- 1Department of Neurosurgery and Department of Biomedical and Clinical Sciences, Linköping University, Linköping, Sweden
- 2Department of Clinical Chemistry and Clinical Pharmacology and Department of Biomedical and Clinical Sciences, Linköping University, Linköping, Sweden
- 3Department of Infectious Diseases, and Department of Biomedical and Clinical Sciences, Linköping University, Linköping, Sweden
- 4Department of Infectious Diseases, Kalmar County Hospital, Kalmar, Sweden
Streptococcus intermedius occasionally causes brain abscesses that can be life-threatening, requiring prompt antibiotic and neurosurgical treatment. The source is often dental, and it may spread to the eye or the brain parenchyma. We report the case of a 34-year-old man with signs of apical periodontitis, endophthalmitis, and multiple brain abscesses caused by Streptococcus intermedius. Initial treatment with meropenem and vancomycin was unsuccessful due to subtherapeutic concentrations, despite recommended dosages. Adequate concentrations could be reached only after increasing the dose of meropenem to 16 g/day and vancomycin to 1.5 g × 4. The patient exhibited high creatinine clearance consistent with augmented renal clearance, although iohexol and cystatin C clearances were normal. Plasma free vancomycin clearance followed that of creatinine. A one-day dose of trimethoprim–sulfamethoxazole led to an increase in serum creatinine and a decrease in both creatinine and urea clearances. These results indicate that increased tubular secretion of the drugs was the cause of suboptimal antibiotic treatment. The patient eventually recovered, but his left eye needed enucleation. Our case illustrates that augmented renal clearance can jeopardize the treatment of serious bacterial infections and that high doses of antibiotics are needed to achieve therapeutic concentrations in such cases. The mechanisms for regulation of kidney tubular transporters of creatinine, urea, vancomycin, and meropenem in critically ill patients are discussed.
Introduction
Augmented renal clearance (ARC), defined as a measured creatinine clearance >130 ml/min/1.73 m2, is increasingly being recognized in critically ill patients. The condition can lead to subtherapeutic concentrations of renally excreted drugs. The case-patient presented with low serum creatinine concentrations and high creatinine clearance, leading to the diagnosis of ARC. The pathophysiology of ARC is unknown, but high cardiac output, increased tubular creatinine secretion, and hormonal effects have been suggested. ARC may jeopardize the treatment of life-threatening deep bacterial infections with, for instance, members of the Streptococcus anginosus group. We report a patient with S. intermedius infection, endogenous endophthalmitis, and septic embolization to the CNS, arising from a dental infection. Increased drug elimination due to ARC resulted in subtherapeutic antibiotic drug concentrations until high doses were administered. The purpose of this case report is to illustrate the possible causes for rapid elimination of the drugs.
Case Description
A 34-year-old man presented at the emergency room with 1-day history of headache, fever, photosensitivity, and visual impairment. On examination, he was awake but slightly disoriented (Glasgow Coma Scale 14). The following were observed: CRP: 50 mg/L, lumbar puncture: 25 cm H2O opening pressure (Lee and Lueck, 2014), CSF: white cell count of 7210 × 106/L (6380 × 106/L polymorphonuclear), lactate 8.2 mmol/L, and albumin 880 mg/L. Meningitis was diagnosed and treatment started with meropenem 2 g × 3, acyclovir 800 mg × 3, and betamethasone 8 mg × 4. CT brain showed no sign of abscess or empyema; bilateral diffuse low-density areas were observed in the frontal and temporal lobes, indicating edema (Figure 1A). Methods, reference values, and drug target values are given in Table 1.
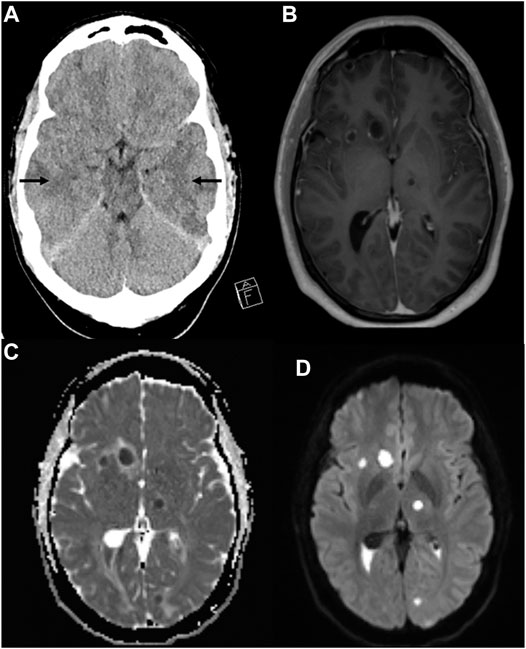
FIGURE 1. Computer tomography showing hypodense areas bilaterally in the temporal lobes indicating edema (arrows) (A). MRI of the brain with T1WI with gadolinium contrast (B), apparent diffusion coefficient (ADC) image (C), and diffusion-weighted imaging (DWI) (D). Multiple peripherally contrast-enhancing lesions with perilesional edema were found both supra- and infratentorial (B). A thin ependymal contrast enhancement is found. Diffusion imaging (C,D) show reduced signaling in the parenchymal lesions and in the content of the posterior horns, indicating multiple abscesses and ventriculitis.
His visual impairment worsened, perceiving only light and dark with his left eye. On day 7, MRI with contrast enhancement revealed multiple brain abscesses (Figures 1B–D) and reduced diffusion, indicating ventriculitis. Reduced diffusion was also observed in the retina of the left eye, suggesting abscess and retinal detachment.
The patient was transferred to the university hospital’s neurosurgical intensive care unit. CSF cultures were negative for bacteria, fungi, and neurotropic viruses. The QuantiFERON test and the tests for HIV and toxoplasmosis were also negative. The echocardiography indicated no endocarditis or other abnormality. The MRI showed no signs of thrombosis in the jugular vein, indicative of Lemierre’s syndrome. The intravitreal and subconjunctival injections of vancomycin and ceftazidime, as well as ocular dexamethasone and cyclopentolate, were administered. A puncture of a subcortical abscess in the right frontal lobe and the placement of an external ventricular drainage were performed.
Day 13 dental examination showed apical periodontitis requiring extraction of one mandibular and three maxillary teeth was identified. Two days later, surgical extirpation of a right frontal cortical abscess was performed. No bacterial growth was seen in cultures, but the material from the brain abscess was sent for 16S rRNA sequencing analysis. S. intermedius was detected and confirmed by PCR analysis. The CSF profiles improved, and day 21 MRI showed slight regression of ventriculitis and some abscesses. Intrathecal antibiotics were discontinued, and the ventricular drainage was removed. Ophthalmological examination showed almost complete retinal detachment with rupture. The eye was beyond help and thus enucleated. The patient was discharged after 6 weeks. Fourteen weeks after admission, the patient still acknowledged cognitive and memory impairments; fatigue; changed sense of smell, sound sensitivity, and photosensitivity; recurrent nightmares; and impaired motor function.
Antibiotic Treatment
Initial treatment was meropenem 2 g × 3 and acyclovir 800 mg × 3. This was changed on day 7 to cefotaxime and metronidazole following the finding of multiple intracerebral abscesses (Table 2). To broaden antibacterial coverage, this was reversed the following day to meropenem and metronidazole, with the addition of intravenous vancomycin, and from day 9, intrathecal vancomycin was administered. On suspicion of toxoplasmosis, he received trimethoprim–sulfamethoxazole (TMP-SMX) on day 11, but this was replaced by rifampicin on day 12.
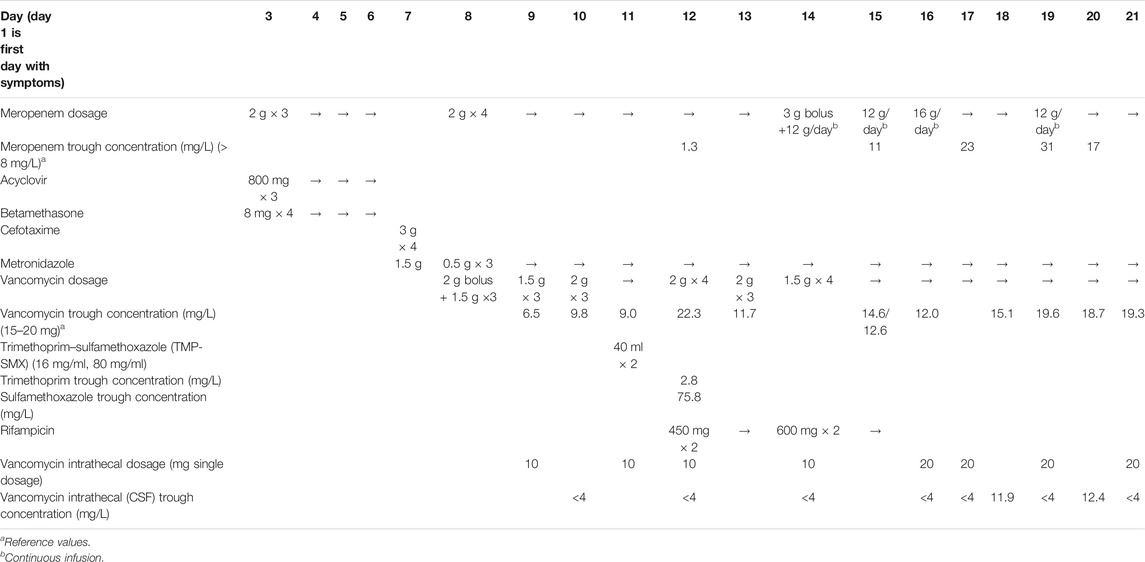
TABLE 2. Antibiotic treatment and drug concentrations in a neurosurgery patient with augmented renal clearance and multiple abscesses in the brain due to S. intermedius infection.
Despite doses ranging from 1.5 g × 3 to 2 g × 4, a stable vancomycin target level of 15–20 mg/L (Rybak et al., 2020) was not achieved until 1.5 g × 4 was given (Table 2). Serum and CSF antibiotic concentrations remained undetectable or subtherapeutic until doses higher than those recommended for meningitis were administered.
Research Investigations
Our patient participated in a prospective observational study on the pharmacokinetics of vancomycin in intensive care patients. Vancomycin clearance was determined over a period of 5 days on three occasions, and iohexol clearance on two (Figure 2). The period included the day when TMP–SMX was administered. ARC was diagnosed since creatinine clearance was above 130 ml/min/1.73 m2 apart from the days immediately following TMP–SMX treatment. Urea clearance was initially 86% above the upper reference limit (Koch et al., 1980) and followed the same pattern as creatinine clearance (Figure 2). However, iohexol and cystatin C clearances were normal.
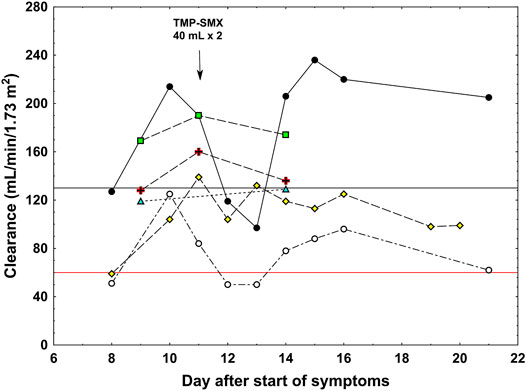
FIGURE 2. Clearance values in a patient with augmented renal clearance. Black circles: measured creatinine clearance, white circles: measured urea clearance, green triangles: iohexol clearance, yellow rhomboids: cystatin C clearance, red cross: total vancomycin clearance, green squares: free vancomycin clearance. The black horizontal line shows the limit for augmented renal clearance (130 ml/min/1.73 m2) and the red horizontal line shows the upper normal reference for urea clearance (60 ml/min/1.73 m2). Arrow shows the dosage of trimethoprim–sulfamethoxazole (TMP-SMX).
Vancomycin clearance calculated from total plasma concentrations was higher than that of iohexol, cystatin C, and creatinine clearances after trimethoprim treatment (Figure 2). The clearance of free vancomycin was even higher, approaching that of creatinine during ARC, indicating that vancomycin underwent both glomerular filtration and tubular secretion. Unfortunately, the total and free vancomycin clearances were not determined each day after TMP–SMX administration, precluding the conclusion regarding the effect of trimethoprim on the systems responsible for the tubular transport of vancomycin.
Discussion
Clinical Observations
The incidence of brain abscess is 0.3–1.3 per 100,000 people per year, 70% being male with an average age of 34 years (Brouwer et al., 2014a). The pathophysiology of brain abscess varies, with pathogens invading the CNS directly or via the bloodstream. The spread from dental infection is usually direct, causing abscesses in the frontal area. Symptoms are often non-specific, but headache is prominent, and only 50% have fever. Altered consciousness and signs of elevated intracranial pressure may occur. Brain abscesses of dental origin are often caused by mixed infections, for example, anaerobic Gram-positive cocci, and aerobic staphylococci and streptococci (Brouwer et al., 2014b). In this case, S. intermedius, a common commensal in the oral cavity, was detected in samples from the abscess material, as in a recent study reporting the predominance of this agent in brain abscesses (Darlow et al., 2020). The broad-spectrum β-lactam antibiotic meropenem, with good CNS penetration, was given, but despite this, endogenous endophthalmitis was diagnosed a week after admission. A recent case report described how S. constellatus of dental origin caused endophthalmitis and brain abscesses (Chheda et al., 2011), and endogenous endophthalmitis caused by S. intermedius has been described after routine dental practice (Mali et al., 2015).
The trough values of vancomycin and meropenem were initially far below their suggested therapeutic ranges, although the patient received recommended doses (Table 2), and clearances of creatinine, urea, and free vancomycin were high (Figure 2). The patient’s BMI was 30.9 kg/m2 (height 197 cm, weight 120 kg), with a body surface area of 2.55 m2, but his serum creatinine the first four days after admission was remarkably low [49, 44, 42, and 41 µmol/L (0.55, 0.49, 0.48, and 0.46 mg/dl)]. Despite his serious infection, sarcopenia was judged not responsible as he was not bedridden and sat in a chair during daytime. On treatment with trimethoprim, a well-known inhibitor of tubular creatinine transport (Berglund et al., 1975), creatinine clearance normalized. Apart from the days immediately following TMP–SMX treatment, creatinine clearance was around 70% higher than iohexol and cystatin C, indicating substantial tubular excretion of creatinine (Figure 2). Total vancomycin clearance was around 45% higher than iohexol and cystatin C clearances, and clearance of free vancomycin was even higher at around 90% of that of creatinine. The high clearance of vancomycin and demand for high doses of both vancomycin and meropenem clearly indicated high tubular secretion of these two drugs. Lonsdale et al. (2013) reported a similar case. This case and our case illustrate the phenomenon of contemporary rapid elimination of vancomycin and meropenem in neurosurgical patients with ARC (Lin Wu et al., 2015).
Tubular Cell Transport of Creatinine, Urea, Vancomycin, and Meropenem
The literature regarding the tubular transport of creatinine is extensive (Wang and Kestenbaum, 2018). Creatinine is largely cleared by glomerular filtration, and normally around 10% is actively excreted by tubular transport. In the steady state, the excretion matches muscle production. In humans, creatinine is transported across the basolateral membrane of the proximal tubule by the human organic cation transporter-2 (hOCT2) (Urakami et al., 2004; Motohashi and Inui, 2013). The antiport transporters MATE1 and MATE2-K regulate its transport across the apical membrane into the tubular lumen. Trimethoprim is a known inhibitor of this transport system; hence, the temporary reduction in creatinine clearance to normal after trimethoprim treatment clearly shows that tubular creatinine transport was upregulated in this case (Figure 2).
Urea is also freely filtered in the glomeruli, but the concentrations along the tubular fluid transport system vary greatly due to resorption of water and removal of urea by transporters in the inner medullary collecting duct cells. The urea transporter UT-A1, at the apical membrane, transports urea from the lumen into the cell, while UT-A3 transports urea across the basal membrane to the interstitium. The final elimination of urea in the urine is around 30–50% of the glomerular filtrate in mammals (Klein et al., 2011). Surprisingly, urea clearance was also affected by treatment with trimethoprim in a similar way as creatinine clearance (Figure 2). The significance of this is unclear and, to our knowledge, has not been reported previously.
Drug transport within the tubular cells is the first fundamental stage in the onset of the nephrotoxic process. Knowledge of these concepts is important for the prevention of iatrogenic kidney damage, particularly in patients with underlying disease receiving concomitant treatment with several potentially nephrotoxic drugs (Fanos and Cataldi, 2001). In experimental models of nephrotoxicity, vancomycin crosses the basolateral membrane of the proximal tubular epithelium via the OCT system (Sokol, 1991). The involvement of MATE1 and MATE2-K proteins in the secretion of vancomycin has yet to be demonstrated (Pais et al., 2020). In our patient, vancomycin and creatinine clearances were simultaneously increased, suggesting that these transporters are also involved; otherwise, intracellular accumulation would have led to toxicity.
In an in vitro study, Shibayama et al. (2007) showed that hOCT1 and hOCT2 transport meropenem but were not able to examine transport across the apical membrane into the lumen. Uchino et al. (2000) reported that Npt1, an inorganic phosphate transporter, participates in the renal secretion of penem antibiotics. While it is known that renal excretion of meropenem is largely through active transport, the mechanism behind the rapid elimination of meropenem in our patient with ARC remains unclear.
On the Causes of and Factors Contributing to Augmented Renal Clearance
There is no consensus in the literature regarding the causes of ARC. Recently, Cook and Hatton–Kolpek (2019) addressed the risk factors and potential contributing factors to the emergence of ARC. Chen and Nicolau (2020) published a review on ARC and put forward suggestions on how to identify ARC. There is further no consensus regarding how to define ARC. It should be kept in mind that low–molecular weight endogenous compounds (like creatinine) and drugs (such as vancomycin and meropenem) can be eliminated both by glomerular filtration and tubular secretion. Nevertheless, there is currently a broad consensus in considering the measured creatinine clearance of 130 ml/min/1.73 m2 as the lower limit for the diagnosis of ARC. We therefore fully adopt the definition of ARC as creatinine clearance slightly above the upper normal reference limit for GFR, that is, >130 ml/min/1.73 m2, as suggested by the Australian team and further advocated by them (Silva et al., 2020) and others (Mahmoud and Shen, 2017; Bilbao-Meseguer et al., 2018; Chen and Nicolau, 2020). Thus, creatinine clearance above this limit indicates ARC, caused by either increased glomerular filtration or tubular secretion. Table 3 shows the clearance rates of our patient both in absolute (ml/min) and normalized (mL/min/1.73 m2) terms. The absolute clearance rates however may just signify high clearance rates due to the big size of the patient and does not by itself indicate ARC. It is therefore confusing that some authors equalize high clearance rates in absolute terms with ARC. Neither can demand for high dosage of drugs by itself define ARC. However, the absolute clearance of each patient is the basis for dosage of the drugs.
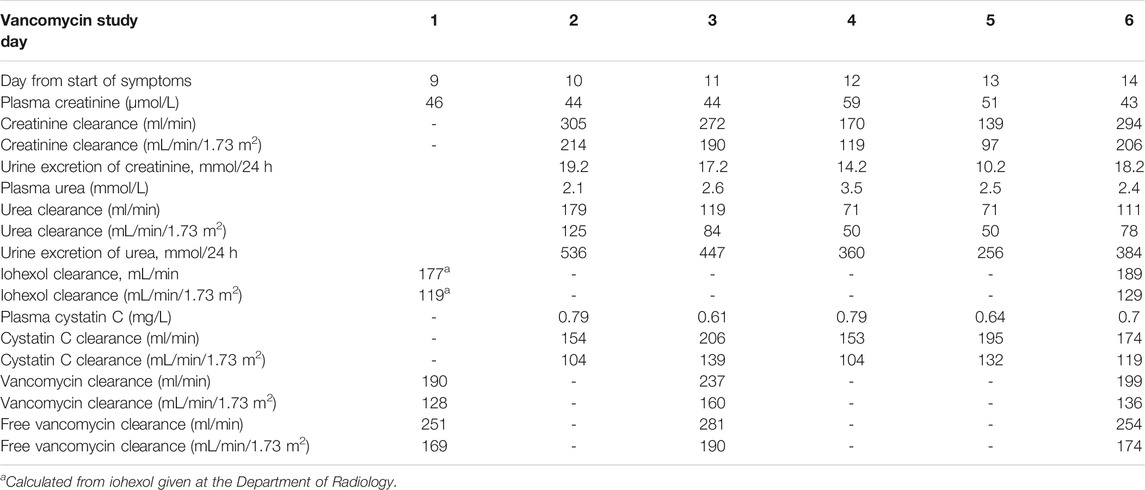
TABLE 3. Creatinine, urea, iohexol, cystatin C, and vancomycin (total and free) clearances together with plasma concentrations of creatinine, urea, and cystatin C and excretion of creatinine and urea during the 6 days of clearance studies.
One suggested and cherished cause of ARC is an increased cardiac output that would give increased renal blood flow, and thus increased GFR (Sime et al., 2015; Atkinson, 2018). In our patient, an echocardiogram was performed that showed no signs of endocarditis. At this investigation, the cardiac output was considered normal with normal contractility, and there were no signs of organic heart failure. We have measured GFR with the best available method (iohexol plasma clearance), and thus, we exclude the increased cardiac output with increased GFR as the cause of ARC in our patient. The GFR measured by iohexol clearance was quite normal, and the creatinine clearance after trimethoprim treatment decreased to half its value into the area of normal GFR. A similar result was found with urea clearance. We therefore consider the cause of ARC in our patient to be increased tubular secretion. The next question however is whether this is constitutional in our patient or induced during the current period of illness. We reviewed the earlier medical records of our patient and found seven serum creatinine values with a mean ± SD of 68.1 ± 13.0 µmol/L during the years 2012–2019. The mean serum creatinine of 44.2 ± 5.7 µmol/L during the present hospital stay was significantly lower than that during the earlier period (p < 0.001). Furthermore, after a follow-up of 16 months, his plasma creatinine was 69 µmol/L, creatinine clearance was 110 ml/min/1.73 m2, urea clearance was 48 ml/min/1.73 m2, and iohexol clearance was 88 ml/min/1.73 m2, that is, quite normal results. It therefore seems that during the stay at neurosurgical unit, he had a period of induced increased tubular secretion of creatinine as the dominant cause of ARC. The conclusion of increased tubular secretions in our patient however does not preclude that increased GFR occurs in other patients. In a recent publication, it was shown that 29 patients had ARC as measured by 6-hour creatinine clearance. Of these, 16 patients had hyperfiltration and 13 had not, as measured by iohexol clearance (Collet et al., 2021). Thus, ARC can be due to increased GFR or increased tubular secretion or both. Our study indicates that this may also be true for drugs such as vancomycin and meropenem. To discriminate between these possibilities, employment of a highly standard GFR method is necessary, as well as actual measurement of creatinine or drug clearances that can indicate augmented clearance beyond the patients true GFR.
Conclusion
This case report describes a 34-year-old man with multiple brain abscesses where the clearances of creatinine, urea, and vancomycin were all increased. High demand for meropenem to reach optimal plasma concentration also indicated increased elimination rate. The increased elimination of these compounds was not due to increased GFR as shown by concomitant measured normal iohexol and cystatin C clearances. We therefore infer that the increased clearances were due to increased tubular secretion. For creatinine, this is proven by the fact that during the study period, temporarily given trimethoprim lowered the clearance of creatinine to normal. High clearance of urea that fell into normal was also found after trimethoprim treatment. However, tubular transport and secretion of urea into the urine are quite different from those of creatinine, and there is no information available in the literature on trimethoprim effects on tubular transporters of urea. This is a new finding and needs to be corroborated in larger studies. Anyhow, urea clearance was higher than normal, and this by itself infers increased excretion into the urine by the tubular system. To our knowledge, there is no reliable information available on human tubular transporters of vancomycin. We have no data on vancomycin clearance the immediate days after trimethoprim dosing, so we cannot evaluate the possible effect of trimethoprim on tubular transport of this drug. However, vancomycin clearance as calculated from non–protein-bound vancomycin was high, above normal GFR and 130 ml/min/1.73 m2, set for definition of ARC, and close to the level of creatinine clearance. Thus, our study shows increased tubular secretion of vancomycin, but we cannot indicate the mechanism for its tubular secretion. A limitation of our study is that the clearance rates of meropenem were not actually measured.
We conclude that tubular secretion is responsible for the clearance rates beyond the true normal GFR. Further studies on tubular secretion are needed to understand the mechanisms for increased tubular secretion of drugs in intensive care patients with ARC. Increased clearances of drugs like vancomycin and β-lactam antibiotics may result in life-threatening subtherapeutic antibiotic concentrations. Finally, dose recommendations for renally excreted drugs in ARC patients should be implemented to prevent treatment failure, increased morbidity, and mortality.
Data Availability Statement
The original contributions presented in the study are included in the article/supplementary material; further inquiries can be directed to the corresponding author.
Ethics Statement
The studies involving human participants were reviewed and approved by Regionala etikprövningsnämnden i Linköping c/o Medical Faculty Linköpings universitet 58185 Linköping. The patients/participants provided their written informed consent to participate in this study. Written informed consent was obtained from the individual(s) for the publication of any potentially identifiable images or data included in this article.
Author Contributions
BK, DND, HH, and AH provided study concept, design, and project execution. MF and HH included the patient in the study and MF, AH, ÅÖB, DND, MH (5th author), and MH (6th author) performed data acquisition and processing. BK drafted the manuscript and included the intellectual content and the revisions from MF, AH, ÅÖB, and PM to the final manuscript. All authors revised the manuscript critically for important intellectual content and approved the final version for submission.
Funding
Swedish government funding to university hospitals for research, ALF (LIO-887221), and VINNOVA, collaboration platform for innovation of existing antibiotics (PLATINEA, 2018-03340).
Conflict of Interest
The authors declare that the research was conducted in the absence of any commercial or financial relationships that could be construed as a potential conflict of interest.
Publisher’s Note
All claims expressed in this article are solely those of the authors and do not necessarily represent those of their affiliated organizations, or those of the publisher, the editors and the reviewers. Any product that may be evaluated in this article, or claim that may be made by its manufacturer, is not guaranteed or endorsed by the publisher.
Acknowledgments
The contribution with the pharmacokinetic calculations by Olof Breuer, MD, is greatly appreciated.
Abbreviations
ARC, augmented renal clearance; BSA, body surface area; CNS, central nervous system; CRP, C-reactive protein; CSF, cerebrospinal fluid; OCT, organic cationic transporter; hOCT1, human organic cation transporter-1; hOCT2, human organic cation transporter-2; MATE1, multidrug and toxin extrusion 1; MATE2-K, multidrug and toxin extrusion 2-K; MRI, magnetic resonance imaging; HIV, human immunodeficiency virus; TMP-SMX, trimethoprim–sulfamethoxazole; UT-A1, urea transporter A1; UT-A3, urea transporter A3.
References
Albanèse, J., Léone, M., Bruguerolle, B., Ayem, M. L., Lacarelle, B., and Martin, C. (2000). Cerebrospinal Fluid Penetration and Pharmacokinetics of Vancomycin Administered by Continuous Infusion to Mechanically Ventilated Patients in an Intensive Care Unit. Antimicrob. Agents Chemother. 44 (5), 1356–1358. doi:10.1128/aac.44.5.1356-1358.2000
Atkinson, A. J. (2018). Augmented Renal Clearance. Transl Clin. Pharmacol. 26 (3), 111–114. doi:10.12793/tcp.2018.26.3.111
Bäck, S. E., Ljungberg, B., Nilsson-Ehle, I., Borgå, O., and Nilsson-Ehle, P. (1989). Age Dependence of Renal Function: Clearance of Iohexol and P-Amino Hippurate in Healthy Males. Scand. J. Clin. Lab. Invest. 49 (7), 641–646. doi:10.1080/00365518909091539
Berglund, F., Killander, J., and Pompeius, R. (1975). Effect of Trimethoprim-Sulfamethoxazole on the Renal Excretion of Creatinine in Man. J. Urol. 114 (6), 802–808. doi:10.1016/s0022-5347(17)67149-0
Bilbao-Meseguer, I., Rodríguez-Gascón, A., Barrasa, H., Isla, A., and Solinís, M. Á. (2018). Augmented Renal Clearance in Critically Ill Patients: A Systematic Review. Clin. Pharmacokinet. 57 (9), 1107–1121. doi:10.1007/s40262-018-0636-7
Bröchner-Mortensen, J. (1972). A Simple Method for the Determination of Glomerular Filtration Rate. Scand. J. Clin. Lab. Invest. 30 (3), 271–274. doi:10.3109/00365517209084290
Brouwer, M. C., Coutinho, J. M., and van de Beek, D. (2014). Clinical Characteristics and Outcome of Brain Abscess: Systematic Review and Meta-Analysis. Neurology 82 (9), 806–813. doi:10.1212/WNL.0000000000000172
Brouwer, M. C., Tunkel, A. R., McKhann, G. M., and van de Beek, D. (2014). Brain Abscess. N. Engl. J. Med. 371 (5), 447–456. doi:10.1056/NEJMra1301635
Chen, I. H., and Nicolau, D. P. (2020). Augmented Renal Clearance and How to Augment Antibiotic Dosing. Antibiotics (Basel) 9 (7), 393. doi:10.3390/antibiotics9070393
Chheda, L. V., Sobol, W. M., Buerk, B. M., and Kurz, P. A. (2011). Endogenous Endophthalmitis with Brain Abscesses Caused by Streptococcus Constellatus. Arch. Ophthalmol. 129 (4), 517–518. doi:10.1001/archophthalmol.2011.59
Collet, M., Hijazi, D., Sevrain, P., Barthelemy, R., Labeyrie, M. A., Prie, D., et al. (2021). Evaluation of Glomerular Filtration Rate Using Iohexol Plasma Clearance in Critically Ill Patients with Augmented Renal Creatinine Clearance: A Single-Centre Retrospective Study. Eur. J. Anaesthesiol 38 (6), 652–658. doi:10.1097/eja.0000000000001501
Cook, A. M., and Hatton-Kolpek, J. (2019). Augmented Renal Clearance. Pharmacotherapy 39 (3), 346–354. doi:10.1002/phar.2231
Darlow, C. A., McGlashan, N., Kerr, R., Oakley, S., Pretorius, P., Jones, N., et al. (2020). Microbial Aetiology of Brain Abscess in a UK Cohort: Prominent Role of Streptococcus Intermedius. J. Infect. 80 (6), 623–629. doi:10.1016/j.jinf.2020.03.011
Dubois, D., and Dubois, E. F. (1989). Nutrition Metabolism Classic - a Formula to Estimate the Approximate Surface-Area if Height and Weight Be Known. 1916. Nutrition 5 (5), 303–311.
Erlandsen, E. J., and Randers, E. (2018). Reference Intervals for Plasma Cystatin C and Plasma Creatinine in Adults Using Methods Traceable to International Calibrators and Reference Methods. J. Clin. Lab. Anal. 32, e22433. doi:10.1002/jcla.22433
Fanos, V., and Cataldi, L. (2001). Renal Transport of Antibiotics and Nephrotoxicity: a Review. J. Chemother. 13 (5), 461–472. doi:10.1179/joc.2001.13.5.461
Grubb, A., Horio, M., Hansson, L. O., Björk, J., Nyman, U., Flodin, M., et al. (2014). Generation of a New Cystatin C-Based Estimating Equation for Glomerular Filtration Rate by Use of 7 Assays Standardized to the International Calibrator. Clin. Chem. 60 (7), 974–986. doi:10.1373/clinchem.2013.220707
Junge, W., Wilke, B., Halabi, A., and Klein, G. (2004). Determination of Reference Intervals for Serum Creatinine, Creatinine Excretion and Creatinine Clearance with an Enzymatic and a Modified Jaffé Method. Clin. Chim. Acta 344 (1-2), 137–148. doi:10.1016/j.cccn.2004.02.007
Klein, J. D., Blount, M. A., and Sands, J. M. (2011). Urea Transport in the Kidney. Compr. Physiol. 1 (2), 699–729. doi:10.1002/cphy.c100030
Koch, C. D., Arnst, E., and Rommel, K. (1980). Urea and Creatinine Levels and Clearances: Observations in 25 Healthy Subjects for One Year (Author's Transl). J. Clin. Chem. Clin. Biochem. 18 (7), 423–429. doi:10.1515/cclm.1980.18.7.423
Krutzén, E., Bäck, S. E., Nilsson-Ehle, I., and Nilsson-Ehle, P. (1984). Plasma Clearance of a New Contrast Agent, Iohexol: a Method for the Assessment of Glomerular Filtration Rate. J. Lab. Clin. Med. 104 (6), 955–961.
Kyhse-Andersen, J., Schmidt, C., Nordin, G., Andersson, B., Nilsson-Ehle, P., Lindström, V., et al. (1994). Serum Cystatin C, Determined by a Rapid, Automated Particle-Enhanced Turbidimetric Method, Is a Better Marker Than Serum Creatinine for Glomerular Filtration Rate. Clin. Chem. 40 (10), 1921–1926. doi:10.1093/clinchem/40.10.1921
Lee, S. C., and Lueck, C. J. (2014). Cerebrospinal Fluid Pressure in Adults. J. Neuroophthalmol 34 (3), 278–283. doi:10.1097/WNO.0000000000000155
Lin Wu, F. L., Liu, S. S., Yang, T. Y., Win, M. F., Lin, S. W., Huang, C. F., et al. (2015). A Larger Dose of Vancomycin Is Required in Adult Neurosurgical Intensive Care Unit Patients Due to Augmented Clearance. Ther. Drug Monit. 37 (5), 609–618. doi:10.1097/FTD.0000000000000187
Lindgren, C., Koskinen, L. O., Ssozi, R., and Naredi, S. (2019). Cerebrospinal Fluid Lactate and Neurological Outcome after Subarachnoid Haemorrhage. J. Clin. Neurosci. 60, 63–67. doi:10.1016/j.jocn.2018.10.025
Lonsdale, D. O., Udy, A. A., Roberts, J. A., and Lipman, J. (2013). Antibacterial Therapeutic Drug Monitoring in Cerebrospinal Fluid: Difficulty in Achieving Adequate Drug Concentrations. J. Neurosurg. 118 (2), 297–301. doi:10.3171/2012.10.JNS12883
Mahmoud, S. H., and Shen, C. (2017). Augmented Renal Clearance in Critical Illness: An Important Consideration in Drug Dosing. Pharmaceutics 9 (3), 36. doi:10.3390/pharmaceutics9030036
Mali, J. O., Falk, N. S., Mali, Y. P., and Mencias, L. (2015). Endogenous Endophthalmitis with Iris Abscess after Routine Dental Cleaning. JAMA Ophthalmol. 133 (5), 616–617. doi:10.1001/jamaophthalmol.2015.12
Marks, L., Duchein, S., González, , Suárez, H. A., and Rivolta, S. (2019). Validation of a Immunoassay for the Determination of Vancomycin in Cerebospinal Fluid. Rev. Fac. Cien Med. Univ. Nac Cordoba 76 (2), 101–106. doi:10.31053/1853.0605.v76.n2.23550
Motohashi, H., and Inui, K. (2013). Organic Cation Transporter OCTs (SLC22) and MATEs (SLC47) in the Human Kidney. Aaps J. 15 (2), 581–588. doi:10.1208/s12248-013-9465-7
Pais, G. M., Liu, J., Zepcan, S., Avedissian, S. N., Rhodes, N. J., Downes, K. J., et al. (2020). Vancomycin-Induced Kidney Injury: Animal Models of Toxicodynamics, Mechanisms of Injury, Human Translation, and Potential Strategies for Prevention. Pharmacotherapy 40 (5), 438–454. doi:10.1002/phar.2388
Rodvold, K. A., Blum, R. A., Fischer, J. H., Zokufa, H. Z., Rotschafer, J. C., Crossley, K. B., et al. (1988). Vancomycin Pharmacokinetics in Patients with Various Degrees of Renal Function. Antimicrob. Agents Chemother. 32 (6), 848–852. doi:10.1128/aac.32.6.848
Rybak, M., Lomaestro, B., Rotschafer, J. C., Moellering, R., Craig, W., Billeter, M., et al. (2009). Therapeutic Monitoring of Vancomycin in Adult Patients: a Consensus Review of the American Society of Health-System Pharmacists, the Infectious Diseases Society of America, and the Society of Infectious Diseases Pharmacists. Am. J. Health Syst. Pharm. 66 (1), 82–98. doi:10.2146/ajhp080434
Rybak, M. J., Le, J., Lodise, T. P., Levine, D. P., Bradley, J. S., Liu, C., et al. (2020). Therapeutic Monitoring of Vancomycin for Serious Methicillin-Resistant Staphylococcus aureus Infections: A Revised Consensus Guideline and Review by the American Society of Health-System Pharmacists, the Infectious Diseases Society of America, the Pediatric Infectious Diseases Society, and the Society of Infectious Diseases Pharmacists. Am. J. Health Syst. Pharm. 77 (11), 835–864. doi:10.1093/ajhp/zxaa036
Scharf, C., Paal, M., Schroeder, I., Vogeser, M., Draenert, R., Irlbeck, M., et al. (2020). Therapeutic Drug Monitoring of Meropenem and Piperacillin in Critical Illness-Experience and Recommendations from One Year in Routine Clinical Practice. Antibiotics (Basel) 9 (3), 131. doi:10.3390/antibiotics9030131
Shibayama, T., Sugiyama, D., Kamiyama, E., Tokui, T., Hirota, T., and Ikeda, T. (2007). Characterization of CS-023 (RO4908463), a Novel Parenteral Carbapenem Antibiotic, and Meropenem as Substrates of Human Renal Transporters. Drug Metab. Pharmacokinet. 22 (1), 41–47. doi:10.2133/dmpk.22.41
Shokouhi, S., and Alavi Darazam, I. (2014). Determination of Vancomycin Trough Level in Serum and Cerebrospinal Fluid of Patients with Acute Community-Acquired Meningitis: A Prospective Study. J. Infect. 69 (5), 424–429. doi:10.1016/j.jinf.2014.06.010
Silva, C. M., Udy, A. A., and Baptista, J. P. (2020). Urinary Creatinine Clearance and Pharmacokinetics Studies: If We Can Measure it, Why Do We Estimate it?. Antimicrob. Agents Chemother. 64 (9), e00980–20. doi:10.1128/AAC.00980-20
Sime, F. B., Udy, A. A., and Roberts, J. A. (2015). Augmented Renal Clearance in Critically Ill Patients: Etiology, Definition and Implications for Beta-Lactam Dose Optimization. Curr. Opin. Pharmacol. 24, 1–6. doi:10.1016/j.coph.2015.06.002
Sokol, P. P. (1991). Mechanism of Vancomycin Transport in the Kidney: Studies in Rabbit Renal brush Border and Basolateral Membrane Vesicles. J. Pharmacol. Exp. Ther. 259 (3), 1283–1287.
Sotgiu, G., Saderi, L., Petruccioli, E., Aliberti, S., Piana, A., Petrone, L., et al. (2019). QuantiFERON TB Gold Plus for the Diagnosis of Tuberculosis: a Systematic Review and Meta-Analysis. J. Infect. 79 (5), 444–453. doi:10.1016/j.jinf.2019.08.018
Teasdale, G., and Jennett, B. (1974). Assessment of Coma and Impaired Consciousness. A Practical Scale. Lancet 2 (7872), 81–84. doi:10.1016/s0140-6736(74)91639-0
Teasdale, G., and Jennett, B. (1976). Assessment and Prognosis of Coma after Head Injury. Acta Neurochir (Wien) 34 (1-4), 45–55. doi:10.1007/BF01405862
Uchino, H., Tamai, I., Yabuuchi, H., China, K., Miyamoto, K., Takeda, E., et al. (2000). Faropenem Transport across the Renal Epithelial Luminal Membrane via Inorganic Phosphate Transporter Npt1. Antimicrob. Agents Chemother. 44 (3), 574–577. doi:10.1128/aac.44.3.574-577.2000
Urakami, Y., Kimura, N., Okuda, M., and Inui, K. (2004). Creatinine Transport by Basolateral Organic Cation Transporter hOCT2 in the Human Kidney. Pharm. Res. 21 (6), 976–981. doi:10.1023/b:pham.0000029286.45788.ad
Wang, K., and Kestenbaum, B. (2018). Proximal Tubular Secretory Clearance: A Neglected Partner of Kidney Function. Clin. J. Am. Soc. Nephrol. 13 (8), 1291–1296. doi:10.2215/CJN.12001017
Keywords: suboptimal antibiotic treatment, tubular cell membrane transporters, glomerular filtration rate, augmented renal clearance, vancomycin clearance, iohexol clearance, creatinine clearance, urea clearance
Citation: Fransson M, Helldén A, Östholm Balkhed Å, Nezirević Dernroth D, Ha M, Haglund M, Milos P, Hanberger H and Kågedal B (2021) Case Report: Subtherapeutic Vancomycin and Meropenem Concentrations due to Augmented Renal Clearance in a Patient With Intracranial Infection Caused by Streptococcus intermedius. Front. Pharmacol. 12:728075. doi: 10.3389/fphar.2021.728075
Received: 20 June 2021; Accepted: 01 September 2021;
Published: 06 October 2021.
Edited by:
Jeffrey Lipman, The University of Queensland, AustraliaReviewed by:
Tony Whitehouse, Queen Elizabeth Hospital Birmingham, United KingdomAndrew Udy, The Alfred Hospital, Australia
Copyright © 2021 Fransson, Helldén, Östholm Balkhed, Nezirević Dernroth, Ha, Haglund, Milos, Hanberger and Kågedal. This is an open-access article distributed under the terms of the Creative Commons Attribution License (CC BY). The use, distribution or reproduction in other forums is permitted, provided the original author(s) and the copyright owner(s) are credited and that the original publication in this journal is cited, in accordance with accepted academic practice. No use, distribution or reproduction is permitted which does not comply with these terms.
*Correspondence: Bertil Kågedal, bertil.kagedal@liu.se
†These authors have contributed equally to this work and share first authorship