- 1Department of Radiology, Shantou Central Hospital, Shantou, Guangdong, China
- 2Department of Radiology, Sun Yat-sen Memorial Hospital, Sun Yat-sen University, Guangzhou, Guangdong, China
- 3Department of Radiology, Meizhou People's Hospital, Meizhou, Guangdong, China
- 4Department of Pulmonary and Critical Care Medicine, Sun Yat-sen Memorial Hospital, Sun Yat-sen University, Guangzhou, Guangdong, China
- 5Department of Neurosurgery, Second Affiliated Hospital of Shantou University Medical College, Shantou, Guangdong, China
- 6School of Information Technology and Electrical Engineering, University of Queensland, Brisbane, QLD, Australia
- 7Department of Neurosurgery, Sun Yat-sen Memorial Hospital, Sun Yat-sen University, Guangzhou, Guangdong, China
Purpose: This study aims to investigate the glymphatic system activity changes in patients with mild traumatic brain injury (mTBI), particularly in MRI-negative patients, using analysis along the perivascular space (ALPS) technology.
Methods: A total of 161 mTBI patients (age: 15–92 years old) and 28 healthy controls (age: 15–84 years old) were included in this retrospective study. The mTBI patients were divided into MRI-negative and MRI-positive groups. ALPS index was calculated automatically using whole-brain T1-MPRAGE imaging and diffusion tensor imaging. The Student's t and chi-squared tests were performed to compare the ALPS index, age, gender, course of disease, and Glasgow Coma Scale (GCS) score between groups. Correlations among ALPS index, age, course of disease and GCS score were computed using Spearman's correlation analysis.
Results: Increased activity of the glymphatic system was suggested in mTBI patients based on ALPS index analysis, including the MRI-negative patients. There was a significant negative correlation between the ALPS index and age. In addition, a weak positive correlation between the ALPS index and course of disease was also observed. On the contrary, there was no significant correlation between the ALPS index and sex nor between the ALPS index and GCS score.
Conclusion: Our study demonstrated that the activity level of the glymphatic system was enhanced in mTBI patients, even when their brain MRI scans were negative. These findings may provide novel insights for understanding the pathophysiology of mild TBI.
Introduction
Traumatic brain injury is a major global health problem, with the highest mortality and disability rates among all traumas (1, 2). Moreover, the incidence rate continuously increases yearly. Among them, mild traumatic brain injury (mTBI) accounts for about 80–90% (3). About 420 million patients live with mTBI worldwide yearly (4).
The pathophysiological changes of brain tissue after mTBI have not yet been fully elucidated. MRI as a clinical detection method is important for understanding brain changes after mTBI; however, some patients can be entirely negative on traditional MRI images (5, 6). Recent studies have found that the glymphatic system may be involved in the pathophysiological process of traumatic brain injury (7–9). Altered expression of aquaporin 4 (AQP4), an essential component of the glymphatic system, was found in an animal model of traumatic brain injury (10). In AQP4 gene deletion mice, the glymphatic system dysfunction promotes post-traumatic neuroinflammation and exacerbates cognitive deficits after traumatic brain injury (11). Mounting evidence shows that the changes in glymphatic system function play an important role in the development of mTBI (12, 13), and imaging the glymphatic system in the brain is expected to become a new perspective in the study of mTBI (14, 15). However, evaluating the glymphatic system in human is limited by traditional imaging technologies.
The analysis along the perivascular space (ALPS) method has been proposed in recent years to detect the functional changes of the human glymphatic system non-invasively and indirectly based on diffusion imaging (16, 17). The ALPS refers to the diffusivity along the perivascular space after eliminating the influence of nerve fibers. Specifically, the ALPS index was calculated from the ratio of the diffusivity in three orthogonal directions in the periventricular white matter. Previous studies have demonstrated ALPS index as a potential biomarker for glymphatic system function, with a lower value indicating reduced activity (18, 19). In this study, we aim to investigate the glymphatic system activity changes in mTBI patients, particularly in MRI-negative patients, using the ALPS technology.
Materials and methods
Patients
Ethical approvals by the institutional review boards were obtained for this retrospective analysis, and the need to obtain informed consent was waived. From January 2017 to February 2022, 814 patients with traumatic brain injury who had undergone whole-brain diffusion tensor imaging (DTI) scans were analyzed retrospectively. The exclusion criteria were as follows: (1) patients with Glasgow coma scale (GCS) scores ≤ 12 (n = 589); (2) DTI data incomplete (n = 12); (4) patients with neoplasms (n = 8); (5) poor DTI image quality (n = 9); (6) patients younger than 15 years old (n = 43). Finally, 161 mTBI patients with GCS scores between 13 and 15 were included. Among them, 27 patients had negative features on the brain MRI images (namely MRI-negative), and 134 had positive features on the brain MRI images (namely MRI-positive). In addition, another 28 aged-matched healthy subjects without neurological diseases were recruited as controls during the same period. Figure 1 shows the patient recruitment pathway and the inclusion and exclusion criteria.
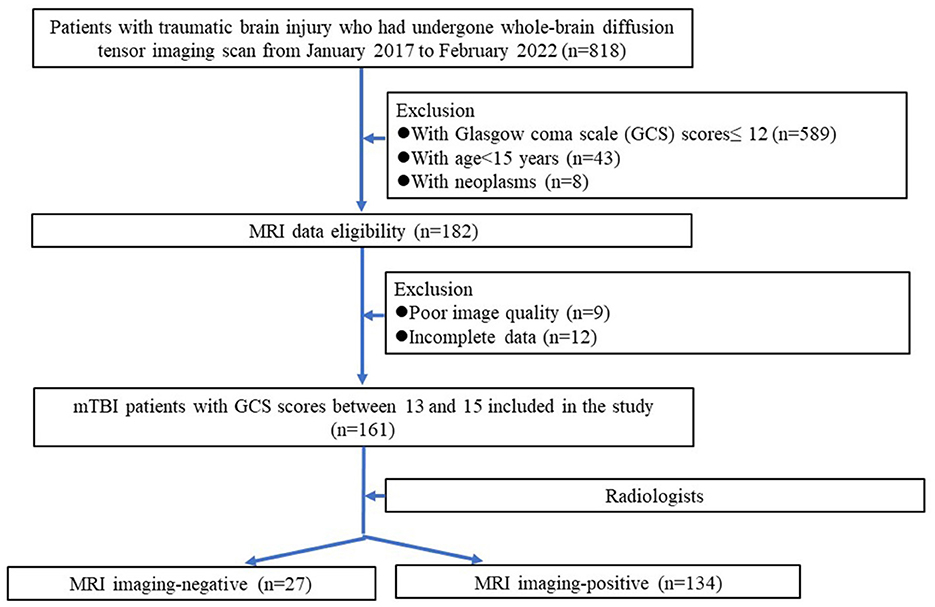
Figure 1. Flowchart of the mTBI patients selection procedure along with the inclusion and exclusion criteria.
MRI acquisition
The MRI data were acquired at a 3.0 T MR scanner (Magnetom Skyra, Siemens), comprising sagittal T1-MPRAGE and whole-brain DTI scans. The patient's head was immobilized with foam pillows inside the head coil to reduce the noise and diminish motion artifacts. DTI images were acquired using a single shot echo planar imaging sequence, with the following parameters: repetition time (TR) = 3,700 ms, echo time (TE) = 95 ms, FOV = 220 × 220 mm, flip angle = 90°, matrix size = 128 × 128 mm2, voxel size = 1.7 × 1.7 × 4.0 mm3, slice thickness = 4.0 mm, parallel acquisition technique (PAT) = 2, directions = 20, b values of 0 and 1,000 s/mm2. The sagittal T1-MPRAGE images were acquired with the following parameters: TR = 2,300 ms, TE = 2.26 ms, TI = 900 ms, FOV = 256 × 256 mm2, flip angle = 8°, matrix size = 256 × 256 mm2, voxel size = 0.5 × 0.5 × 0.5 mm3, slice thickness = 0.50 mm, PAT = 3.
ALPS analysis
To reduce subjective error, we devised an automated handler of FSL (version 5.0.9) to calculate the ALPS index. The processing flow chart was as follows. First, a glymphatic atlas was built. The Montreal Neurological Institute (MNI) 152 template and JHU-ICBM-Labels atlas were employed as an initial reference frame for alignment. Four 3 mm diameter spherical regions of interest (ROIs) were placed in the bilateral superior longitudinal fascicle (SLF) and superior corona radiata (SCR) according to the previously reported method (16). The MNI coordinates of the centers of the ROIs were set as (64,57,49), (26,57,49), (58,57,49), and (32,57,49). The ROIs were labeled separately and saved as a glymphatic atlas. Second, the individual fractional anisotropy (FA) maps were linearly registered to individual T1 anatomical maps, yielding a transformation matrix. Third, a linear plus non-linear approach was used to morph the individual T1 anatomical maps to MNI space. Fourth, the transformation relationships obtained in steps two and three were combined into a deformation field from the individual FA maps to MNI space. Finally, the deformation field was applied to each diffusion map, and the mean value of the signal was extracted for each ROI in the glymphatic atlas. Average values were taken for identical structures on both sides. The ALPS index was calculated as [(Dxxslf + Dxxscr)/(Dyyscr + Dzzslf)], where Dxxslf and Dxxscr were the x-axis diffusivity in the areas of SLF and SCR, Dyyscr was the y-axis diffusivity in the area of SCR, and Dzzslf was the z-axis diffusivity in the area of SLF.
Statistical analysis
Statistical analyses were performed using R software Version: 3.6.4 (http://www.r-project.org/) and SPSS software version 19 (IBM Corporation). The normality of the variables was evaluated using the Shapiro–Wilk test. The Student's t and chi-squared tests were performed to compare the ALPS index, age, gender, course of disease, and GCS score between the mTBI and healthy-control groups. Correlations among ALPS index, age, course of disease and GCS score were computed using Spearman's correlation analysis. Statistical significance was set at P-value <0.05 (two-tailed).
Result
Patient characteristics
A total of 161 mTBI patients aged 15–92 and 28 healthy controls aged 15–84 were included. Patient characteristics of healthy controls (HC) and mTBI groups are compared in Table 1. There was no statistically significant difference in the age between healthy controls and mTBI patients (P = 0.097). In contrast, there was a statistically significant difference in the ALPS index between healthy controls and mTBI patients (P = 0.003, Figure 2A). Further comparisons showed a significant difference in the young group (P = 0.001), but no difference in the middle-aged (P = 0.121) and elderly groups (P = 0.953) (Figure 2B). Besides, there was a statistically significant difference in sex between healthy controls and mTBI patients (P = 0.019).
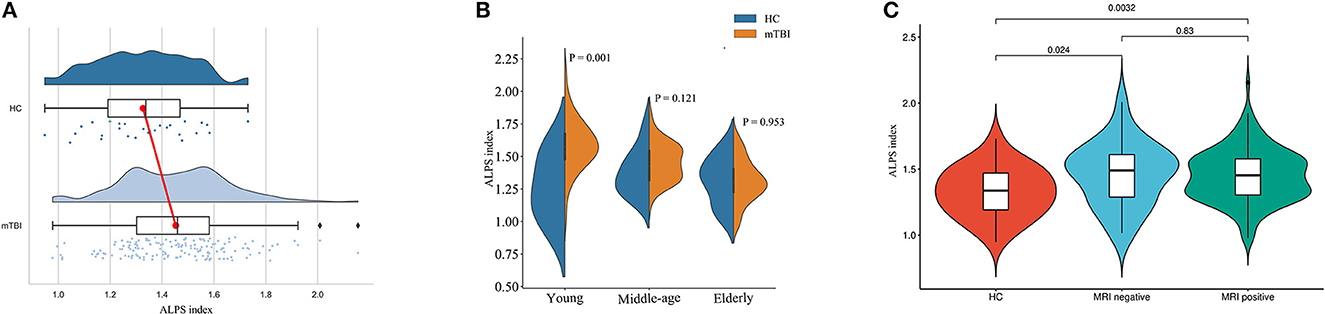
Figure 2. Comparison of ALPS indexes between different subject groups. (A) Raincloud plot depicting the ALPS index in each individual. Compared with healthy controls, the mTBI patients have a higher mean ALPS index. (B) The data were divided into three subgroups according to age stage, and the differences between mTBI patients and normal controls were compared. The difference was significant in the young group (P = 0.001), but not in either the middle-aged or the elderly group. (C) The ALPS indexes were higher in both MRI-negative and MRI-positive mTBI patients than in healthy controls (P = 0.024 and P = 0.003). There was no statistically significant difference in the ALPS index between mTBI patients with MRI-negative and MRI-positive (P = 0.830).
ALPS index between different subgroups
Of all 161 mTBI patients, 27 (16.7%) were MRI-negative, and 134 (83.2%) were MRI-positive (Table 2). Compared to Healthy controls, both MRI-negative and MRI-positive mTBI sub-groups had significantly higher ALPS indexes (Figure 2C), with P-values of 0.023 and 0.003, respectively. The mean ALPS index value of MRI-negative patients was slightly higher than that of MRI-positive patients, but the difference was not statistically significant (P = 0.808). Figure 3 illustrates the MRI-negative and MRI-positive manifestations from two mTBI patients with similar ALPS indexes.
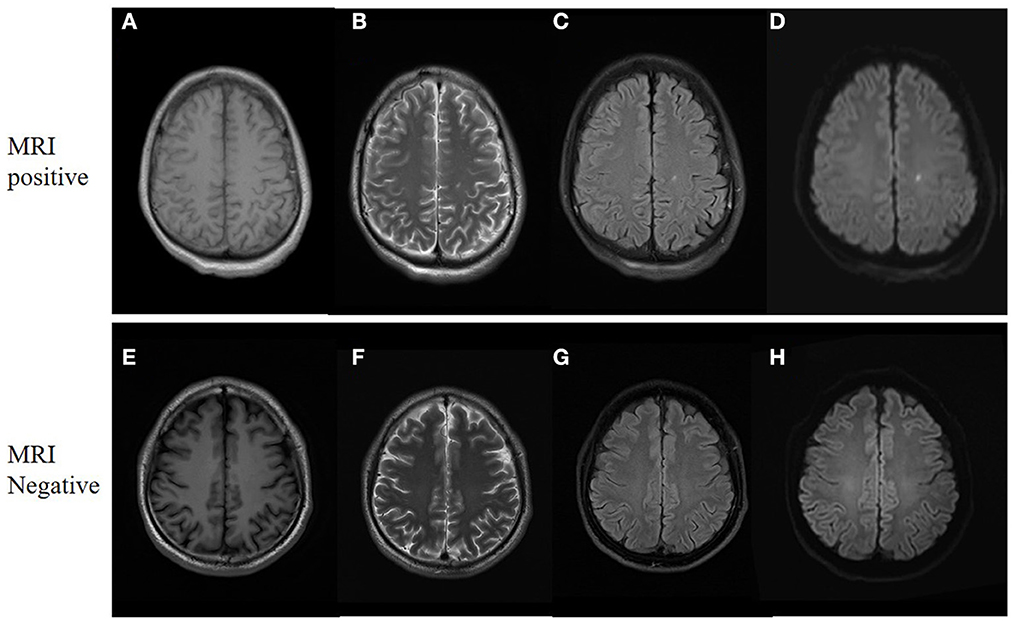
Figure 3. MRI manifestations of two mTBI patients. (A–D), a 31-year-old male with mTBI. Axial non-contrast MRI shows a small punctate contusion of the left corona radiata. The ALPS index is 1.45. (E–H), a 33-year-old male with mTBI. The MRI findings were negative, whereas the ALPS index is 1.46. (A, E) are T1 weighted images. (B, F) are T2 weighted images. (C, G) are T2-Flair images. (D, H) are diffusion weighted images.
Correlations of ALPS index with age, course of disease, and GCS score
There was a significant negative correlation between the ALPS index and age (r = −0.61, P < 0.001, Figure 4A) and also a weak positive correlation between the ALPS index and course of disease (r = 0.19, P = 0.016, Figure 4B). In contrast, there was no significant correlation between the ALPS index and sex (r = −0.10, P = 0.161) nor between the ALPS index and GCS score (r = −0.02, P = 0.842).
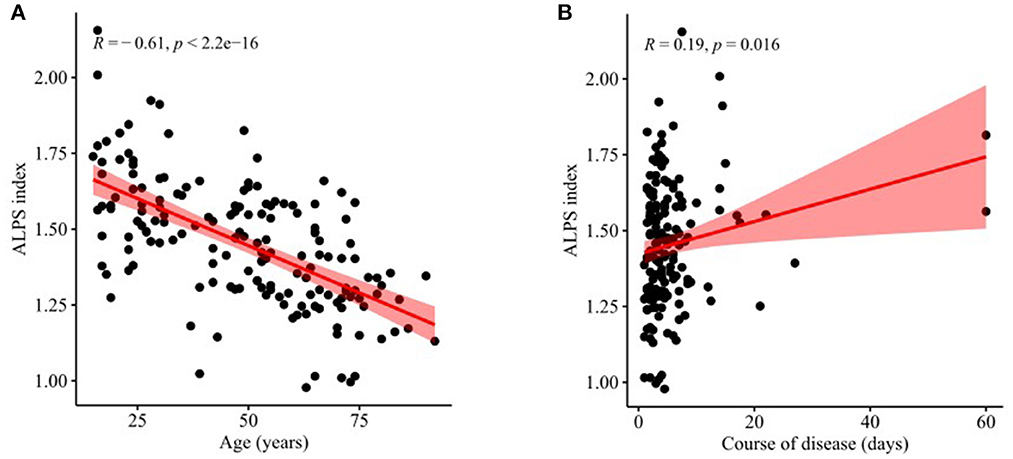
Figure 4. Correlations of ALPS index with age and course of disease. (A) There was a significant negative correlation between the ALPS index and age (r = −0.61, P < 0.001). (B) There was a weak positive correlation between the ALPS index and course of disease (r = 0.19, P = 0.016).
Discussion
In this study, we demonstrated that in 161 mTBI patients aged 15–90 s, the activity of the glymphatic system function seemed to be enhanced compared with healthy controls, indicated by the ALPS index. This enhancement was more pronounced at younger ages. Notably, of about seventeen percent of mTBI patients who showed negative brain MRI, changes in the glymphatic system function were detected using the ALPS method. Furthermore, there was a significant negative correlation between the ALPS index and age. A weak positive correlation between the ALPS index and course of disease was also reported. In contrast, there was no significant correlation between the ALPS index and sex nor between the ALPS index and GCS score.
The effect of mTBI on the glymphatic system of the brain is still inconclusive. We found that the activity of the glymphatic system suggested to be increased in patients with mTBI, which might be related to several factors. First, the increased expression of AQP4 after trauma, as previous studies evaluated by western blot or immunohistochemical staining, have been observed in animal models (10, 20, 21). The AQP4, as part of the glymphatic system, has been shown to support perivascular fluid and solute movement (22, 23). In contrast, the dysregulation of AQP4 in gene-knockout mice might inhibit glymphatic system function and exacerbate trauma progression (11). Second, changes in glymphatic system function might be related to hemodynamic fluctuations, as reported in an animal study (24). In a repetitive mTBI model, the glymphatic influx was demonstrated to be increased, whereas the efflux was slower (13). Third, since the glymphatic system plays a crucial role in transporting biomarkers of traumatic brain injury (25, 26), the enhancement might be a compensating mechanism to reduce secondary damage by facilitating the elimination of endotoxic products. It is worth noting that our result was present in mTBI patients, whereas impairment of the glymphatic system could occur in different trauma groups (27–29). Since different studies used trauma of varying severity, and the research objects were focused on young subjects without the elderly, it was difficult to represent the overall population of the disease. The broad age range of patients in this study would better represent the mTBI patient population. Interestingly, our results suggested that the effect of mTBI on the glymphatic system was more pronounced at younger ages, which might be related to the previously published aging of the glymphatic system (30). However, patients with moderate or severe traumatic brain injury may have variable outcomes concerning glymphatic system function.
Another important finding was that changes in the function of the glymphatic system could be detected in patients with negative brain MRIs. Although neuroimaging is an important detection modality for mTBI, many patients still have negative results in standard structural imaging (31, 32). Previous studies have suggested that this is due to the neuropathological changes in these patients being functional impairment rather than structural damage (33). Significant advances in neuroimaging have recently made it possible to study functional abnormalities in mTBI using a variety of methods (34–36). The technology used in this study is based on diffusion imaging non-invasively. Through calculation models to eliminate the interference of fibers in the brain, changes in the diffusivity of the perivascular space can be obtained, representing the activity level of the glymphatic system (16, 17, 37). However, ALPS indexes were conventionally calculated manually, which might introduce subjective error and depend on examiner expertise. To solve the above problem, we proposed an automated processing program in this study to ensure the objectivity of the ALPS index. In contrast, GCS scores are still assessed subjectively and the subjective error might be one of the reasons for the lack of correlation between the two indicators.
As expected, there was a significant negative correlation between the ALPS index and age. According to previous animal experiments, the glymphatic system might be hypo-functioning due to a general decline in cerebrospinal fluid-interstitial fluid exchange in the elder (38–40). This decline was associated with decreased CSF production and arterial pulsatility, which affected glymphatic influx (41–43). In addition, a recent study found that aging mice had impaired meningeal lymphatic function, which might also contribute to glymphatic dysfunction (44). Therefore, the treatment of elderly patients needs to be different from that of younger patients. For elderly patients with mTBI, appropriate treatment to improve cerebral circulation, maintain the activity of the glymphatic system without excessive dehydration, and promote the elimination of endotoxic products might improve the progression of mTBI.
There are several limitations of this study. First, although our cohort was focused on mTBI, there was a substantial difference among etiologies. Therefore, the results may differ from animal models with a single etiology. Second, the sleep status of the study participants was not recorded, which is considered critical for regulating the glymphatic system and deserves analysis in future prospective studies. Third, there was no pathological validation since it was a patient study. Detailed mechanism studies are needed in further experiments.
Conclusion
Our study demonstrated that the activity level of the glymphatic system was enhanced in mTBI patients, including MRI-negative patients. Moreover, there was a significant negative correlation between the glymphatic system function and age. These findings may provide novel insights for understanding the pathophysiology of mild TBI.
Data availability statement
The raw data supporting the conclusions of this article will be made available by the authors, without undue reservation.
Ethics statement
The studies involving human participants were reviewed and approved by Meizhou People's Hospital. Written informed consent from the participants' legal guardian/next of kin was not required to participate in this study in accordance with the national legislation and the institutional requirements.
Author contributions
ZD, ZY, ZL, ML, and XW: concept and design. ZD, ZY, ZL, ML, HS, ZZ, WY, and ZH: drafting of the manuscript. ZD, ML, HS, XC, DL, and XW: critical revision of the manuscript for important intellectual content. ZY, ZL, HS, and XC: statistical analysis. ZD, ZY, ZL, ML, XC, DL, and XW: administrative, technical, and material support. All authors agreed to be accountable for the content of the work. All authors: acquisition, analysis, and interpretation of data. All authors contributed to the article and approved the submitted version.
Funding
This study was supported in part by grants from the National Natural Science Foundation of China (82101985), Natural Science Foundation of Guangdong Province (2021A1515011179), and Medical Scientific Foundation of Guangdong Province (B2021280).
Conflict of interest
The authors declare that the research was conducted in the absence of any commercial or financial relationships that could be construed as a potential conflict of interest.
Publisher's note
All claims expressed in this article are solely those of the authors and do not necessarily represent those of their affiliated organizations, or those of the publisher, the editors and the reviewers. Any product that may be evaluated in this article, or claim that may be made by its manufacturer, is not guaranteed or endorsed by the publisher.
References
1. Daugherty J, Waltzman D, Sarmiento K, Xu L. Traumatic brain injury-related deaths by race/ethnicity, sex, intent, and mechanism of injury - United States, 2000-2017. MMWR Morb Mortal Wkly Rep. (2019) 68:1050–6. doi: 10.15585/mmwr.mm6846a2
2. Maas AIR, Menon DK, Manley GT, Abrams M, Åkerlund C, Andelic N, et al. Traumatic brain injury: progress and challenges in prevention, clinical care, and research. Lancet Neurol. (2022) 21:1004–60. doi: 10.1016/S1474-4422(22)00309-X
3. Levin HS, Diaz-Arrastia RR. Diagnosis, prognosis, and clinical management of mild traumatic brain injury. Lancet Neurol. (2015) 14:506–17. doi: 10.1016/S1474-4422(15)00002-2
4. Gardner RC, Yaffe K. Epidemiology of mild traumatic brain injury and neurodegenerative disease. Mol Cell Neurosci. (2015) 66:75–80. doi: 10.1016/j.mcn.2015.03.001
5. Huang Y-L, Kuo Y-S, Tseng Y-C, Chen DY-T, Chiu W-T, Chen C-J. Susceptibility-weighted MRI in mild traumatic brain injury. Neurology. (2015) 84:580. doi: 10.1212/WNL.0000000000001237
6. Van Der Naalt J, Jacobs B. Highlights mild traumatic brain injury 2021. Curr Opin Anesthesiol. (2022) 35. doi: 10.1097/ACO.0000000000001177
7. Doustar J, Danan IJ. Glymphatic system dysfunction in mild traumatic brain injury. Neurology. (2022) 98:S24. doi: 10.1212/01.wnl.0000801968.87371.4e
8. Ferrara M, Bertozzi G, Volonnino G, Di Fazio N, Frati P, Cipolloni L, et al. Glymphatic system a window on tbi pathophysiology: a systematic review. Int J Mol Sci. (2022) 23:9138. doi: 10.3390/ijms23169138
9. Piantino JA, Iliff JJ, Lim MM. The bidirectional link between sleep disturbances and traumatic brain injury symptoms: a role for glymphatic dysfunction? Biol Psychiatry. (2022) 91:478–87. doi: 10.1016/j.biopsych.2021.06.025
10. Ren Z, Iliff JJ, Yang L, Yang J, Chen X, Chen MJ, et al. ‘Hit & Run' model of closed-skull traumatic brain injury (TBI) reveals complex patterns of post-traumatic AQP4 dysregulation. J Cerebr Blood Flow Metab. (2013) 33:834–45. doi: 10.1038/jcbfm.2013.30
11. Iliff JJ, Chen MJ, Plog BA, Zeppenfeld DM, Soltero M, Yang L, et al. Impairment of glymphatic pathway function promotes tau pathology after traumatic brain injury. J Neurosci. (2014) 34:16180. doi: 10.1523/JNEUROSCI.3020-14.2014
12. Bolte AC, Dutta AB, Hurt ME, Smirnov I, Kovacs MA, Mckee CA, et al. Meningeal lymphatic dysfunction exacerbates traumatic brain injury pathogenesis. Nat Commun. (2020) 11:4524. doi: 10.1038/s41467-020-18113-4
13. Christensen J, Wright DK, Yamakawa GR, Shultz SR, Mychasiuk R. Repetitive mild traumatic brain injury alters glymphatic clearance rates in limbic structures of adolescent female rats. Sci Rep. (2020) 10:6254. doi: 10.1038/s41598-020-63022-7
14. Rasmussen MK, Mestre H, Nedergaard M. The glymphatic pathway in neurological disorders. Lancet Neurol. (2018) 17:1016–24. doi: 10.1016/S1474-4422(18)30318-1
15. Nedergaard M, Goldman SA. Glymphatic failure as a final common pathway to dementia. Science. (2020) 370:50–6. doi: 10.1126/science.abb8739
16. Taoka T, Masutani Y, Kawai H, Nakane T, Matsuoka K, Yasuno F, et al. Evaluation of glymphatic system activity with the diffusion MR technique: diffusion tensor image analysis along the perivascular space (DTI-ALPS) in Alzheimer's disease cases. Jpn J Radiol. (2017) 35:172–8. doi: 10.1007/s11604-017-0617-z
17. Siow TY, Toh CH, Hsu J-L, Liu G-H, Lee S-H, Chen N-H, et al. Association of sleep, neuropsychological performance, and gray matter volume with glymphatic function in community-dwelling older adults. Neurology. (2022) 98:e829. doi: 10.1212/WNL.0000000000201080
18. Bae YJ, Choi BS, Kim J-M, Choi J-H, Cho SJ, Kim JH. Altered glymphatic system in idiopathic normal pressure hydrocephalus. Parkinsonism Relat Disord. (2021) 82:56–60. doi: 10.1016/j.parkreldis.2020.11.009
19. Chen H-L, Chen P-C, Lu C-H, Tsai N-W, Yu C-C, Chou K-H, et al. Associations among cognitive functions, plasma DNA, and diffusion tensor image along the perivascular space (DTI-ALPS) in patients with Parkinson's Disease. Oxid Med Cell Longev. (2021) 2021:4034509. doi: 10.1155/2021/4034509
20. Tomura S, Nawashiro H, Otani N, Uozumi Y, Toyooka T, Ohsumi A, et al. Effect of decompressive craniectomy on aquaporin-4 expression after lateral fluid percussion injury in rats. J Neurotrauma. (2010) 28:237–43. doi: 10.1089/neu.2010.1443
21. Higashida T, Kreipke CW, Rafols JA, Peng C, Schafer S, Schafer P, et al. The role of hypoxia-inducible factor-1α, aquaporin-4, and matrix metalloproteinase-9 in blood-brain barrier disruption and brain edema after traumatic brain injury: Laboratory investigation. J Neurosurg. (2011) 114:92–101. doi: 10.3171/2010.6.JNS10207
22. Nedergaard M. Garbage truck of the brain. Science. (2013) 340:1529–30. doi: 10.1126/science.1240514
23. Mestre H, Hablitz LM, Xavier AL, Feng W, Zou W, Pu T, et al. Aquaporin-4-dependent glymphatic solute transport in the rodent brain. Elife. (2018) 7:e40070. doi: 10.7554/eLife.40070.022
24. Hayward NM, Tuunanen PI, Immonen R, Ndode-Ekane XE, Pitkänen A, Gröhn O. Magnetic resonance imaging of regional hemodynamic and cerebrovascular recovery after lateral fluid-percussion brain injury in rats. J Cereb Blood Flow Metab. (2011) 31:166–77. doi: 10.1038/jcbfm.2010.67
25. Jessen NA, Munk ASF, Lundgaard I, Nedergaard M. The glymphatic system: a beginner's guide. Neurochem Res. (2015) 40:2583–99. doi: 10.1007/s11064-015-1581-6
26. Plog BA, Dashnaw ML, Hitomi E, Peng W, Liao Y, Lou N, et al. Biomarkers of traumatic injury are transported from brain to blood via the glymphatic system. J Neurosci. (2015) 35:518–26. doi: 10.1523/JNEUROSCI.3742-14.2015
27. Li L, Chopp M, Ding G, Davoodi-Bojd E, Zhang L, Li Q, et al. MRI detection of impairment of glymphatic function in rat after mild traumatic brain injury. Brain Res. (2020) 1747:147062. doi: 10.1016/j.brainres.2020.147062
28. Zhang E, Wan X, Yang L, Wang D, Chen Z, Chen Y, et al. Omega-3 polyunsaturated fatty acids alleviate traumatic brain injury by regulating the glymphatic pathway in mice. Front Neurol. (2020) 11:707. doi: 10.3389/fneur.2020.00707
29. Park JH, Bae YJ, Kim JS, Jung WS, Choi JW, Roh TH, et al. (2022). Glymphatic system evaluation using diffusion tensor imaging in patients with traumatic brain injury. Neuroradiology. 65:551–7. doi: 10.1007/s00234-022-03073-x
30. Dai Z, Yang Z, Chen X, Zheng W, Zhuang Z, Liao Y, et al. The aging of glymphatic system in human brain and its correlation with brain charts and neuropsychological functioning. Cereb Cortex. (2023). doi: 10.1093/cercor/bhad086
31. Yue JK, Vassar MJ, Lingsma HF, Cooper SR, Okonkwo DO, Valadka AB, et al. Transforming research and clinical knowledge in traumatic brain injury pilot: multicenter implementation of the common data elements for traumatic brain injury. J Neurotrauma. (2013) 30:1831–44. doi: 10.1089/neu.2013.2970
32. Bigler ED, Abildskov TJ, Goodrich-Hunsaker NJ, Black G, Christensen ZP, Huff T, et al. Structural neuroimaging findings in mild traumatic brain injury. Sports Med Arthrosc. (2016) 24:e42–52. doi: 10.1097/JSA.0000000000000119
33. Mccrory P, Meeuwisse WH, Aubry M, Cantu RC, Dvorák J, Echemendia RJ, et al. Consensus statement on concussion in sport: The 4th International Conference on Concussion in Sport, Zurich, November 2012. J Athl Train. (2013) 48:554–75. doi: 10.4085/1062-6050-48.4.05
34. Wilde EA, Bouix S, Tate DF, Lin AP, Newsome MR, Taylor BA, et al. Advanced neuroimaging applied to veterans and service personnel with traumatic brain injury: state of the art and potential benefits. Brain Imaging Behav. (2015) 9:367–402. doi: 10.1007/s11682-015-9444-y
35. Wintermark M, Coombs L, Druzgal TJ, Field AS, Filippi CG, Hicks R, et al. Traumatic brain injury imaging research roadmap. Am J Neuroradiol. (2015) 36:E12. doi: 10.3174/ajnr.A4254
36. Zhuang Z, Shen Z, Chen Y, Dai Z, Zhang X, Mao Y, et al. Mapping the changes of glutamate using glutamate chemical exchange saturation transfer (GluCEST) technique in a traumatic brain injury model: a longitudinal pilot study. ACS Chem Neurosci. (2019) 10:649–57. doi: 10.1021/acschemneuro.8b00482
37. Zhou W, Shen B, Shen WQ, Chen H, Zheng YF, Fei JJ. Dysfunction of the glymphatic system might be related to iron deposition in the normal aging brain. Front Aging Neurosci. (2020) 12:559603. doi: 10.3389/fnagi.2020.559603
38. Kress BT, Iliff JJ, Xia M, Wang M, Wei HS, Zeppenfeld D, et al. Impairment of paravascular clearance pathways in the aging brain. Ann Neurol. (2014) 76:845–61. doi: 10.1002/ana.24271
39. Yue X, Mei Y, Zhang Y, Tong Z, Cui D, Yang J, et al. New insight into Alzheimer's disease: light reverses Aβ-obstructed interstitial fluid flow and ameliorates memory decline in APP/PS1 mice. Alzheimers Dement. (2019) 5:671–84. doi: 10.1016/j.trci.2019.09.007
40. Shetty AK, Zanirati G. The interstitial system of the brain in health and disease. Aging Dis. (2020) 11:200–11. doi: 10.14336/AD.2020.0103
41. Lee KJ, Jung KH, Park CY, Kim JM, Lee ST, Chu K, et al. Increased arterial pulsatility and progression of single subcortical infarction. Eur Radiol. (2017) 27:899–906. doi: 10.1007/s00330-016-4486-0
42. Mestre H, Mori Y, Nedergaard M. The brain's glymphatic system: current controversies. Trends Neurosci. (2020) 43:458–66. doi: 10.1016/j.tins.2020.04.003
43. Silva I, Silva J, Ferreira R, Trigo D. Glymphatic system, AQP4, and their implications in Alzheimer's disease. Neurol Res Pract. (2021) 3:5. doi: 10.1186/s42466-021-00102-7
Keywords: mild traumatic brain injury, glymphatic system, human, magnetic resonance imaging, in vivo
Citation: Dai Z, Yang Z, Li Z, Li M, Sun H, Zhuang Z, Yang W, Hu Z, Chen X, Lin D and Wu X (2023) Increased glymphatic system activity in patients with mild traumatic brain injury. Front. Neurol. 14:1148878. doi: 10.3389/fneur.2023.1148878
Received: 02 February 2023; Accepted: 24 April 2023;
Published: 12 May 2023.
Edited by:
Govind Nair, National Institutes of Health (NIH), United StatesReviewed by:
James Q. Truong, United States Army Public Health Command, United StatesToshiaki Taoka, Nagoya University, Japan
Copyright © 2023 Dai, Yang, Li, Li, Sun, Zhuang, Yang, Hu, Chen, Lin and Wu. This is an open-access article distributed under the terms of the Creative Commons Attribution License (CC BY). The use, distribution or reproduction in other forums is permitted, provided the original author(s) and the copyright owner(s) are credited and that the original publication in this journal is cited, in accordance with accepted academic practice. No use, distribution or reproduction is permitted which does not comply with these terms.
*Correspondence: Xiaofeng Chen, 15766214509@163.com; Daiying Lin, lindaiying917@163.com; Xianheng Wu, wuxh68@163.com
†These authors have contributed equally to this work and share first authorship