancer. Nutrients (2021) 13:1718–9. doi: 10.3390/nu13051718
4. Hemavathy K, Ashraf SI, Ip YT. Snail/slug Family of Repressors: Slowly Going Into the Fast Lane of Development and Cancer. Gene (2000) 257:1–12. doi: 10.1016/s0378-1119(00)00371-1
5. Wang Y, Shi J, Chai K, Ying X, Zhou BP. The Role of Snail in EMT and Tumorigenesis. Curr Cancer Drug Targets (2013) 13:963–72. doi: 10.2174/15680096113136660102
6. Whiteman EL, Liu CJ, Fearon ER, Margolis B. The Transcription Factor Snail Represses Crumbs3 Expression and Disrupts Apico-Basal Polarity Complexes. Oncogene (2008) 27:3875–9. doi: 10.1038/onc.2008.9
7. Chiang C, Ayyanathan K. Snail/Gfi-1 (SNAG) Family Zinc Finger Proteins in Transcription Regulation, Chromatin Dynamics, Cell Signaling, Development, and Disease. Cytokine Growth Factor Rev (2013) 24:123–31. doi: 10.1016/j.cytogfr.2012.09.002
8. Nieto MA. The Snail Superfamily of Zinc-Finger Transcription Factors. Nat Rev Mol Cell Biol (2002) 3:155–66. doi: 10.1038/nrm757
9. Dominguez D, Montserrat-Sentis B, Virgos-Soler A, Guaita S, Grueso J, Porta M, et al. Phosphorylation Regulates the Subcellular Location and Activity of the Snail Transcriptional Repressor. Mol Cell Biol (2003) 23:5078–89. doi: 10.1128/MCB.23.14.5078-5089.2003
10. Zhou BP, Deng J, Xia W, Xu J, Li YM, Gunduz M, et al. Dual Regulation of Snail by GSK-3beta-Mediated Phosphorylation in Control of Epithelial-Mesenchymal Transition. Nat Cell Biol (2004) 6:931–40. doi: 10.1038/ncb1173
11. Hanahan D, Weinberg RA. Hallmarks of Cancer: The Next Generation. Cell (2011) 144:646–74. doi: 10.1016/j.cell.2011.02.013
12. Gall TM, Frampton AE. Gene of the Month: E-Cadherin (CDH1). J Clin Pathol (2013) 66:928–32. doi: 10.1136/jclinpath-2013-201768
13. Peinado H, Ballestar E, Esteller M, Cano A. Snail Mediates E-Cadherin Repression by the Recruitment of the Sin3A/histone Deacetylase 1 (HDAC1)/HDAC2 Complex. Mol Cell Biol (2004) 24:306–19. doi: 10.1128/MCB.24.1.306-319.2004
14. Dong C, Wu Y, Yao J, Wang Y, Yu Y, Rychahou PG, et al. G9a Interacts With Snail and is Critical for Snail-Mediated E-Cadherin Repression in Human Breast Cancer. J Clin Invest (2012) 122:1469–86. doi: 10.1172/JCI57349
15. Dong C, Wu Y, Wang Y, Wang C, Kang T, Rychahou PG, et al. Interaction With Suv39H1 is Critical for Snail-Mediated E-Cadherin Repression in Breast Cancer. Oncogene (2013) 32:1351–62. doi: 10.1038/onc.2012.169
16. Odero-Marah V, Hawsawi O, Henderson V, Sweeney J. Epithelial-Mesenchymal Transition (EMT) and Prostate Cancer. Adv Exp Med Biol (2018) 1095:101–10. doi: 10.1007/978-3-319-95693-0_6
17. Vu T, Datta PK. Regulation of EMT in Colorectal Cancer: A Culprit in Metastasis. Cancers (Basel) (2017) 9:171. doi: 10.3390/cancers9120171
18. Nieszporek A, Skrzypek K, Adamek G, Majka M. Molecular Mechanisms of Epithelial to Mesenchymal Transition in Tumor Metastasis. Acta Biochim Pol (2019) 66:509–20. doi: 10.18388/abp.2019_2899
19. Liu X, Yun F, Shi L, Li ZH, Luo NR, Jia YF. Roles of Signaling Pathways in the Epithelial-Mesenchymal Transition in Cancer. Asian Pac J Cancer Prev (2015) 16:6201–6. doi: 10.7314/apjcp.2015.16.15.6201
20. Xu J, Lamouille S, Derynck R. TGF-Beta-Induced Epithelial to Mesenchymal Transition. Cell Res (2009) 19:156–72. doi: 10.1038/cr.2009.5
21. Miyazono K. Transforming Growth Factor-Beta Signaling in Epithelial-Mesenchymal Transition and Progression of Cancer. Proc Jpn Acad Ser B Phys Biol Sci (2009) 85:314–23. doi: 10.2183/pjab.85.314
22. Vincent T, Neve EP, Johnson JR, Kukalev A, Rojo F, Albanell J, et al. A SNAIL1-SMAD3/4 Transcriptional Repressor Complex Promotes TGF-Beta Mediated Epithelial-Mesenchymal Transition. Nat Cell Biol (2009) 11:943–50. doi: 10.1038/ncb1905
23. Jiang X, Zhang Z, Song C, Deng H, Yang R, Zhou L, et al. Glaucocalyxin A Reverses EMT and TGF-Beta1-Induced EMT by Inhibiting TGF-Beta1/Smad2/3 Signaling Pathway in Osteosarcoma. Chem Biol Interact (2019) 307:158–66. doi: 10.1016/j.cbi.2019.05.005
24. Tong X, Wang S, Lei Z, Li C, Zhang C, Su Z, et al. MYOCD and SMAD3/SMAD4 Form a Positive Feedback Loop and Drive TGF-Beta-Induced Epithelial-Mesenchymal Transition in Non-Small Cell Lung Cancer. Oncogene (2020) 39:2890–904. doi: 10.1038/s41388-020-1189-4
25. Li C, Ao H, Chen G, Wang F, Li F. The Interaction of CDH20 With Beta-Catenin Inhibits Cervical Cancer Cell Migration and Invasion via TGF-Beta/Smad/SNAIL Mediated EMT. Front Oncol (2019) 9:1481. doi: 10.3389/fonc.2019.01481
26. Costa R, Han HS, Gradishar WJ. Targeting the PI3K/AKT/mTOR Pathway in Triple-Negative Breast Cancer: A Review. Breast Cancer Res Treat (2018) 169:397–406. doi: 10.1007/s10549-018-4697-y
27. Chen H, Zhou L, Wu X, Li R, Wen J, Sha J, et al. The PI3K/AKT Pathway in the Pathogenesis of Prostate Cancer. Front Biosci (Landmark Ed) (2016) 21:1084–91. doi: 10.2741/4443
28. Marquard FE, Jucker M. PI3K/AKT/mTOR Signaling as a Molecular Target in Head and Neck Cancer. Biochem Pharmacol (2020) 172:113729. doi: 10.1016/j.bcp.2019.113729
29. Ersahin T, Tuncbag N, Cetin-Atalay R. The PI3K/AKT/mTOR Interactive Pathway. Mol Biosyst (2015) 11:1946–54. doi: 10.1039/c5mb00101c
30. Porta C, Paglino C, Mosca A. Targeting PI3K/Akt/mTOR Signaling in Cancer. Front Oncol (2014) 4:64. doi: 10.3389/fonc.2014.00064
31. Zhao GX, Xu YY, Weng SQ, Zhang S, Chen Y, Shen XZ, et al. CAPS1 Promotes Colorectal Cancer Metastasis via Snail Mediated Epithelial Mesenchymal Transformation. Oncogene (2019) 38:4574–89. doi: 10.1038/s41388-019-0740-7
32. Liu JJ, Li LZ, Xu P. Upregulation of TRPM8 can Promote the Colon Cancer Liver Metastasis Through Mediating Akt/GSK-3 Signal Pathway. Biotechnol Appl Biochem (2021) 0:1–10. doi: 10.1002/bab.2102
33. Lan Y, Han J, Wang Y, Wang J, Yang G, Li K, et al. STK17B Promotes Carcinogenesis and Metastasis via AKT/GSK-3beta/Snail Signaling in Hepatocellular Carcinoma. Cell Death Dis (2018) 9:236. doi: 10.1038/s41419-018-0262-1
34. Liu H, Xu L, He H, Zhu Y, Liu J, Wang S, et al. Hepatitis B Virus X Protein Promotes Hepatoma Cell Invasion and Metastasis by Stabilizing Snail Protein. Cancer Sci (2012) 103:2072–81. doi: 10.1111/cas.12017
35. Gao Y, Zheng H, Li L, Zhou C, Chen X, Zhou X, et al. KIF3C Promotes Proliferation, Migration, and Invasion of Glioma Cells by Activating the PI3K/AKT Pathway and Inducing EMT. BioMed Res Int (2020) 2020:6349312. doi: 10.1155/2020/6349312
36. Chen L, Wu Q, Xu X, Yang C, You J, Chen F, et al. Cancer/testis Antigen LDHC Promotes Proliferation and Metastasis by Activating the PI3K/Akt/GSK-3beta-Signaling Pathway and the in Lung Adenocarcinoma. Exp Cell Res (2021) 398:112414. doi: 10.1016/j.yexcr.2020.112414
37. Wu YJ, Lin SH, Din ZH, Su JH, Liu CI. Sinulariolide Inhibits Gastric Cancer Cell Migration and Invasion Through Downregulation of the EMT Process and Suppression of FAK/PI3K/AKT/mTOR and MAPKs Signaling Pathways. Mar Drugs (2019) 17:668. doi: 10.3390/md17120668
38. Cheng Y, Pan Y, Pan Y, Wang O. MNX1-AS1 is a Functional Oncogene That Induces EMT and Activates the AKT/mTOR Pathway and MNX1 in Breast Cancer. Cancer Manag Res (2019) 11:803–12. doi: 10.2147/CMAR.S188007
39. Sharma N, Nanta R, Sharma J, Gunewardena S, Singh KP, Shankar S, et al. PI3K/AKT/mTOR and Sonic Hedgehog Pathways Cooperate Together to Inhibit Human Pancreatic Cancer Stem Cell Characteristics and Tumor Growth. Oncotarget (2015) 6:32039–60. doi: 10.18632/oncotarget.5055
40. Lau MT, Leung PC. The PI3K/Akt/mTOR Signaling Pathway Mediates Insulin-Like Growth Factor 1-Induced E-Cadherin Down-Regulation and Cell Proliferation in Ovarian Cancer Cells. Cancer Lett (2012) 326:191–8. doi: 10.1016/j.canlet.2012.08.016
41. Yu B, Jiang K, Zhang J. MicroRNA-124 Suppresses Growth and Aggressiveness of Osteosarcoma and Inhibits TGF-Beta-Mediated AKT/GSK-3beta/SNAIL-1 Signaling. Mol Med Rep (2018) 17:6736–44. doi: 10.3892/mmr.2018.8637
42. Matsumoto T, Yokoi A, Hashimura M, Oguri Y, Akiya M, Saegusa M. TGF-Beta-Mediated LEFTY/Akt/GSK-3beta/Snail Axis Modulates Epithelial-Mesenchymal Transition and Cancer Stem Cell Properties in Ovarian Clear Cell Carcinomas. Mol Carcinog (2018) 57:957–67. doi: 10.1002/mc.22816
43. Schaefer KN, Peifer M. Wnt/Beta-Catenin Signaling Regulation and a Role for Biomolecular Condensates. Dev Cell (2019) 48:429–44. doi: 10.1016/j.devcel.2019.01.025
44. Clevers H, Nusse R. Wnt/beta-Catenin Signaling and Disease. Cell (2012) 149:1192–205. doi: 10.1016/j.cell.2012.05.012
45. Li X, Deng W, Lobo-Ruppert SM, Ruppert JM. Gli1 Acts Through Snail and E-Cadherin to Promote Nuclear Signaling by Beta-Catenin. Oncogene (2007) 26:4489–98. doi: 10.1038/sj.onc.1210241
46. Schmalhofer O, Brabletz S. Brabletz T. E-Cadherin, Beta-Catenin, and ZEB1 in Malignant Progression of Cancer. Cancer Metastasis Rev (2009) 28:151–66. doi: 10.1007/s10555-008-9179-y
47. Yook JI, Li XY, Ota I, Hu C, Kim HS, Kim NH, et al. Weiss SJ. A Wnt-Axin2-GSK3beta Cascade Regulates Snail1 Activity in Breast Cancer Cells. Nat Cell Biol (2006) 8:1398–406. doi: 10.1038/ncb1508
48. Houde C, Li Y, Song L, Barton K, Zhang Q, Godwin J, et al. Overexpression of the NOTCH Ligand JAG2 in Malignant Plasma Cells From Multiple Myeloma Patients and Cell Lines. Blood (2004) 104:3697–704. doi: 10.1182/blood-2003-12-4114
49. Kopan R, Ilagan MX. The Canonical Notch Signaling Pathway: Unfolding the Activation Mechanism. Cell (2009) 137:216–33. doi: 10.1016/j.cell.2009.03.045
50. Wang Z, Li Y, Kong D, Sarkar FH. The Role of Notch Signaling Pathway in Epithelial-Mesenchymal Transition (EMT) During Development and Tumor Aggressiveness. Curr Drug Targets (2010) 11:745–51. doi: 10.2174/138945010791170860
51. Carballo GB, Honorato JR, de Lopes G, Spohr T. A Highlight on Sonic Hedgehog Pathway. Cell Commun Signal (2018) 16:11. doi: 10.1186/s12964-018-0220-7
52. Katoh Y, Katoh M. Hedgehog Signaling, Epithelial-to-Mesenchymal Transition and miRNA (Review). Int J Mol Med (2008) 22:271–5. doi: 10.3892/ijmm_00000019
53. Riaz SK, Ke Y, Wang F, Kayani MA, Malik M. Influence of SHH/GLI1 Axis on EMT Mediated Migration and Invasion of Breast Cancer Cells. Sci Rep (2019) 9:6620. doi: 10.1038/s41598-019-43093-x
54. Ke Z, Caiping S, Qing Z, Xiaojing W. Sonic Hedgehog-Gli1 Signals Promote Epithelial-Mesenchymal Transition in Ovarian Cancer by Mediating PI3K/AKT Pathway. Med Oncol (2015) 32:368. doi: 10.1007/s12032-014-0368-y
55. Feldmann G, Dhara S, Fendrich V, Bedja D, Beaty R, Mullendore M, et al. Blockade of Hedgehog Signaling Inhibits Pancreatic Cancer Invasion and Metastases: A New Paradigm for Combination Therapy in Solid Cancers. Cancer Res (2007) 67:2187–96. doi: 10.1158/0008-5472.CAN-06-3281
56. Fendrich V, Waldmann J, Esni F, Ramaswamy A, Mullendore M, Buchholz M, et al. Snail and Sonic Hedgehog Activation in Neuroendocrine Tumors of the Ileum. Endocr Relat Cancer (2007) 14:865–74. doi: 10.1677/ERC-07-0108
57. Li X, Deng W, Nail CD, Bailey SK, Kraus MH, Ruppert JM, et al. Snail Induction is an Early Response to Gli1 That Determines the Efficiency of Epithelial Transformation. Oncogene (2006) 25:609–21. doi: 10.1038/sj.onc.1209077
58. Guo YJ, Pan WW, Liu SB, Shen ZF, Xu Y, Hu LL. ERK/MAPK Signalling Pathway and Tumorigenesis. Exp Ther Med (2020) 19:1997–2007. doi: 10.3892/etm.2020.8454
59. Hsu YL, Hou MF, Kuo PL, Huang YF, Tsai EM. Breast Tumor-Associated Osteoblast-Derived CXCL5 Increases Cancer Progression by ERK/MSK1/Elk-1/Snail Signaling Pathway. Oncogene (2013) 32:4436–47. doi: 10.1038/onc.2012.444
60. Grotegut S, von Schweinitz D, Christofori G, Lehembre F. Hepatocyte Growth Factor Induces Cell Scattering Through MAPK/Egr-1-Mediated Upregulation of Snail. EMBO J (2006) 25:3534–45. doi: 10.1038/sj.emboj.7601213
61. Dolcet X, Llobet D, Pallares J, Matias-Guiu X. NF-kB in Development and Progression of Human Cancer. Virchows Arch (2005) 446:475–82. doi: 10.1007/s00428-005-1264-9
62. Wu Y, Deng J, Rychahou PG, Qiu S, Evers BM, Zhou BP. Stabilization of Snail by NF-kappaB is Required for Inflammation-Induced Cell Migration and Invasion. Cancer Cell (2009) 15:416–28. doi: 10.1016/j.ccr.2009.03.016
63. Yang Y, Li Y, Wang K, Wang Y, Yin W, Li L. P38/NF-Kappab/Snail Pathway is Involved in Caffeic Acid-Induced Inhibition of Cancer Stem Cells-Like Properties and Migratory Capacity in Malignant Human Keratinocyte. PloS One (2013) 8:e58915. doi: 10.1371/journal.pone.0058915
64. Zhang K, Zhaos J, Liu X, Yan B, Chen D, Gao Y, et al. Activation of NF-B Upregulates Snail and Consequent Repression of E-Cadherin in Cholangiocarcinoma Cell Invasion. Hepatogastroenterology (2011) 58:1–7. doi: 10.1186/1746-160X-7-15
65. Zhu QC, Gao RY, Wu W, Qin HL. Epithelial-Mesenchymal Transition and Its Role in the Pathogenesis of Colorectal Cancer. Asian Pac J Cancer Prev (2013) 14:2689–98. doi: 10.7314/apjcp.2013.14.5.2689
66. Li J, Deng Z, Wang Z, Wang D, Zhang L, Su Q, et al. Zipper-Interacting Protein Kinase Promotes Epithelial-Mesenchymal Transition, Invasion and Metastasis Through AKT and NF-kB Signaling and Is Associated With Metastasis and Poor Prognosis in Gastric Cancer Patients. Oncotarget (2015) 6:8323–38. doi: 10.18632/oncotarget.3200
67. Swatek KN, Komander D. Ubiquitin Modifications. Cell Res (2016) 26:399–422. doi: 10.1038/cr.2016.39
68. Nandi D, Tahiliani P, Kumar A, Chandu D. The Ubiquitin-Proteasome System. J Biosci (2006) 31:137–55. doi: 10.1007/BF02705243
69. Wu X, Luo Q, Liu Z. Ubiquitination and Deubiquitination of MCL1 in Cancer: Deciphering Chemoresistance Mechanisms and Providing Potential Therapeutic Options. Cell Death Dis (2020) 11:556. doi: 10.1038/s41419-020-02760-y
70. Vinas-Castells R, Beltran M, Valls G, Gomez I, Garcia JM, Montserrat-Sentis B, et al. The Hypoxia-Controlled FBXL14 Ubiquitin Ligase Targets SNAIL1 for Proteasome Degradation. J Biol Chem (2010) 285:3794–805. doi: 10.1074/jbc.M109.065995
71. Xu M, Zhu C, Zhao X, Chen C, Zhang H, Yuan H, et al. Atypical Ubiquitin E3 Ligase Complex Skp1-Pam-Fbxo45 Controls the Core Epithelial-to-Mesenchymal Transition-Inducing Transcription Factors. Oncotarget (2015) 6:979–94. doi: 10.18632/oncotarget.2825
72. Jin Y, Shenoy AK, Doernberg S, Chen H, Luo H, Shen H, et al. FBXO11 Promotes Ubiquitination of the Snail Family of Transcription Factors in Cancer Progression and Epidermal Development. Cancer Lett (2015) 362:70–82. doi: 10.1016/j.canlet.2015.03.037
73. Zheng H, Shen M, Zha YL, Li W, Wei Y, Blanco MA, et al. PKD1 Phosphorylation-Dependent Degradation of SNAIL by SCF-FBXO11 Regulates Epithelial-Mesenchymal Transition and Metastasis. Cancer Cell (2014) 26:358–73. doi: 10.1016/j.ccr.2014.07.022
74. Sun R, Xie HY, Qian JX, Huang YN, Yang F, Zhang FL, et al. FBXO22 Possesses Both Protumorigenic and Antimetastatic Roles in Breast Cancer Progression. Cancer Res (2018) 78:5274–86. doi: 10.1158/0008-5472.CAN-17-3647
75. Jia Z, Wang M, Li S, Li X, Bai XY, Xu Z, et al. Wu H. U-Box Ubiquitin Ligase PPIL2 Suppresses Breast Cancer Invasion and Metastasis by Altering Cell Morphology and Promoting SNAI1 Ubiquitination and Degradation. Cell Death Dis (2018) 9:63. doi: 10.1038/s41419-017-0094-4
76. Liu Y, Zhou H, Zhu R, Ding F, Li Y, Cao X, et al. SPSB3 Targets SNAIL for Degradation in GSK-3beta Phosphorylation-Dependent Manner and Regulates Metastasis. Oncogene (2018) 37:768–76. doi: 10.1038/onc.2017.370
77. Jin Y, Zhang Y, Li B, Zhang J, Dong Z, Hu X, et al. TRIM21 Mediates Ubiquitination of Snail and Modulates Epithelial to Mesenchymal Transition in Breast Cancer Cells. Int J Biol Macromol (2019) 124:846–53. doi: 10.1016/j.ijbiomac.2018.11.269
78. Zou S, Ma C, Yang F, Xu X, Jia J, Liu Z. FBXO31 Suppresses Gastric Cancer EMT by Targeting Snail1 for Proteasomal Degradation. Mol Cancer Res (2018) 16:286–95. doi: 10.1158/1541-7786.MCR-17-0432
79. Li H, Wang Z, Zhang W, Qian K, Xu W, Zhang S. Fbxw7 Regulates Tumor Apoptosis, Growth Arrest and the Epithelial-to-Mesenchymal Transition in Part Through the RhoA Signaling Pathway in Gastric Cancer. Cancer Lett (2016) 370:39–55. doi: 10.1016/j.canlet.2015.10.006
80. Wu W, Ding H, Cao J, Zhang W. FBXL5 Inhibits Metastasis of Gastric Cancer Through Suppressing Snail1. Cell Physiol Biochem (2015) 35:1764–72. doi: 10.1159/000373988
81. Vinas-Castells R, Frias A, Robles-Lanuza E, Zhang K, Longmore GD, Garcia DHA, et al. Nuclear Ubiquitination by FBXL5 Modulates Snail1 DNA Binding and Stability. Nucleic Acids Res (2014) 42:1079–94. doi: 10.1093/nar/gkt935
82. Xiao G, Li Y, Wang M, Li X, Qin S, Sun X, et al. FBXW7 Suppresses Epithelial-Mesenchymal Transition and Chemo-Resistance of Non-Small-Cell Lung Cancer Cells by Targeting Snai1 for Ubiquitin-Dependent Degradation. Cell Prolif (2018) 51:e12473. doi: 10.1111/cpr.12473
83. Savita U, Karunagaran D. MicroRNA-106b-25 Cluster Targets Beta-TRCP2, Increases the Expression of Snail and Enhances Cell Migration and Invasion in H1299 (Non Small Cell Lung Cancer) Cells. Biochem Biophys Res Commun (2013) 434:841–7. doi: 10.1016/j.bbrc.2013.04.025
84. Wang X, De Geyter C, Jia Z, Peng Y, Zhang H. HECTD1 Regulates the Expression of SNAIL: Implications for Epithelialmesenchymal Transition. Int J Oncol (2020) 56:1186–98. doi: 10.3892/ijo.2020.5002
85. Ma X, Ma X, Qiu Y, Zhu L, Lin Y, You Y, et al. TRIM50 Suppressed Hepatocarcinoma Progression Through Directly Targeting SNAIL for Ubiquitous Degradation. Cell Death Dis (2018) 9:608. doi: 10.1038/s41419-018-0644-4
86. Park SM, Park SH, Ryu KJ, Kim IK, Han H, Kim HJ, et al. Downregulation of CHIP Promotes Ovarian Cancer Metastasis by Inducing Snail-Mediated Epithelial-Mesenchymal Transition. Mol Oncol (2019) 13:1280–95. doi: 10.1002/1878-0261.12485
87. Liu R, Wang L, Gan T, Pan T, Huang J, Bai M. Long Noncoding RNA LINC00511 Promotes Cell Growth and Invasion in Triple-Negative Breast Cancer by Interacting With Snail. Cancer Manag Res (2019) 11:5691–9. doi: 10.2147/CMAR.S203455
88. Yan Q, Zeng Z, Gong Z, Zhang W, Li X, He B, et al. EBV-miR-BART10-3p Facilitates Epithelial-Mesenchymal Transition and Promotes Metastasis of Nasopharyngeal Carcinoma by Targeting BTRC. Oncotarget (2015) 6:41766–82. doi: 10.18632/oncotarget.6155
89. Zhang Y, Zhang X, Ye M, Jing P, Xiong J, Han Z, et al. FBW7 Loss Promotes Epithelial-to-Mesenchymal Transition in Non-Small Cell Lung Cancer Through the Stabilization of Snail Protein. Cancer Lett (2018) 419:75–83. doi: 10.1016/j.canlet.2018.01.047
90. He ZJ, Li W, Chen H, Wen J, Gao YF, Liu YJ. miR-1306-3p Targets FBXL5 to Promote Metastasis of Hepatocellular Carcinoma Through Suppressing Snail Degradation. Biochem Biophys Res Commun (2018) 504:820–6. doi: 10.1016/j.bbrc.2018.09.059
91. Lin M, Wang ZW, Zhu X. FBXO45 is a Potential Therapeutic Target for Cancer Therapy. Cell Death Discovery (2020) 6:55. doi: 10.1038/s41420-020-0291-2
92. Qin ZY, Wang T, Su S, Shen LT, Zhu GX, Liu Q, et al. BRD4 Promotes Gastric Cancer Progression and Metastasis Through Acetylation-Dependent Stabilization of Snail. Cancer Res (2019) 79:4869–81. doi: 10.1158/0008-5472.CAN-19-0442
93. Song L, Guo J, Chang R, Peng X, Li J, Xu X, et al. LKB1 Obliterates Snail Stability and Inhibits Pancreatic Cancer Metastasis in Response to Metformin Treatment. Cancer Sci (2018) 109:1382–92. doi: 10.1111/cas.13591
94. Komander D, Clague MJ, Urbe S. Breaking the Chains: Structure and Function of the Deubiquitinases. Nat Rev Mol Cell Biol (2009) 10:550–63. doi: 10.1038/nrm2731
95. Cheng J, Guo J, North BJ, Wang B, Cui CP, Li H, et al. Functional Analysis of Deubiquitylating Enzymes in Tumorigenesis and Development. Biochim Biophys Acta Rev Cancer (2019) 1872:188312. doi: 10.1016/j.bbcan.2019.188312
96. Wei R, Liu X, Yu W, Yang T, Cai W, Liu J, et al. Deubiquitinases in Cancer. Oncotarget (2015) 6:12872–89. doi: 10.18632/oncotarget.3671
97. Tanguturi P, Kim KS, Ramakrishna S. The Role of Deubiquitinating Enzymes in Cancer Drug Resistance. Cancer Chemother Pharmacol (2020) 85:627–39. doi: 10.1007/s00280-020-04046-8
98. Herhaus L, Al-Salihi M, Macartney T, Weidlich S, Sapkota GP. OTUB1 Enhances TGFbeta Signalling by Inhibiting the Ubiquitylation and Degradation of Active SMAD2/3. Nat Commun (2013) 4:2519. doi: 10.1038/ncomms3519
99. Zhou H, Liu Y, Zhu R, Ding F, Cao X, Lin D, et al. OTUB1 Promotes Esophageal Squamous Cell Carcinoma Metastasis Through Modulating Snail Stability. ONCOGENE (2018) 37:3356–68. doi: 10.1038/s41388-018-0224-1
100. Li L, Zhou H, Zhu R, Liu Z. USP26 Promotes Esophageal Squamous Cell Carcinoma Metastasis Through Stabilizing Snail. Cancer Lett (2019) 448:52–60. doi: 10.1016/j.canlet.2019.02.007
101. Ning B, Zhao W, Qian C, Liu P, Li Q, Li W, et al. USP26 Functions as a Negative Regulator of Cellular Reprogramming by Stabilising PRC1 Complex Components. Nat Commun (2017) 8:349. doi: 10.1038/s41467-017-00301-4
102. Guo X, Zhu R, Luo A, Zhou H, Ding F, Yang H, et al. EIF3H Promotes Aggressiveness of Esophageal Squamous Cell Carcinoma by Modulating Snail Stability. J Exp Clin Cancer Res (2020) 39:175. doi: 10.1186/s13046-020-01678-9
103. Zhu R, Liu Y, Zhou H, Li L, Li Y, Ding F, et al. Deubiquitinating Enzyme PSMD14 Promotes Tumor Metastasis Through Stabilizing SNAIL in Human Esophageal Squamous Cell Carcinoma. Cancer Lett (2018) 418:125–34. doi: 10.1016/j.canlet.2018.01.025
104. Wu Y, Wang Y, Lin Y, Liu Y, Wang Y, Jia J, et al. Dub3 Inhibition Suppresses Breast Cancer Invasion and Metastasis by Promoting Snail1 Degradation. Nat Commun (2017) 8:14228. doi: 10.1038/ncomms14228
105. Watanabe K, Yokoyama S, Kaneto N, Hori T, Iwakami Y, Kato S, et al. COP9 Signalosome Subunit 5 Regulates Cancer Metastasis by Deubiquitinating SNAIL. Oncotarget (2018) 9:20670–80. doi: 10.18632/oncotarget.25060
106. Cai J, Li M, Wang X, Li L, Li Q, Hou Z, et al. USP37 Promotes Lung Cancer Cell Migration by Stabilizing Snail Protein via Deubiquitination. Front Genet (2019) 10:1324. doi: 10.3389/fgene.2019.01324
107. Fan L, Chen Z, Wu X, Cai X, Feng S, Lu J, et al. Ubiquitin-Specific Protease 3 Promotes Glioblastoma Cell Invasion and Epithelial-Mesenchymal Transition via Stabilizing Snail. Mol Cancer Res (2019) 17:1975–84. doi: 10.1158/1541-7786.MCR-19-0197
108. Xu L, Li J, Bao Z, Xu P, Chang H, Wu J, et al. Silencing of OTUB1 Inhibits Migration of Human Glioma Cells In Vitro. Neuropathology (2017) 37:217–26. doi: 10.1111/neup.12366
109. Choi BJ, Park SA, Lee SY, Cha YN, Surh YJ. Hypoxia Induces Epithelial-Mesenchymal Transition in Colorectal Cancer Cells Through Ubiquitin-Specific Protease 47-Mediated Stabilization of Snail: A Potential Role of Sox9. Sci Rep (2017) 7:15918. doi: 10.1038/s41598-017-15139-5
110. Qian W, Li Q, Wu X, Li W, Li Q, Zhang J, et al. Deubiquitinase USP29 Promotes Gastric Cancer Cell Migration by Cooperating With Phosphatase SCP1 to Stabilize Snail Protein. Oncogene (2020) 39:6802–15. doi: 10.1038/s41388-020-01471-0
111. Hogg SJ, Beavis PA, Dawson MA, Johnstone RW. Targeting the Epigenetic Regulation of Antitumour Immunity. Nat Rev Drug Discovery (2020) 19:776–800. doi: 10.1038/s41573-020-0077-5
112. Dawson MA, Kouzarides T. Cancer Epigenetics: From Mechanism to Therapy. Cell (2012) 150:12–27. doi: 10.1016/j.cell.2012.06.013
113. Baylin SB, Herman JG. DNA Hypermethylation in Tumorigenesis: Epigenetics Joins Genetics. Trends Genet (2000) 16:168–74. doi: 10.1016/s0168-9525(99)01971-x
114. Chen Y, Wang K, Qian CN, Leach R. DNA Methylation Is Associated With Transcription of Snail and Slug Genes. Biochem Biophys Res Commun (2013) 430:1083–90. doi: 10.1016/j.bbrc.2012.12.034
115. Jiang H, Cao HJ, Ma N, Bao WD, Wang JJ, Chen TW, et al. Chromatin Remodeling Factor ARID2 Suppresses Hepatocellular Carcinoma Metastasis via DNMT1-Snail Axis. Proc Natl Acad Sci U S A (2020) 117:4770–80. doi: 10.1073/pnas.1914937117
116. Liu ZX, Li LM, Sun HL, Liu SM. Link Between M6a Modification and Cancers. Front Bioeng Biotechnol (2018) 6:89. doi: 10.3389/fbioe.2018.00089
117. Sun T, Wu R, Ming L. The Role of M6a RNA Methylation in Cancer. BioMed Pharmacother (2019) 112:108613. doi: 10.1016/j.biopha.2019.108613
118. Li J, Chen F, Peng Y, Lv Z, Lin X, Chen Z, et al. N6-Methyladenosine Regulates the Expression and Secretion of TGFbeta1 to Affect the Epithelial-Mesenchymal Transition of Cancer Cells. CELLS-BASEL (2020) 9:296. doi: 10.3390/cells9020296
119. Guo P, Chen W, Li H, Li M, Li L. The Histone Acetylation Modifications of Breast Cancer and Their Therapeutic Implications. Pathol Oncol Res (2018) 24:807–13. doi: 10.1007/s12253-018-0433-5
120. Narita T, Weinert BT, Choudhary C. Functions and Mechanisms of Non-Histone Protein Acetylation. Nat Rev Mol Cell Biol (2019) 20:156–74. doi: 10.1038/s41580-018-0081-3
121. Zhang J, Jia L, Liu T, Yip YL, Tang WC, Lin W, et al. Mtorc2-Mediated PDHE1alpha Nuclear Translocation Links EBV-LMP1 Reprogrammed Glucose Metabolism to Cancer Metastasis in Nasopharyngeal Carcinoma. Oncogene (2019) 38:4669–84. doi: 10.1038/s41388-019-0749-y
122. Ren X, Yang X, Cheng B, Chen X, Zhang T, He Q, et al. HOPX Hypermethylation Promotes Metastasis via Activating SNAIL Transcription in Nasopharyngeal Carcinoma. Nat Commun (2017) 8:14053. doi: 10.1038/ncomms14053
123. Chang R, Zhang Y, Zhang P, Zhou Q. Snail Acetylation by Histone Acetyltransferase P300 in Lung Cancer. Thorac Cancer (2017) 8:131–7. doi: 10.1111/1759-7714.12408
124. Hsu DS, Wang HJ, Tai SK, Chou CH, Hsieh CH, Chiu PH, et al. Acetylation of Snail Modulates the Cytokinome of Cancer Cells to Enhance the Recruitment of Macrophages. Cancer Cell (2014) 26:534–48. doi: 10.1016/j.ccell.2014.09.002
125. West AC, Johnstone RW. New and Emerging HDAC Inhibitors for Cancer Treatment. J Clin Invest (2014) 124:30–9. doi: 10.1172/JCI69738
126. Lakshmaiah KC, Jacob LA, Aparna S, Lokanatha D, Saldanha SC. Epigenetic Therapy of Cancer With Histone Deacetylase Inhibitors. J Cancer Res Ther (2014) 10:469–78. doi: 10.4103/0973-1482.137937
127. Xu W, Liu H, Liu ZG, Wang HS, Zhang F, Wang H, et al. Histone Deacetylase Inhibitors Upregulate Snail via Smad2/3 Phosphorylation and Stabilization of Snail to Promote Metastasis of Hepatoma Cells. Cancer Lett (2018) 420:1–13. doi: 10.1016/j.canlet.2018.01.068
128. Jiang GM, Wang HS, Zhang F, Zhang KS, Liu ZC, Fang R, et al. Histone Deacetylase Inhibitor Induction of Epithelial-Mesenchymal Transitions via Up-Regulation of Snail Facilitates Cancer Progression. Biochim Biophys Acta (2013) 1833:663–71. doi: 10.1016/j.bbamcr.2012.12.002
129. Lei X, Lei Y, Li JK, Du WX, Li RG, Yang J, et al. Immune Cells Within the Tumor Microenvironment: Biological Functions and Roles in Cancer Immunotherapy. Cancer Lett (2020) 470:126–33. doi: 10.1016/j.canlet.2019.11.009
131. Li B, Chan HL, Chen P. Immune Checkpoint Inhibitors: Basics and Challenges. Curr Med Chem (2019) 26:3009–25. doi: 10.2174/0929867324666170804143706
132. Han Y, Liu D, Li L. PD-1/PD-L1 Pathway: Current Researches in Cancer. Am J Cancer Res (2020) 10:727–42.
133. Yi M, Jiao D, Xu H, Liu Q, Zhao W, Han X, et al. Biomarkers for Predicting Efficacy of PD-1/PD-L1 Inhibitors. Mol Cancer (2018) 17:129. doi: 10.1186/s12943-018-0864-3
134. Kim S, Koh J, Kim MY, Kwon D, Go H, Kim YA, et al. PD-L1 Expression is Associated With Epithelial-to-Mesenchymal Transition in Adenocarcinoma of the Lung. Hum Pathol (2016) 58:7–14. doi: 10.1016/j.humpath.2016.07.007
135. Chen C, Li S, Xue J, Qi M, Liu X, Huang Y, et al. PD-L1 Tumor-Intrinsic Signaling and Its Therapeutic Implication in Triple-Negative Breast Cancer. JCI Insight (2021) 6:e131458. doi: 10.1172/jci.insight.131458
136. Wang S, Li J, Xie J, Liu F, Duan Y, Wu Y, et al. Programmed Death Ligand 1 Promotes Lymph Node Metastasis and Glucose Metabolism in Cervical Cancer by Activating Integrin Beta4/SNAI1/SIRT3 Signaling Pathway. Oncogene (2018) 37:4164–80. doi: 10.1038/s41388-018-0252-x
137. Monney L, Sabatos CA, Gaglia JL, Ryu A, Waldner H, Chernova T, et al. Th1-Specific Cell Surface Protein Tim-3 Regulates Macrophage Activation and Severity of an Autoimmune Disease. Nature (2002) 415:536–41. doi: 10.1038/415536a
138. Shang Y, Li Z, Li H, Xia H, Lin Z. TIM-3 Expression in Human Osteosarcoma: Correlation With the Expression of Epithelial-Mesenchymal Transition-Specific Biomarkers. Oncol Lett (2013) 6:490–4. doi: 10.3892/ol.2013.1410
139. Lin H, Yang B. Teng M. T-Cell Immunoglobulin Mucin-3 as a Potential Inducer of the Epithelial-Mesenchymal Transition in Hepatocellular Carcinoma. Oncol Lett (2017) 14:5899–905. doi: 10.3892/ol.2017.6961
140. Feng ZM, Guo SM. Tim-3 Facilitates Osteosarcoma Proliferation and Metastasis Through the NF-kappaB Pathway and Epithelial-Mesenchymal Transition. Genet Mol Res (2016) 15:10.4238/gmr.15037844. doi: 10.4238/gmr.15037844
141. Shan B, Man H, Liu J, Wang L, Zhu T, Ma M, et al. TIM-3 Promotes the Metastasis of Esophageal Squamous Cell Carcinoma by Targeting Epithelial-Mesenchymal Transition via the Akt/GSK-3beta/Snail Signaling Pathway. Oncol Rep (2016) 36:1551–61. doi: 10.3892/or.2016.4938
142. Kudo-Saito C, Shirako H, Takeuchi T, Kawakami Y. Cancer Metastasis is Accelerated Through Immunosuppression During Snail-Induced EMT of Cancer Cells. Cancer Cell (2009) 15:195–206. doi: 10.1016/j.ccr.2009.01.023
143. Kudo-Saito C, Shirako H, Ohike M, Tsukamoto N, Kawakami Y. CCL2 is Critical for Immunosuppression to Promote Cancer Metastasis. Clin Exp Metastasis (2013) 30:393–405. doi: 10.1007/s10585-012-9545-6
144. Faget J, Groeneveld S, Boivin G, Sankar M, Zangger N, Garcia M, et al. Neutrophils and Snail Orchestrate the Establishment of a Pro-Tumor Microenvironment in Lung Cancer. Cell Rep (2017) 21:3190–204. doi: 10.1016/j.celrep.2017.11.052
145. Taki M, Abiko K, Baba T, Hamanishi J, Yamaguchi K, Murakami R, et al. Snail Promotes Ovarian Cancer Progression by Recruiting Myeloid-Derived Suppressor Cells via CXCR2 Ligand Upregulation. Nat Commun (2018) 9:1685. doi: 10.1038/s41467-018-03966-7
146. Gabrilovich DI. Myeloid-Derived Suppressor Cells. Cancer Immunol Res (2017) 5:3–8. doi: 10.1158/2326-6066.CIR-16-0297
147. Kumar V, Patel S, Tcyganov E, Gabrilovich DI. The Nature of Myeloid-Derived Suppressor Cells in the Tumor Microenvironment. Trends Immunol (2016) 37:208–20. doi: 10.1016/j.it.2016.01.004
148. Qian Y, Yao W, Yang T, Yang Y, Liu Y, Shen Q, et al. aPKC-Iota/P-Sp1/Snail Signaling Induces Epithelial-Mesenchymal Transition and Immunosuppression in Cholangiocarcinoma. Hepatology (2017) 66:1165–82. doi: 10.1002/hep.29296
149. Hsieh CH, Tai SK, Yang MH. Snail-Overexpressing Cancer Cells Promote M2-Like Polarization of Tumor-Associated Macrophages by Delivering MiR-21-Abundant Exosomes. Neoplasia (2018) 20:775–88. doi: 10.1016/j.neo.2018.06.004
150. Du F, Yang R, Ma HL, Wang QY, Wei SL. Expression of Transcriptional Repressor Slug Gene in Mouse Endometrium and Its Effect During Embryo Implantation. Appl Biochem Biotechnol (2009) 157:346–55. doi: 10.1007/s12010-008-8521-8
151. Swain SD, Grifka-Walk HN, Gripentrog J, Lehmann M, Deuling B, Jenkins B, et al. Slug and Snail Have Differential Effects in Directing Colonic Epithelial Wound Healing and Partially Mediate the Restitutive Effects of Butyrate. Am J Physiol Gastrointest Liver Physiol (2019) 317:G531–44. doi: 10.1152/ajpgi.00071.2019
152. Liu W, Liu Y, Liu H, Zhang W, An H, Xu J. Snail Predicts Recurrence and Survival of Patients With Localized Clear Cell Renal Cell Carcinoma After Surgical Resection. Urol Oncol (2015) 33:69.e1–10. doi: 10.1016/j.urolonc.2014.08.003
153. Gou Y, Ding W, Xu K, Wang H, Chen Z, Tan J, et al. Snail is an Independent Prognostic Indicator for Predicting Recurrence and Progression in Non-Muscle-Invasive Bladder Cancer. Int Urol Nephrol (2015) 47:289–93. doi: 10.1007/s11255-014-0874-z
154. Oh KY, Yoon HJ, Lee JI, Ahn SH, Hong SD. Twist and Snail Expression in Tumor and Stromal Cells of Epithelial Odontogenic Tumors. J Oral Pathol Med (2017) 46:127–33. doi: 10.1111/jop.12479
155. Wang G, Ma W, Li Y, Jiang Y, Ma G, Zhang X, et al. Prognostic Value of Twist, Snail and E-Cadherin Expression in Pathological N0 Non-Small-Cell Lung Cancer: A Retrospective Cohort Study. Eur J Cardiothorac Surg (2018) 54:237–45. doi: 10.1093/ejcts/ezy022
156. Rashed HE, Hussein S, Mosaad H, Abdelwahab MM, Abdelhamid MI, Mohamed SY, et al. Prognostic Significance of the Genetic and the Immunohistochemical Expression of Epithelial-Mesenchymal-Related Markers in Colon Cancer. Cancer Biomark (2017) 20:107–22. doi: 10.3233/CBM-170034
157. Zhao G, Kim KY, Zheng Z, Oh Y, Yoo DS, Lee ME, et al. AXIN2 and SNAIL Expression Predict the Risk of Recurrence in Cutaneous Squamous Cell Carcinoma After Mohs Micrographic Surgery. Oncol Lett (2020) 19:2133–40. doi: 10.3892/ol.2020.11324
158. Kwon CH, Park HJ, Choi JH, Lee JR, Kim HK, Jo HJ, et al. Snail and Serpina1 Promote Tumor Progression and Predict Prognosis in Colorectal Cancer. Oncotarget (2015) 6:20312–26. doi: 10.18632/oncotarget.3964
159. van Nes JG, de Kruijf EM, Putter H, Faratian D, Munro A, Campbell F, et al. Co-Expression of SNAIL and TWIST Determines Prognosis in Estrogen Receptor-Positive Early Breast Cancer Patients. Breast Cancer Res Treat (2012) 133:49–59. doi: 10.1007/s10549-011-1684-y
160. Mikami S, Katsube K, Oya M, Ishida M, Kosaka T, Mizuno R, et al. Expression of Snail and Slug in Renal Cell Carcinoma: E-Cadherin Repressor Snail is Associated With Cancer Invasion and Prognosis. Lab Invest (2011) 91:1443–58. doi: 10.1038/labinvest.2011.111
161. Shin NR, Jeong EH, Choi CI, Moon HJ, Kwon CH, Chu IS, et al. Overexpression of Snail is Associated With Lymph Node Metastasis and Poor Prognosis in Patients With Gastric Cancer. BMC Cancer (2012) 12:521. doi: 10.1186/1471-2407-12-521
162. Zheng X, Carstens JL, Kim J, Scheible M, Kaye J, Sugimoto H, et al. Epithelial-To-Mesenchymal Transition is Dispensable for Metastasis But Induces Chemoresistance in Pancreatic Cancer. Nature (2015) 527:525–30. doi: 10.1038/nature16064
163. Richards KE, Zeleniak AE, Fishel ML, Wu J, Littlepage LE, Hill R. Cancer-Associated Fibroblast Exosomes Regulate Survival and Proliferation of Pancreatic Cancer Cells. Oncogene (2017) 36:1770–8. doi: 10.1038/onc.2016.353
164. Wang H, Wang Z, Li Y, Lu T, Hu G. Silencing Snail Reverses Epithelial-Mesenchymal Transition and Increases Radiosensitivity in Hypopharyngeal Carcinoma. Onco Targets Ther (2020) 13:497–511. doi: 10.2147/OTT.S237410
165. Liang H, Chen G, Li J, Yang F. Snail Expression Contributes to Temozolomide Resistance in Glioblastoma. Am J Transl Res (2019) 11:4277–89.
166. Wang H, Li JM, Wei W, Yang R, Chen D, Ma XD, et al. Regulation of ATP-Binding Cassette Subfamily B Member 1 by Snail Contributes to Chemoresistance in Colorectal Cancer. Cancer Sci (2020) 111:84–97. doi: 10.1111/cas.14253
167. Fan F, Samuel S, Evans KW, Lu J, Xia L, Zhou Y, et al. Overexpression of Snail Induces Epithelial-Mesenchymal Transition and a Cancer Stem Cell-Like Phenotype in Human Colorectal Cancer Cells. Cancer Med (2012) 1:5–16. doi: 10.1002/cam4.4
168. Li HM, Bi YR, Li Y, Fu R, Lv WC, Jiang N, et al. A Potent CBP/p300-Snail Interaction Inhibitor Suppresses Tumor Growth and Metastasis in Wild-Type P53-Expressing Cancer. Sci Adv (2020) 6:eaaw8500. doi: 10.1126/sciadv.aaw8500
169. Azmi AS, Bollig-Fischer A, Bao B, Park BJ, Lee SH, Yong-Song G, et al. Systems Analysis Reveals a Transcriptional Reversal of the Mesenchymal Phenotype Induced by SNAIL-Inhibitor GN-25. BMC Syst Biol (2013) 7:85. doi: 10.1186/1752-0509-7-85
Keywords: Snail1, EMT, signaling pathway, ubiquitination, methylation, acetylation, tumor immunity
Citation: Tang X, Sui X, Weng L and Liu Y (2021) SNAIL1: Linking Tumor Metastasis to Immune Evasion. Front. Immunol. 12:724200. doi: 10.3389/fimmu.2021.724200
Received: 12 June 2021; Accepted: 15 November 2021;
Published: 30 November 2021.
Edited by:
Qin Yan, Yale University, United StatesReviewed by:
Khaled Murshed, Hamad Medical Corporation, QatarDongdong Zhang, Institute of Biophysics (CAS), China
Copyright © 2021 Tang, Sui, Weng and Liu. This is an open-access article distributed under the terms of the Creative Commons Attribution License (CC BY). The use, distribution or reproduction in other forums is permitted, provided the original author(s) and the copyright owner(s) are credited and that the original publication in this journal is cited, in accordance with accepted academic practice. No use, distribution or reproduction is permitted which does not comply with these terms.
*Correspondence: Liang Weng, wengliang@csu.edu.cn; Yongshuo Liu, liuyongshuo@pku.edu.cn