Alterations in the vaginal microbiota of patients with preterm premature rupture of membranes
- 1Department of Obstetrics, The Second Hospital of Shandong University, Jinan, China
- 2Center for Reproductive Medicine, Department of Obstetrics and Gynecology, Qilu Hospital, Cheeloo College of Medicine, Shandong University, Jinan, China
Background: Preterm premature rupture of membranes (PPROM) is a common pregnancy complication. Yet, the main cause of PPROM remains poorly understood. In this study, we used 16S rRNA gene sequencing technology to identify the differences in vaginal microbiota between pregnant women with PPROM and those who delivered at term.
Methods: Vaginal samples were collected from 48 patients with PPROM and 54 age- and gestational age-matched pregnant women who delivered at term (controls). The vaginal microbiota of the two groups was compared using 16S rRNA gene sequencing of the V3-V4 regions.
Results: The vaginal microbial composition of the PPROM group was significantly different from that of the control group. Our results showed that the diversity of vaginal microbiota in patients with PPROM increased compared with controls. The relative abundance of Lactobacillus iners, Gardnerella vaginalis, Prevotella bivia, Ochrobactrum sp., Prevotella timonensis, and Ureaplasma parvum were more abundant in patients with PPROM, while Lactobacillus crispatus and Lactobacillus gasseri were more abundant in controls. Ochrobactrum sp., Prevotella timonensis, and Gardnerella vaginalis, could serve as biomarkers for PPROM. Finally, we proposed several metabolic pathways, including PWY-6339, PWY-6992, and PWY-7295.
Conclusion: PPROM is characterized by vaginal microbial dysbiosis. The dysbiotic vaginal microbiota signatures in patients with PPROM include a higher bacterial diversity, decreased autochthonous bacteria, and increased pathogenic bacteria. These results may be beneficial for developing biomarkers for screening and early diagnosis of PPROM and may provide effective preventative treatments.
Introduction
The premature rupture of membranes (PROM) is defined as membrane rupture prior to the onset of labor. PROM that occurs before 37 weeks of gestation is defined as preterm premature rupture of membranes (PPROM). PPROM increases neonatal and maternal disease risks, which is among the main causes of premature birth. PPROM leads to approximately 18%–20% of perinatal deaths in the United States. An increase in perinatal mortality and neonatal morbidity by 4-fold and 3-fold, respectively, has been observed in patients with PPROM. Common neonatal complications of PPROM include respiratory distress syndrome (RDS), intrauterine infection, and intraventricular hemorrhage (IVH). RDS occurs in 10%–40% of PPROM patients and leads to 40%–70% of neonatal deaths, while intrauterine infection occurs in 15%–30% of women with PPROM and causes 3%–20% of neonatal deaths (Dale et al., 1989; Yoon et al., 2000). Other neonatal complications include fetal pulmonary hypoplasia, skeletal deformities, cord prolapse, and neuro-developmental impairment (Yoon et al., 2000; Powell et al., 2021). In addition to neonatal complications, PPROM may also lead to complications in pregnant women. Intrauterine infection with clinical evidence has been observed in 15%–35% of women with PPROM, while postpartum infection occurs in approximately 15%–25% of PPROM cases (Garite and Freeman, 1982; Beydoun and Yasin, 1986; Kenyon et al., 2013). Moreover, placentae abruptio occurs in 2%–5% of pregnancies with PPROM (Ananth et al., 2004). These maternal and infant complications pose a serious burden on society and families.
Various pathological mechanisms, acting either alone or in combination with other factors, can lead to PPROM. For example, vaginal microbiota has an important role in the vagina (Turnbaugh et al., 2007) and can affect the health of the female genital tract (O'Hanlon et al., 2010). The abnormal vaginal microbiota is an important risk factor for PPROM (Coleman et al., 2013; Brown et al., 2018). Hypothetically, the colonization of pathogenic bacteria in the local and upper cervix and fetal membrane (Parry and Strauss, 1998) can lead to an inflammatory cascade reaction (Fortunato et al., 2002; Helmig et al., 2002; Shobokshi and Shaarawy, 2002), and in turn, the remodeling and destruction of membrane structure and premature rupture (Fortner et al., 2014). Previous studies on vaginal microorganisms have obtained data through microscopic examinations and traditional culture methods. However, owing to the large abundance and diversity of bacterial species in the vagina, not all bacteria may be identified through traditional culture, isolation, and identification methods (Reinhold-Hurek et al., 2015). Thus, information regarding vaginal microbiota is limited.
In recent years, the development of 16S rRNA gene sequencing technology has provided a new method for studying microbiota, but few studies have investigated the composition of vaginal microbiota in patients with PPROM (Baldwin et al., 2015; Kacerovsky et al., 2015; Paramel Jayaprakash et al., 2016). Studies on vaginal microbiota showed ethnic specificity between vaginal microbiota and preterm birth (Callahan et al., 2017). The integrated human microbiome program (iHMP) showed that the vaginal microbiota was a risk factor of preterm birth in African American women (Fettweis et al., 2019). Moreover, pregnant women of European descent with high levels of Lactobacillus crispatus showed a relatively low risk of preterm birth. Whilst the composition of vaginal microbiota differs between women of African and European descent, its impact on the risk of preterm birth remains controversial.
The relationship between the characteristics of vaginal microbiota in pregnant women in China and PPROM is still not well understood. Further research into the occurrence, prediction, and management of the pregnancy complications is required. In this study, we used 16S rRNA gene sequencing technology to compare the characteristics of vaginal microbiota between patients with PPROM and age- and gestational age-matched pregnant women who delivered at term to further investigate the relationship between vaginal microbiota and PPROM.
Methods
Study design and sample collection
The study was approved by the Second Hospital of Shandong University Research Ethics Board (KYLL-2018 (LW)043). All subjects provided written informed consent prior to sample collection.
This cross-sectional study aimed to compare the characteristics of vaginal microbiota between patients with PPROM and age- and gestational age-matched pregnant women who delivered at term (controls). All patients were enrolled at the Second Hospital of Shandong University between January 2019 and April 2020. The diagnosis of PROM included: (1) visual pooling of clear fluid in the posterior fornix of the vagina or leakage of fluid from the cervical os; (2) an alkaline pH of the cervicovaginal discharge (nitrazine test, pH ≥6.5), turning yellow nitrazin paper to blue; (3) microscopic fern-like crystals of the cervicovaginal discharge upon drying. The inclusion criteria for the PPROM group were: age of the pregnant women >18 years old, singleton gestation, gestational age between 24–36+6 weeks, and confirmed PROM based on clinical criteria ≤12 h. The exclusion criteria for the PPROM group were: the application of antibiotics, probiotics, and immunosuppressants in recent 2 months; fetal developmental abnormalities; complications, such as heart disease, immune diseases, cervical surgery, and so on. The inclusion criteria for the term delivery group included: age of pregnant women >18 years old, singleton gestation, normal routine examination of leucorrhea, and gestational age between 24–36+6 weeks. The exclusion criteria were: history of preterm labour or PPROM; with PPROM; application of antibiotics, probiotics, and immunosuppressants in recent 2 months; fetal developmental abnormalities; complications, such as heart disease, immune diseases, cervical surgery, and so on.
Three swabs were collected from the women with PPROM at enrolment: a cervical swab for microbial cultivation and two vaginal swabs from the anterior vaginal fornix, lateral and anterior vaginal wall (one for Nugent scoring and one for vaginal microbiota analysis). All swabs for the microbiota analysis were stored at -80°C within 2 h of sampling, and all swabs for cultivation and Nugent scoring were presented to the central laboratory of the Second Hospital of Shandong University. Vaginal secretions were cultured with a blood plate.
Demographic and clinical characteristics were collected from medical records and participants.
DNA extraction and 16S rRNA gene sequencing
Bacterial genomic DNA from vaginal swabs was extracted using a modified cetyltrimethylammonium bromide (CTAB) protocol. The V3-V4 region of the 16S rRNA genes was amplified by PCR and sequencing was performed using the Illumina NovaSeq PE250 platform with NovaSeq 6000 SP Reagent Kit (500 cycles) at Shanghai Personal Biotechnology Co., Ltd (Shanghai, China).
Bioinformatic analysis of 16S rRNA sequencing
The 16S rRNA sequencing data analysis was performed using Quantitative Insights Into Microbial Ecology (QIIME2 V. 2019.4) (Bolyen and Rideout, 2019) and R packages (v3.2.0). DADA2 software, wrapped in QIIME2, was used to quality filter, denoise, merge, and remove chimera (Callahan et al., 2016). Non-singleton amplicon sequence variants (ASVs) were aligned with Multiple Alignment using Fast Fourier Transformation (Katoh et al., 2002) and used to construct a phylogeny with FastTree2 (Price et al., 2009). The α-diversity metrics and β-diversity metrics were estimated using the diversity plugin with samples rarefied to 60308 sequences per sample (Lozupone and Knight, 2005; Lozupone et al., 2007). Taxonomy was assigned to ASVs using the classify-sklearn naïve Bayes taxonomy classifier in a feature-classifier plugin (Bokulich et al., 2018) against the Greengenes Database (DeSantis et al., 2006) (Release 13.8, http://greengenes.secondgenome.com/). Lactobacillus species were further annotated and validated by manual BLAST search in the National Biotechnology Information Center (NCBI) database.
Statistical analysis
The α-diversity indices were calculated using the ASV table in QIIME2, visualised by R script as box plots to intuitively show the differences in diversity and richness between the PPROM group and term delivery controls. Statistical significance was verified using the Kruskal-Wallis rank-sum test and Dunn’s test post-hoc. A β-diversity analysis was performed to investigate the structural variation of microbial communities between the two groups using Jaccard metrics and was visualized using nonmetric multidimensional scaling (NMDS) and principal coordinate analysis (PCoA). Analysis of similarities (ANOSIM) using QIIME2 was used to assess whether the differences in microbiota structure between the two groups was significant. We used linear discriminant analysis effect size (LEfSe) analysis to identify taxa or pathways that were differentially abundant between patients with PPROM and term delivery controls (Segata et al., 2011). This method uses the non-parametric factorial Kruskal-Wallis rank-sum test to detect elements with significant differential abundances and linear discriminant analysis (LDA) to calculate the effect size of each. The operating characteristic curves (receiving operational curve, ROC) were constructed, and the area under the curve (AUC) was calculated to assess the diagnostic performance of the model using a random forests algorithm for analysis based on the species that were significantly different according to LEfSe analysis results. The correlation network was constructed using the SparCC algorithm python, and iGraph packages. The differences in maternal age and gestational age between the PPROM and term delivery groups were tested by an independent sample t-test using SPSS software. Other statistical analyses were performed, such as Fisher’s exact test. A P-value < 0.05 was considered to be statistically significant.
Results
Clinical characteristics of the study participants
Data were derived from vaginal microbiota of a large and well-characterized cohort which comprised 48 patients with PPROM and 54 age- and gestational age-matched pregnant women who delivered at term (controls). Table 1 shows the detailed demographic and clinical features of all women involved in the study. Bacterial vaginosis is a mixed infection caused by vaginal dysbacteriosis, mainly characterized by the increase of thin vaginal secretions with a fishy smell. Abnormal vaginal secretion culture refers to the growth of aerobic bacteria on the culture plates.
Species accumulation curve
The species accumulation curve tended to be flat, indicating that the total number of ASV in the community would not significantly increase with new samples and that the sample size was sufficient (Supplementary Figure S1).
Bacteria differentially abundant in PPROM versus controls
At the genus level, the most abundant vaginal bacteria in term delivery controls were Lactobacillus (relative abundance: 91.8% ± 23.7%; data shown as mean ± SD), followed by Gardnerella (relative abundance: 3.2% ± 13.1%; data shown as mean ± SD) and Atopobium (relative abundance: 2.9% ± 12.5%; data shown as mean ± SD). Most samples in the control group were dominated by Lactobacillus (90.74%), and few samples were dominated by Gardnerella (3.70%) and Prevotella (1.85%). The dominant taxa was defined as having a relative abundance of more than 50% (Supplementary Figures S2A, B). In the PPROM samples, the most abundant genus was Lactobacillus (relative abundance: 71.5% ± 32.6%; data shown as mean ± SD), followed by Gardnerella (relative abundance: 6.9% ± 7.1%; data shown as mean ± SD) and Prevotella (relative abundance: 4.4% ± 10.7%; data shown as mean ± SD). The majority of the PPROM group was dominated by Lactobacillus (77.1%) (Supplementary Figures S2C, D).
Compared to controls, the proportion of Lactobacillus as the dominant taxa decreased significantly, and the proportion of non-single genera as the dominant genus increased significantly (P<0.01, Fisher exact test).
The most abundant species found in the term delivery controls was Lactobacillus crispatus (relative abundance: 59.6% ± 46.8%; data shown as mean ± SD), followed by Lactobacillus iners (relative abundance: 24.2% ± 38.9%; data shown as mean ± SD), and Lactobacillus gasseri (relative abundance: 3.5% ± 13.9%; data shown as mean ± SD) (Figure 1A). Lactobacillus crispatus was found to be the dominant species in 63% of the term delivery control samples. Furthermore, Lactobacillus iners was dominant in 12 samples (22.2%), and Gardnerella vaginalis was the dominant species in two samples (3.7%) (Figure 1B).
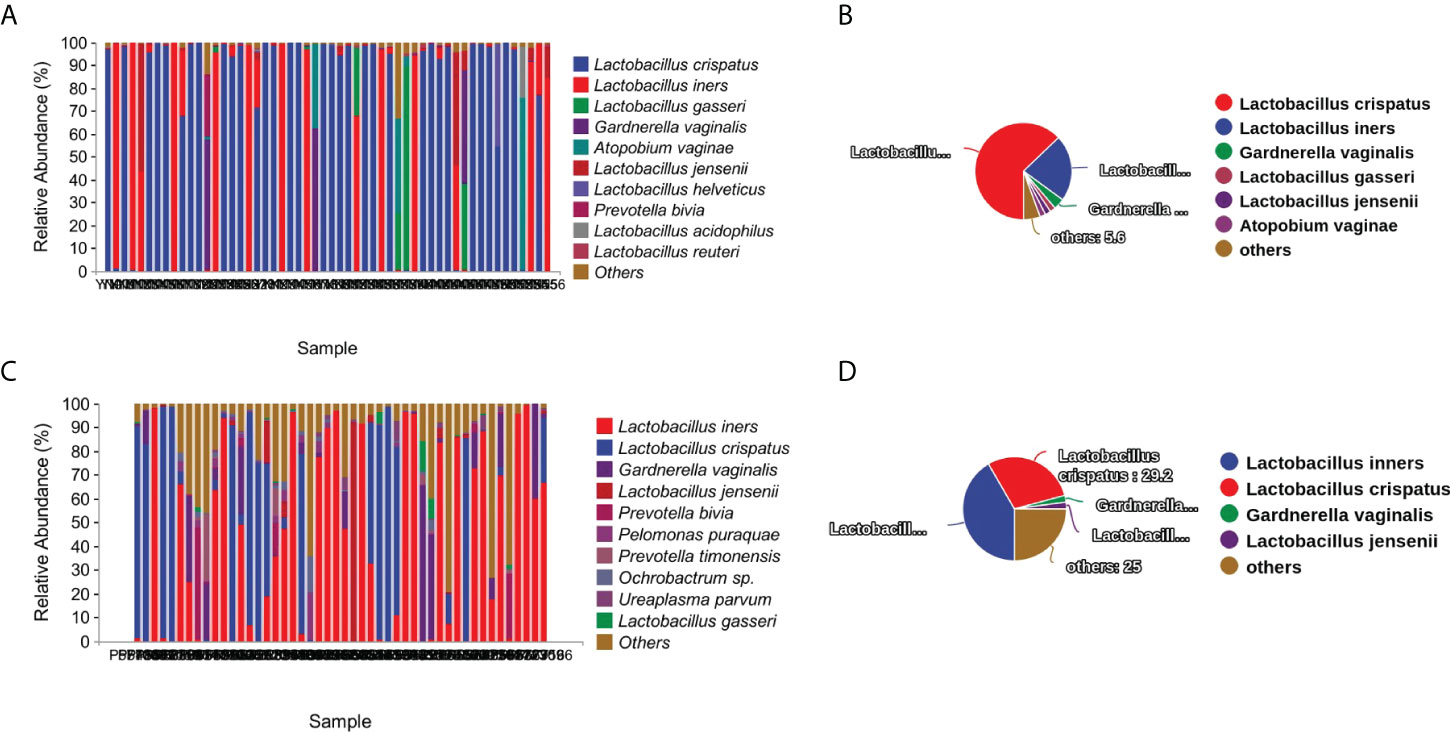
Figure 1 Vaginal microbiota composition and percentage of dominant taxa at species level. (A) Vaginal microbiota composition in the term delivery group at the species level. (B) Percentage of dominant species in the term delivery group. (C) Vaginal microbiota composition in the PPROM group at the species level. (D) Percentage of dominant species in the PPROM group.
In the PPROM group, Lactobacillus iners (relative abundance: 41.5% ± 39.6%; data shown as mean ± SD), followed by the Lactobacillus crispatus (relative abundance: 25.6% ± 38.1%; data shown as mean ± SD), and Gardnerella vaginalis (relative abundance: 6.9% ± 14.2%; data shown as mean ± SD) (Figure 1C) were the most abundant species. Less than half of the PPROM samples were dominated by Lactobacillus iners (41.7%), 29.2% were dominated by Lactobacillus crispatus, one sample was dominated by both Gardnerella vaginalis and Lactobacillus jensenii (2.1%), and 25.0% were not dominated by a single species (Figure 1D).
By comparing the proportion of dominant species in the two groups, we found that the proportion of Lactobacillus crispatus and Lactobacillus gasseri as the dominant taxa in group PPROM significantly decreased, while the proportion of Lactobacillus inners as the dominant taxa increased significantly compared with the term delivery control group (P<0.01, Fisher exact test).
Increased α-diversity and altered overall microbial composition in patients with PPROM
To evaluate the diversity, abundance, and uniformity of the vaginal microbial communities, we compared the Chao1, Observed species, Shannon, Simpson, Faith’s PD, and Pielou’s evenness indices between the groups. Significantly higher diversity and abundance were observed in the vaginal microbiota from the PPROM group compared with the control group, as measured by Chao1 (p = 1.8e-10) and Shannon (p = 1.3e-6) diversity indices (Kruskal-Wallis rank-sum test, Dunn post hoc test) (Figure 2A and Supplementary Figure S3A).
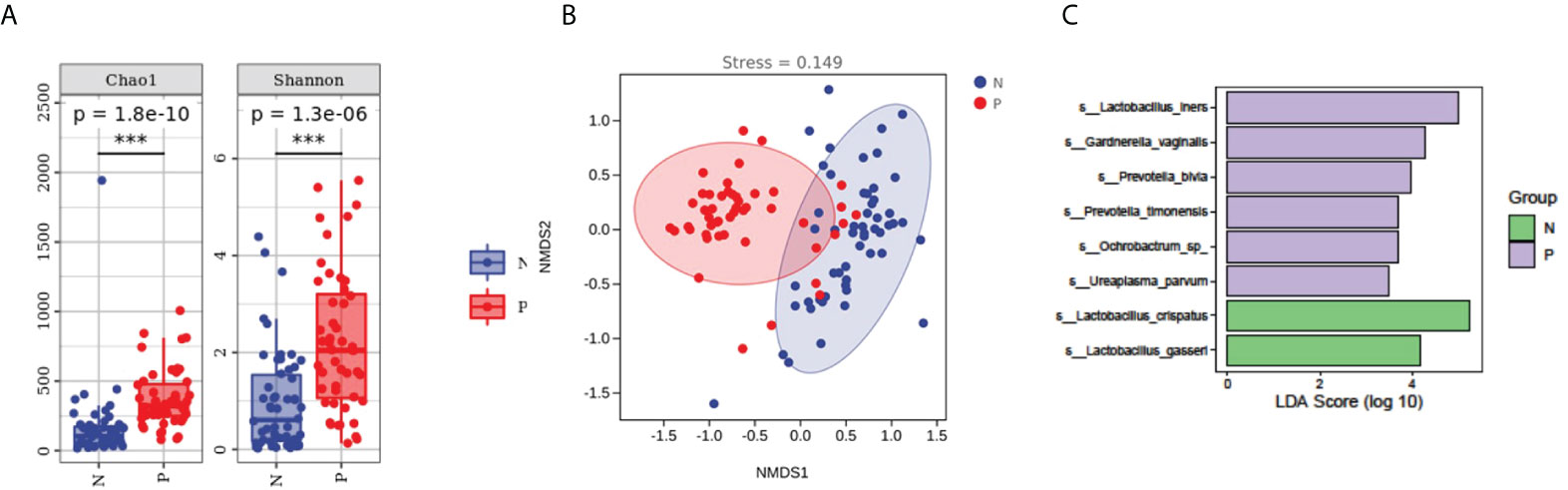
Figure 2 Comparisons of α- and β-diversities between the PPROM and term delivery groups. (A) Comparisons of α-diversity groups. ***It indicates that there is a significant difference between the two groups. (B) Comparisons of β-diversity between groups. (C) Differences in bacterial abundance in patients with PPROM versus controls.
We performed an NMDS analysis based on the Jaccard distance to assess the overall diversity in microbial composition. The results demonstrated a significant difference in vaginal microbial composition between the term delivery control and PPROM groups (p = 0.002; ANOSIM, stress = 0.0154; Figure 2B). PCoA based on Jaccard distance also demonstrated a significant difference between the two groups (pseudo-F: 6.53307, p = 0.002) (Supplementary Figure S2B).
Difference in bacterial abundance in patients with PPROM versus controls
We performed LEfSe analysis on the vaginal microbiota composition of the two groups to identify which taxa were significantly different.
The relative abundance of Lactobacillus iners, Gardnerella vaginalis, Prevotella bivia, Ochrobactrum sp, Prevotella timonensis, and Ureaplasma parvum were significantly higher in women with PPROM, whilst the abundance of Lactobacillus crispatus and Lactobacillus gasseri were lower compared to the term delivery group (using LDA score=3.5) (Figure 2C).
The vaginal microbiota-based signature associated with disease status
We assessed the potential value of vaginal microbiota as a biomarker for predicting the PPROM risk.Using the random forests algorithm, we found that the combination of Lactobacillus crispatus, Lactobacillus iners, Lactobacillus gasseri, Gardnerella vaginal, Prevotella bivia, Ochrobactrum sp., Prevotella timonensis, and Ureaplasma parvum could discriminate PPROM from controls, with an AUC of 91.3% (CI: 0.86–0.97) (Figure 3A). Additionally, using eight different species analyzed by LEfSe as predictors, and the generated AUC for ROCs (using SPSS 23), we found that Ochrobactrum sp. (AUC: 0.89, 95% CI: 0.81–0.96), Prevotella timonensis (AUC: 0.76, 95% CI: 0.67–0.86), and Gardnerella vaginal (AUC: 0.75, 95% CI: 0.65–0.84) could discriminate PPROM from controls (Figure 3B).
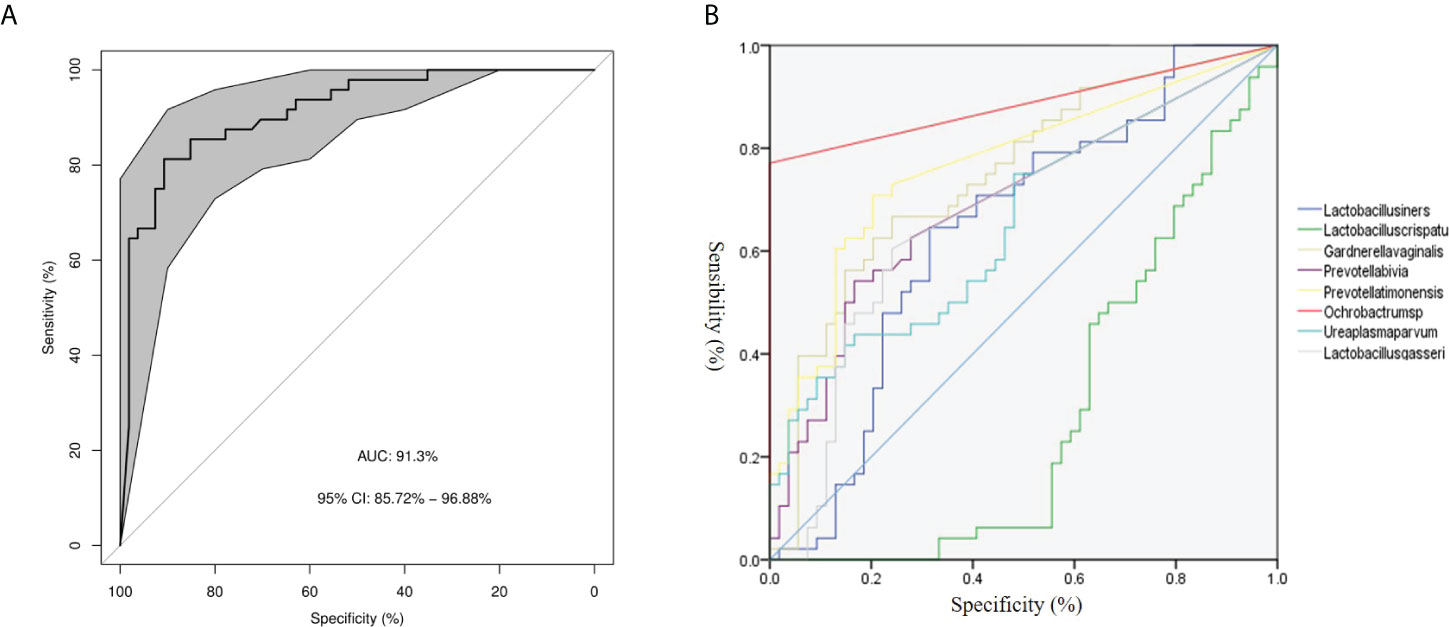
Figure 3 Disease classification based on vaginal microbiota signature. (A) The ROC curve of 8 different species. (B) The ROC curves of 8 different species.
Differential functions of bacteria in patients with PPROM versus controls
We performed a bacterial community network analysis in both groups to explore the bacterial interactions of key species in the vaginal ecosystem. The vaginal microbiota from term delivery women showed that Lactobacillus crispatus and Lactobacillus iners were located at key positions in the microbiota network. Other Lactobacillus species, such as Lactobacillus jensenii, had a close interaction with Lactobacillus crispatus and Lactobacillus iners (Figure 4A).
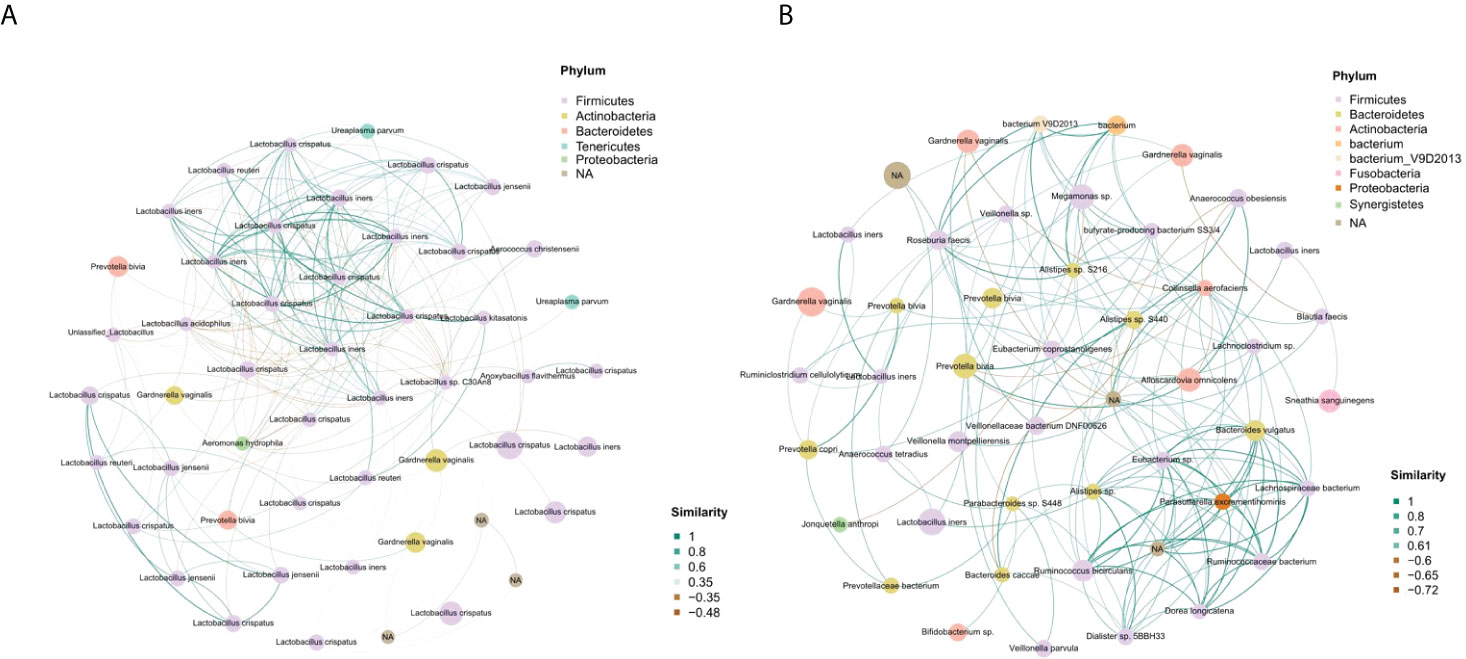
Figure 4 Identification of the vaginal microbial network. (A) The vaginal microbial network of the term delivery group. (B) The vaginal microbial network of the PPROM group.
In the PPROM group, Lactobacillus crispatus had no correlation with other species, and Lactobacillus iners formed a positive correlation function network with Gardnerella vaginalis, Prevotella sp., Veillonella montpellierensis and sneathia sanguinegens. Parasutterella excrementihominis was in the key position and showed a positive correlation fuction network with Bacteroides vulgatus, Eubacterium sp. and Ruminococcaceae bacterium (Figure 4B).
Overall, the microbial function in women with PPROM was distinct from that of women in the term delivery group (Figure 5A). Moreover, 50 metabolic pathways were significantly different between the PPROM group and term delivery group (P <0.05), of which 23 were significantly upregulated in the PPROM group compared to the control group (P <0.05; Figure 5A). The most affected pathways included PWY-6339, followed by PWY-6992 and PWY-7295.
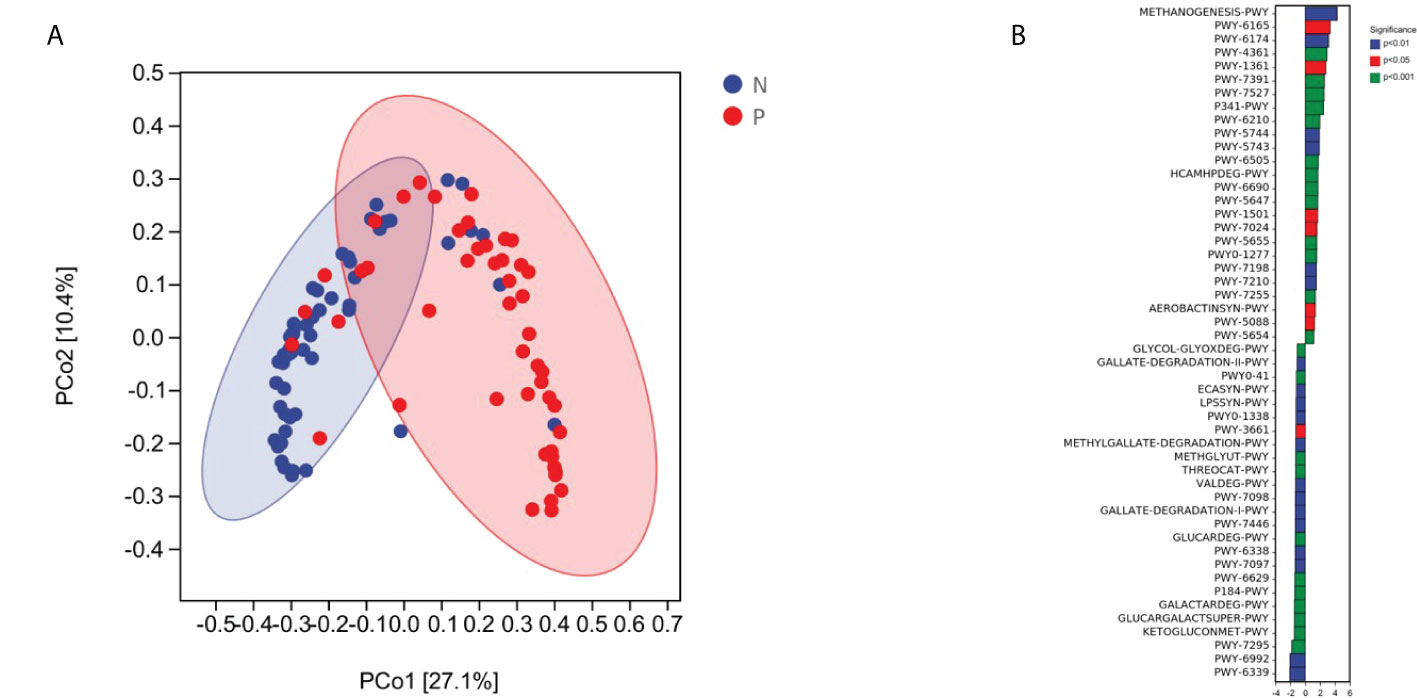
Figure 5 Comparisons of functions and metabolic pathways between the PPROM and term delivery groups. (A) Functional differences between groups were analyzed using principal coordinate analysis based on the Bray Curtis distance (p < 0.05). (B) Differential metabolic pathways identified by linear discriminant analysis effect size between groups.
Discussion
Previous studies have shown that vaginal microbiota is related to certain aspects of women’s health, such as preterm birth (Stout et al., 2017; Brown et al., 2019a). Studies conducted in Europe (Kindinger et al., 2017; Stafford et al., 2017) and North America (Callahan et al., 2017; Fettweis et al., 2019) on Caucasian populations have revealed positive associations between increased vaginal microbial diversity and risk of preterm birth; Gardnerella vaginalis, Sneathia spp., Prevotella spp., and Mollicutes spp. were identified as microbial markers and risk factors. Moreover, studies in the United States investigating African decent women reported decreased vaginal diversity as a risk factor for preterm birth (Nelson et al., 2016). Recent publications have also demonstrated a low Lactobacillus spp. dominance and high species diversity in vaginal microbial communities in women with PPROM (Brown et al., 2019b).
In this study, we used 16S rRNA gene sequencing to further explore the difference in vaginal microbiota in Chinese women with PPROM and in term delivery. We found high diversity and low Lactobacillus dominance in the PPROM group, which is consistent with previous studies.
The vaginal microbiota fluctuates according to circulating hormone levels during a woman’s lifespan. The increased estrogen level promotes the proliferation of vaginal epithelial cells and the deposition of glycogen, and the thickened vaginal epithelium produces lactic acid (Linhares et al., 2011), resulting in the decrease of vaginal pH value, which is suitable for the colonization of acidophilic bacteria, such as Lactobacillus spp. Lactobacillus spp metabolizes abundant glycogen in the vagina producing lactic acid isomers, further reducing the vaginal pH value. Very few other microorganisms could survive in such an acidic environment. Therefore, Lactobacillus spp becomes the dominant commensal in most individuals (Hickey et al., 2012). Furthermore, during pregnancy, the placenta secretes a large amount of estrogen, which reduces the diversity of vaginal microbiota and promotes Lactobacillus spp dominance (Siimes et al., 1983). Lactobacillus spp dominance is established through competitive exclusion and secretion of some chemicals, such as bacteriocin and biosurfactants. Such environment protects the host against the colonization of pathogens. Previous studies have shown that these pathogens are related to bacterial vaginosis, pelvic inflammatory diseases, vulvovaginal candidiasis, sexually transmitted diseases, human immunodeficiency virus infection, and high-risk human papillomavirus infection (Velraeds et al., 1998; Borgdorff et al., 2014; Zheng et al., 2015). Our study demonstrated that the reduced Lactobacillus spp abundance and Lactobacillus spp dominance are related to the colonization of pathogenic bacteria, which may cause PPROM.
We further studied the species of Lactobacillus spp. Compared with term delivery women, Lactobacillus iners relative abundance and dominance increased in the patients with PPROM, while the abundance and proportion of L. crispatus dominant bacteria decreased. Kacerovsky et al. studied the cervical microbiota of 61 patients with PPROM using hierarchical clustering methods and found 4 community state types (CSTS) of cervical microbiota: CSTI (L. crispatus dominance), CST III (L. iners dominance), CST IV-A (non-Lactobacillus dominance) and CST IV-B (G. vaginalis and sneathia sanguinegens dominance). Non-Lactobacillus CST type was associated with a strong cervicitis reaction and a high incidence rate of microbial invasion of the amniotic cavity, while CSTI was associated with the lowest level of inflammation and microbial amniotic cavity invasion. Our study showed that 41.7% of the PPROM samples were dominated by Lactobacillus iners, 29.2% of the PPROM samples were dominated by Lactobacillus crispatus, 2.1% of the PPROM samples were dominated by Gardnerella vaginalis, and 25.0% were not dominated by a single species, which corresponds respectively to CST III, CST I, CST IV-B, and CST IV-A.
We further studied the relationship between microorganisms of the vaginal microbiota. Lactobacillus crispatus and Lactobacillus iners were located at the key positions in the microbiota network of the term delivery group. Other Lactobacillus species, such as Lactobacillus jensenii, closely interacted with Lactobacillus crispatus and Lactobacillus iners. In the PPROM group, Lactobacillus crispatus did not correlate with other species, and Lactobacillus iners formed a positive correlation network with Gardnerella vaginalis, Prevotella sp., Veillonella montpellierensis, and sneathia sanguinegens. Therefore, the decrease of Lactobacillus crispatus dominance and the increase of Lactobacillus iners dominance may be one of the reasons for PPROM. We speculate that this may be caused due to the following reasons: due to the lack of gene encoding D-lactate dehydrogenase, Lactobacillus iners produces L-lactate and cannot maintain the acidity in the vagina, so it cannot provide sufficient protection to prevent vaginal dysbacteriosis (Saito et al., 2014). Compared with L-lactic acid, D-lactic acid has a stronger defense against potential pathogenic bacteria.
We also identified eight species that were significantly different in the vaginal microbiota of the two groups. Among them, decreased Lactobacillus crispatus and increased Lactobacillus iners were most related to the disease status, and the correlation between Ochrobactrum spp and PPROM has not been reported, which may be related to the ethnic specificity of the vaginal microbiota. ROC analysis of these eight differential species was further carried out to explore their clinical application. Ochrobactrum sp., Prevotella timonensis, and G. vaginalis can be used as biomarkers to distinguish the disease status of PPROM. The discovery of these biomarkers provides a theoretical foundation for predicting, preventing, and treating PPROM. The vaginal microbiota of pregnant women can be screened in the early and middle trimesters of pregnancy. If the above biomarkers are positive and the abundance of Lactobacillus crispatus decreases, patients can be regarded as a high-risk group and thus can be treated accordingly.
We screened eight metabolic pathways, including the super pathway of methylglyoxal degradation (METHGLYUT-PWY), nicotinamide adenine dinucleotide biosynthesis pathway II (NADSYN-PWY), L-tryptophan biosynthesis super pathway (PWY-6629), L-tryptophan degradation pathway (PWY-5655), L-valine degradation pathway I (VALDEG-PWY), polymyxin resistance pathway (PWY0-1338), L-valine degradation pathway I (VALDEG-PWY), and Enterobacter common antigen synthesis pathway (ECASYN-PWY), which are related to infection. We hypothesized that these pathways are related to the pathogenesis of PPROM; yet, this hypothesis needs to be confirmed by further experiments. The super pathway of methylglyoxal degradation of vaginal microbiota increased in patients with PPROM, which was involved in the degradation of methylglyoxal. Methylglyoxal is a dicarbonyl compound, which is a by-product of glycolysis by some bacteria. Studies have shown that neutrophils produce methylglyoxal as an antibacterial agent during bacterial infection, and the infection of group A streptococcus is related to the degradation of methylglyoxal. Therefore, we infer that the methylglyoxal degradation super pathway may participate in the occurrence of PPROM (Zhang et al., 2016). The nicotinamide adenine dinucleotide biosynthesis pathway II of vaginal microbiota in the PPROM group decreased. Nicotinamide is an important molecule found in all living cells. The regulation of NAD metabolism has been proved to be related to a variety of diseases, including cancer, neurodegenerative diseases, and inflammatory diseases. Mesquita et al. showed that its regulation is also related to host-pathogen interaction (Mesquita et al., 2016). Other studies also showed that NAD could inhibit Candida infection (Belenky et al., 2007). In the PPROM group, the L-tryptophan biosynthesis super pathway increased, and the L-tryptophan degradation pathway decreased. Studies showed that abnormal metabolism of L-tryptophan may be related to abnormal pregnancy and infection (Badawy et al., 2016). Another study demonstrated that tryptophan metabolism was related to urogenital Chlamydia infection (Bommana et al., 2021). The L-valine degradation pathway I increased in the vaginal microbiota of patients with PPROM. Studies showed that L-valine increased the phagocytosis of macrophages and effectively killed Gram-negative bacteria (Escherichia coli and Pseudomonas aeruginosa) and Gram-positive bacteria (Staphylococcus aureus) (Chen et al., 2017). The occurrence of PPROM may be related to L-valine degradation. The polymyxin resistance pathway in vaginal microbiota of patients with PPROM increased. Polymyxin (PMX) is a cationic peptide antibiotic that binds to Escherichia coli lipopolysaccharide (LPS) and prevents inflammatory activation. Studies showed that PMX was associated with partial remission of intrauterine inflammation (Saito et al., 2014). At the same time, the Enterobacter common antigen synthesis pathway (ECASYN-PWY) in vaginal microbiota of patients with PPROM increased. Studies fund that Enterobacter antigen is associated with urogenital infection during pregnancy (Naumann et al., 1979). To sum up, in this study, the metabolic pathway of vaginal microbiota of patients with PPROM significantly changed compared with pregnant women of term delivery group, and the changed metabolic pathways were mostly related to infection, suggesting that infection has an important role in the pathogenesis of PPROM.
The present study has several limitations. First, this is a cross-sectional study that lacks information on the dynamic changes of the vaginal microbiota in patients with PPROM. Furthermore, although significant differences between the PPROM group and term delivery controls were identified, samples from the PPROM group were taken after membrane rupture. Thus, the microbiota characteristics could be affected by other substances, such as bacteria on the membrane and amniotic fluid. However, multiple studies have shown that amniotic fluid is very similar to a sterile environment (Sacks et al., 2018). Moreover, this study showed that the average vaginal pH value of patients with PPROM was in the normal range, suggesting that PROM and amniotic fluid outflow had little effect on vaginal pH value of PPROM pregnant women. So the influence of amniotic fluid should be limited. The PPROM is difficult to predict, so it is difficult to obtain vaginal microbial samples immediately before PPROM. In some studies, vaginal microorganisms were sampled several times during pregnancy to observe the difference in vaginal microbiota between pregnant women with PPROM and normal pregnant women. Because the occurrence of PPROM is difficult to predict, the sampling at fixed time points may be far away from the occurrence of PPROM, and the vaginal microbiota may change greatly during this period. Therefore, the state of vaginal microbiota at the time of sampling may be difficult to reflect the situation of vaginal microbial composition at the time of PPROM, and there may be great variation in the results. At the same time, the incidence rate of PPROM is 2%-4%. In order to obtain a considerable number of samples of patients with PPROM, thousands of samples need to be studied, which increases the cost. This study collected vaginal microbial samples within 12 hours of PPROM and before applying antibiotics. Since this field is still in its infancy and there is little data on the vaginal microbiota of patients with PPROM in the Chinese population, we believe our study provides valuable information to the field.
Conclusion
This study characterized the changes in vaginal microbiota in women of Chinese nationality, comparing patients with PPROM with age- and gestational age-matched pregnant women who delivered at term. We identified several taxa that significantly increased in the vaginal microbiota in patients with PPROM, where altered signalling pathways were mainly related to infection. Our study provides valuable information on the correlation between vaginal microbiota and PPROM. Yet, further studies are required to determine this relationship’s underlying mechanisms and causality. Nevertheless, our findings provide valuable information to the field, concentrating on Chinese women with PPROM.
Data availability statement
The datasets presented in this study can be found in online repositories. The raw data has been uploaded to NCBI (Accession to cite for these SRA data: PRJNA792044).
Ethics statement
The studies involving human participants were reviewed and approved by Second Hospital of Shandong University, Jinan, Shandong. The patients/participants provided their written informed consent to participate in this study.
Author contributions
XD and YX are co-correspondence authors and contributed equally. XD designed, supervised the study and wrote the manuscript. YX performed the analysis and provided constructive discussions. CY as the first author performed the study, helped analyze the data, and contributed to writing the manuscript. FH contributed to data analyses and helped write the manuscript. GX and SD collected and prepared samples and helped write the manuscript. All authors have read and approved the final manuscript.
Funding
The project is supported by Yongping Xu Scientific Research and Development Fund (26010232007168).
Conflict of interest
The authors declare that the research was conducted in the absence of any commercial or financial relationships that could be construed as a potential conflict of interest.
Publisher’s note
All claims expressed in this article are solely those of the authors and do not necessarily represent those of their affiliated organizations, or those of the publisher, the editors and the reviewers. Any product that may be evaluated in this article, or claim that may be made by its manufacturer, is not guaranteed or endorsed by the publisher.
Supplementary material
The Supplementary Material for this article can be found online at: https://www.frontiersin.org/articles/10.3389/fcimb.2022.858732/full#supplementary-material
Supplementary Figure 1 | Species accumulation curve.
Supplementary Figure 2 | Vaginal microbiota composition and percentage of dominant taxa at the genus level. (A) Vaginal microbiota composition in the term delivery group at the genus level. (B) Percentage of the dominant genera in the term delivery group. (C) Vaginal microbiota composition in the PPROM group at the genus level. (D) Percentage of the dominant genera in the PPROM group.
Supplementary Figure 3 | Comparisons of α- and β-diversities between the PPROM and term delivery groups. (A) Comparisons of α-diversity between groups. (B) PCoA analysis based on Jaccard distance between groups.
References
Ananth, C. V., Oyelese, Y., Srinivas, N., Yeo, L., Vintzileos, A. M. (2004). Preterm premature rupture of membranes, intrauterine infection, and oligohydramnios: risk factors for placental abruption. Obstet. Gynecol. 104, 71–77. doi: 10.1097/01.AOG.0000128172.71408.a0
Badawy, A. A., Namboodiri, A. M., Moffett, J. R. (2016). The end of the road for the tryptophan depletion concept in pregnancy and infection. Clin. Sci. (London) 130, 1327–1333. doi: 10.1042/cs20160153
Baldwin, E. A., Walther-Antonio, M., MacLean, A. M., Gohl, D. M, Beckman, K. B, Chen, J., et al. (2015). Persistent microbial dysbiosis in preterm premature rupture of membranes from onset until delivery. PeerJ 3, e1398. doi: 10.7717/peerj.1398
Belenky, P., Bogan, K. L., Brenner, C. (2007). NAD+ metabolism in health and disease. Trends Biochem. Sci. 32, 12–19. doi: 10.1016/j.tibs.2006.11.006
Beydoun, S. N., Yasin, S. Y. (1986). Premature rupture of the membranes before 28 weeks: conservative management. Am. J. Obstet. Gynecol. 155, 471–479. doi: 10.1016/0002-9378(86)90257-7
Bokulich, N. A., Kaehler, B. D., Rideout, J. R., Dillon, M., Bolyen, E., Knight, R., et al. (2018). Optimizing taxonomic classification of marker-gene amplicon sequences with QIIME 2's q2-feature-classifier plugin. Microbiome 6, 90. doi: 10.1186/s40168-018-0470-z
Bolyen, E., Rideout, J. R. (2019). Reproducible, interactive, scalable and extensible microbiome data science using QIIME 2. Nat. Biotechnol. 37, 852–857. doi: 10.1038/s41587-019-0209-9
Bommana, S., Somboonna, N., Richards, G., Tarazkar, M., Dean, D. (2021). Tryptophan operon diversity reveals evolutionary trends among geographically disparate chlamydia trachomatis ocular and urogenital strains affecting tryptophan repressor and synthase function. mBio 12 (3), e00605–21. doi: 10.1128/mBio.00605-21
Borgdorff, H., Tsivtsivadze, E., Verhelst, R., Marzorati, M., Jurriaans, S., Ndayisaba, G. F., et al. (2014). Lactobacillus-dominated cervicovaginal microbiota associated with reduced HIV/STI prevalence and genital HIV viral load in African women. ISME J. 8, 1781–1793. doi: 10.1038/ismej.2014.2
Brown, R. G., Al-Memar, M., Marchesi, J. R., Lee, Y. S., Smith, A., Chan, D., et al. (2019a). Establishment of vaginal microbiota composition in early pregnancy and its association with subsequent preterm prelabor rupture of the fetal membranes. Trans. Res. J. Lab. Clin. Med. 207, 30–43. doi: 10.1016/j.trsl.2018.12.005
Brown, R. G., Chan, D., Terzidou, V., Lee, Y. S., Smith, A., Marchesi, J. R., et al. (2019b). Prospective observational study of vaginal microbiota pre- and post-rescue cervical cerclage. BJOG an Int. J. Obstet. Gynaecol. 126, 916–925. doi: 10.1111/1471-0528.15600
Brown, R. G., Marchesi, J. R., Lee, Y. S., Smith, A., Lehne, B., Kindinger, L. M., et al. (2018). Vaginal dysbiosis increases risk of preterm fetal membrane rupture, neonatal sepsis and is exacerbated by erythromycin. BMC Med. 16, 9. doi: 10.1186/s12916-017-0999-x
Callahan, B. J., DiGiulio, D. B., Goltsman, D. S. A., Sun, C. L., Costello, E. K., Jeganathan, P., et al. (2017). Replication and refinement of a vaginal microbial signature of preterm birth in two racially distinct cohorts of US women. Proc. Natl. Acad. Sci. U. S .A. 114, 9966–9971. doi: 10.1073/pnas.1705899114
Callahan, B. J., McMurdie, P. J., Rosen, M. J., Han, A. W., Johnson, A. J., Holmes, S. P. (2016). DADA2: High-resolution sample inference from illumina amplicon data. Nat. Methods 13, 581–583. doi: 10.1038/nmeth.3869
Chen, X. H., Liu, S. R., Peng, B., Li, D., Cheng, Z. X., Zhu, J. X., et al. (2017). Exogenous l-valine promotes phagocytosis to kill multidrug-resistant bacterial pathogens. Front. Immunol. 8. doi: 10.3389/fimmu.2017.00207
Coleman, J. S., Gaydos, C. A., Witter, F. (2013). Trichomonas vaginalis vaginitis in obstetrics and gynecology practice: new concepts and controversies. Obstetrical Gynecol. Survey 68, 43–50. doi: 10.1097/OGX.0b013e318279fb7d
Dale, P. O., Tanbo, T., Bendvold, E., Moe, N. (1989). Duration of the latency period in preterm premature rupture of the membranes. maternal and neonatal consequences of expectant management. Eur. J. Obstet. Gynecol. Reprod. Biol. 30, 257–262. doi: 10.1016/0028-2243(89)90010-5
DeSantis, T. Z., Hugenholtz, P., Larsen, N., Rojas, M., Brodie, E. L., Keller, K., et al. (2006). Greengenes, a chimera-checked 16S rRNA gene database and workbench compatible with ARB. Appl. Environ. Microbiol. 72, 5069–5072. doi: 10.1128/aem.03006-05
Fettweis, J. M., Serrano, M. G., Brooks, J. P., et al. (2019). The vaginal microbiome and preterm birth. Nat. Med. 25, 1012–1021. doi: 10.1038/s41591-019-0450-2
Fortner, K. B., Grotegut, C. A., Ransom, C. E., Bentley, R. C., Feng, L., Lan, L., et al. (2014). Bacteria localization and chorion thinning among preterm premature rupture of membranes. PloS One 9, e83338. doi: 10.1371/journal.pone.0083338
Fortunato, S. J., Menon, R., Lombardi, S. J. (2002). Role of tumor necrosis factor-alpha in the premature rupture of membranes and preterm labor pathways. Am. J. Obstet. Gynecol. 187, 1159–1162. doi: 10.1067/mob.2002.127457
Garite, T. J., Freeman, R. K. (1982). Chorioamnionitis in the preterm gestation. Obstet. Gynecol. 59, 539–545.
Helmig, B. R., Romero, R., Espinoza, J., Chaiworapongsa, T., Bujold, E., Gomez, R., et al. (2002). Neutrophil elastase and secretory leukocyte protease inhibitor in prelabor rupture of membranes, parturition and intra-amniotic infection. J. Maternal Fetal Neonatal Med. 12, 237–246. doi: 10.1080/jmf.12.4.237.246
Hickey, R. J., Zhou, X., Pierson, J. D., Ravel, J., Forney, L. J. (2012). Understanding vaginal microbiome complexity from an ecological perspective. Trans. Res. J. Lab. Clin. Med. 160, 267–282. doi: 10.1016/j.trsl.2012.02.008
Kacerovsky, M., Vrbacky, F., Kutova, R., Pliskova, L., Andrys, C., Musilova, I., et al. (2015). Cervical microbiota in women with preterm prelabor rupture of membranes. PLoS One 10, e0126884. doi: 10.1371/journal.pone.0126884
Katoh, K., Misawa, K., Kuma, K., Miyata, T. (2002). MAFFT: a novel method for rapid multiple sequence alignment based on fast Fourier transform. Nucleic Acids Res. 30, 3059–3066. doi: 10.1093/nar/gkf436
Kenyon, S., Boulvain, M., Neilson, J. P. (2013). Antibiotics for preterm rupture of membranes. Cochrane Database Syst. Rev. 12, Cd001058. doi: 10.1002/14651858.CD001058.pub3
Kindinger, L. M., Bennett, P. R., Lee, Y. S., Marchesi, J. R., Smith, A., Cacciatore, S., et al. (2017). The interaction between vaginal microbiota, cervical length, and vaginal progesterone treatment for preterm birth risk. Microbiome 5, 6. doi: 10.1186/s40168-016-0223-9
Linhares, I. M., Summers, P. R., Larsen, B., Giraldo, P. C., Witkin, S. S. (2011). Contemporary perspectives on vaginal pH and lactobacilli. Am. J. Obstet. Gynecol. 204, 120.e121–125. doi: 10.1016/j.ajog.2010.07.010
Lozupone, C. A., Hamady, M., Kelley, S. T., Knight, R. (2007). Quantitative and qualitative beta diversity measures lead to different insights into factors that structure microbial communities. Appl. Environ. Microbiol. 73, 1576–1585. doi: 10.1128/aem.01996-06
Lozupone, C., Knight, R. (2005). UniFrac: a new phylogenetic method for comparing microbial communities. Appl. Environ. Microbiol. 71, 8228–8235. doi: 10.1128/aem.71.12.8228-8235.2005
Mesquita, I., Varela, P., Belinha, A., Gaifem, J., Laforge, M., Vergnes, B., et al. (2016). Exploring NAD+ metabolism in host-pathogen interactions. Cell. Mol. Life Sci. CMLS 73, 1225–1236. doi: 10.1007/s00018-015-2119-4
Naumann, G., Budde, E., Nimmich, W., Herrmann, C., Scholz, B. (1979). [Common antigen for serological detection of urinary tract infections during pregnancy (author's transl)]. Zentralblatt fur Gynakologie 101, 1489–1494.
Nelson, D. B., Shin, H., Wu, J., Dominguez-Bello, M. G. (2016). The gestational vaginal microbiome and spontaneous preterm birth among nulliparous African American women. Am. J. Perinatol. 33, 887–893. doi: 10.1055/s-0036-1581057
O'Hanlon, D. E., Lanier, B. R., Moench, T. R., Cone, R. A. (2010). Cervicovaginal fluid and semen block the microbicidal activity of hydrogen peroxide produced by vaginal lactobacilli. BMC Infect. Dis. 10, 120. doi: 10.1186/1471-2334-10-120
Paramel Jayaprakash, T., Wagner, E. C., van Schalkwyk, J., Albert, A. Y., Hill, J. E., Money, D. M. (2016). High diversity and variability in the vaginal microbiome in women following preterm premature rupture of membranes (PPROM): A prospective cohort study. PloS One 11, e0166794. doi: 10.1371/journal.pone.0166794
Parry, S., Strauss, J. F., 3rd (1998). Premature rupture of the fetal membranes. New Engl. J. Med. 338, 663–670. doi: 10.1056/nejm199803053381006
Powell, J. M., Frank, Z. C., Clark, G. V., Lo, J. O., Caughey, A. B. (2021). Expectant management of preterm premature rupture of membranes at 34 weeks: a cost effectiveness analysis. J. Maternal Fetal Neonatal Med.: the official journal of the European Association of Perinatal Medicine, the Federation of Asia and Oceania Perinatal Societies, the International Society of Perinatal Obstetricians,, 1–9. doi: 10.1080/14767058.2021.2017874
Price, M. N., Dehal, P. S., Arkin, A. P. (2009). FastTree: computing large minimum evolution trees with profiles instead of a distance matrix. Mol. Biol. Evol. 26, 1641–1650. doi: 10.1093/molbev/msp077
Reinhold-Hurek, B., Bünger, W., Burbano, C. S., Sabale, M., Hurek, T. (2015). Roots shaping their microbiome: global hotspots for microbial activity. Annu. Rev. Phytopathol. 53, 403–424. doi: 10.1146/annurev-phyto-082712-102342
Sacks, D., Baxter, B., Campbell, B. C. V., Carpenter, J. S., Cognard, C., Dippel, D., et al. (2018). Multisociety consensus quality improvement revised consensus statement for endovascular therapy of acute ischemic stroke. Int. J. Stroke Off. J. Int. Stroke Soc. 13, 612–632. doi: 10.1177/1747493018778713
Saito, M., Payne, M. S., Miura, Y., Ireland, D. J., Stock, S., Kallapur, S. G., et al. (2014). Polymyxin b agonist capture therapy for intrauterine inflammation: proof-of-principle in a fetal ovine model. Reprod. Sci. 21, 623–631. doi: 10.1177/1933719113508820
Segata, N., Izard, J., Waldron, L., Gevers, D., Miropolsky, L., Garrett, W. S., et al. (2011). Metagenomic biomarker discovery and explanation. Genome Biol. 12, R60. doi: 10.1186/gb-2011-12-6-r60
Shobokshi, A., Shaarawy, M. (2002). Maternal serum and amniotic fluid cytokines in patients with preterm premature rupture of membranes with and without intrauterine infection. Int. J. Gynaecol. Obstet. 79, 209–215. doi: 10.1016/s0020-7292(02)00238-2
Siimes, A. S., Immonen, I., Stenman, U. H., Kärkkäinen, J. E., Pekonen, F., Fyhrquist, F. Y. (1983). Plasma renin substrate in the prediction of pregnancy outcome in threatened abortion. Br. J. Obstet. Gynaecol. 90, 1186–1192. doi: 10.1111/j.1471-0528.1983.tb06470.x
Stafford, G. P., Parker, J. L., Amabebe, E., Kistler, J., Reynolds, S., Stern, V., et al. (2017). Spontaneous preterm birth is associated with differential expression of vaginal metabolites by lactobacilli-dominated microflora. Front. Physiol. 8. doi: 10.3389/fphys.2017.00615
Stout, M. J., Zhou, Y., Wylie, K. M., Tarr, P. I., Macones, G. A., Tuuli, M. G. (2017). Early pregnancy vaginal microbiome trends and preterm birth. Am. J. Obstet. Gynecol. 217, 356.e351–356.e318. doi: 10.1016/j.ajog.2017.05.030
Turnbaugh, P. J., Ley, R. E., Hamady, M., Fraser-Liggett, C. M., Knight, R., Gordon, J. I. (2007). The human microbiome project. Nature 449, 804–810. doi: 10.1038/nature06244
Velraeds, M. M., van de Belt-Gritter, B., van der Mei, H. C., Reid, G., Busscher, H. J. (1998). Interference in initial adhesion of uropathogenic bacteria and yeasts to silicone rubber by a lactobacillus acidophilus biosurfactant. J. Med. Microbiol. 47, 1081–1085. doi: 10.1099/00222615-47-12-1081
Yoon, B. H., Romero, R., Park, J. S., Kim, C. J., Kim, S. H., Choi, J. H., et al. (2000). Fetal exposure to an intra-amniotic inflammation and the development of cerebral palsy at the age of three years. Am. J. Obstet. Gynecol. 182, 675–681. doi: 10.1067/mob.2000.104207
Zhang, M. M., Ong, C. L., Walker, M. J., McEwan, A. G. (2016). Defence against methylglyoxal in group a streptococcus: a role for glyoxylase I in bacterial virulence and survival in neutrophils? Pathog. Dis. 74, 2. doi: 10.1093/femspd/ftv122
Keywords: PPROM, PROM, vaginal microbiota, PTB, preterm birth
Citation: Yan C, Hong F, Xin G, Duan S, Deng X and Xu Y (2022) Alterations in the vaginal microbiota of patients with preterm premature rupture of membranes. Front. Cell. Infect. Microbiol. 12:858732. doi: 10.3389/fcimb.2022.858732
Received: 20 January 2022; Accepted: 15 July 2022;
Published: 08 August 2022.
Edited by:
George Seghal Kiran, Pondicherry University, IndiaReviewed by:
Jose Eleuterio Junior, Federal University of Ceara, BrazilRichard Brown, Imperial College London, United Kingdom
Copyright © 2022 Yan, Hong, Xin, Duan, Deng and Xu. This is an open-access article distributed under the terms of the Creative Commons Attribution License (CC BY). The use, distribution or reproduction in other forums is permitted, provided the original author(s) and the copyright owner(s) are credited and that the original publication in this journal is cited, in accordance with accepted academic practice. No use, distribution or reproduction is permitted which does not comply with these terms.
*Correspondence: Xiaohui Deng, dxh@sdu.edu.cn; Yongping Xu, xyp1117@sdu.edu.cn