The interactions between oral-gut axis microbiota and Helicobacter pylori
- 1State Key Laboratory of Oral Diseases & National Clinical Research Center for Oral Diseases & West China School of Stomatology, Sichuan University, Chengdu, China
- 2Department of Operative Dentistry and Endodontics, West China Hospital of Stomatology, Sichuan University, Chengdu, China
In the human body, each microbial habitat exhibits a different microbial population pattern, and these distinctive microflorae are highly related to the development of diseases. The microbial interactions from host different niches are becoming crucial regulators to shape the microbiota and their physiological or pathological functions. The oral cavity and gut are the most complex and interdependent microbial habitats. Helicobacter pylori is one of the most important pathogens from digestive tract, especially the stomach, due to its direct relationships with many gastric diseases including gastric cancer. H. pylori infections can destroy the normal gastric environment and make the stomach a livable channel to enhance the microbial interactions between oral cavity and gut, thus reshaping the oral and gut microbiomes. H. pylori can be also detected in the oral and gut, while the interaction between the oral-gut axis microbiota and H. pylori plays a major role in H. pylori’s colonization, infection, and pathogenicity. Both the infection and eradication of H. pylori and its interaction with oral-gut axis microbiota can alter the balance of the microecology of the oral-gut axis, which can affect the occurrence and progress of related diseases. The shift of oral-gut axis microbiota and their interactions with H. pylori maybe potential targets for H. pylori infectious diagnosis and treatment.
Introduction
The balance and dysbiosis of the human microbiome are inextricably associated with health and disease (Hou et al., 2021). There are many different and specific microbial habitats in the human body. Each microbial habitat shows a different microbial population pattern, and the microbial interactions within the same niche or from different niches are important for the microecological balance and host health (Baquero et al., 2021). The oral cavity and gut are the most complex microbial habitats. The interaction between oral and gut microbiota is complicated, unstable, and interconnected (Acharya et al., 2017). Under normal physiological conditions, they can maintain a fine-tuned balance, but the imbalance of crosstalk will contribute to the occurrence and development of diseases (Albuquerque-Souza and Sahingur, 2022).
The transmission of oral to gut and gut to oral microorganisms can shape and/or reshape the microbial ecosystem in both habitats and thus regulate the pathogenesis of different diseases (Park et al., 2021), especially in cases of oral-gut barrier damage (Khor et al., 2021). The composition of the gut microbiota was similar to the oral microbiome under low gastric acidity condition caused by the long-term usage of proton pump inhibitors and the urease produced by infected Helicobacter pylori, further suggesting the interorgan translocation of the oral and gut microbiota due to the oral-gut barrier dysfunction (Park et al., 2021). H. pylori, a gram-negative human pathogen, has infected approximately fifty percent of humans worldwide (Lee et al., 2022). It is one of the most studied bacteria which can survive stably in the gastric acid environment and has co-evolved with humans for thousands of years (Kang and Blaser, 2006). Due to its strong correlation with gastric cancer, the World Health Organization’s International Agency for Research on Cancer (IARC) classified H. pylori as “Group 1” carcinogen to humans. H. pylori infection was capable to change the pH of the gastric environment (Camilo et al., 2017), and it can transmit through the oral-oral and fecal-oral route (Mégraud, 1995) to cause gastric diseases including chronic gastritis, gastric ulcer, gastric adenocarcinoma, etc. (Tsay and Hsu, 2018). The abundance of H. pylori in the mouth is very low compared to that in the stomach as it constituted approximately 42% – 97% of the total gastric bacterial community (Schulz et al., 2018), but H. pylori can significantly affect the oral community while some other microorganisms can also affect its colonization in the oral cavity (Vasapolli et al., 2019). H. pylori affects the microbiota and diseases of the oral-gut axis (Mladenova and Durazzo, 2018) as it connects the entire gastrointestinal tract through its transmission route from mouth to the stomach.
Helicobacter pylori and oral microbiome
Oral microbiome composition
As the initiation point of digestion, the oral cavity with its unique niches, such as the gingival sulcus, the tongue, the hard and soft palates, the saliva, and the teeth, is an exceptionally complex habitat that presents over 700 species of microorganisms including bacteria, fungi, viruses, and protozoa (Mark Welch et al., 2019). Actinobacteria, Bacteroidetes, Firmicutes, Proteobacteria, TM7 (Saccharibacteria), and Spirochaetes are common bacteria at the phylum level, while Fusobacterium, Gemella, Haemophilus, Neisseria, Porphyromonas, Prevotella, Streptococcus, Veillonella, Actinomyces, Alloprevotella, Pseudomonas, Treponema, Solobacterium are common at the genus level, which can be found in all the oral sites of healthy subjects (Sharma et al., 2018b; Park et al., 2021). In particular, Streptococcus was the most abundant genus which represented 12% to 66% of the total genera detected in the oral cavity. The abundance of Neisseria, Prevotella, and Haemophilus genera were also high, counting for 6% to 29% of the total bacteria detected (Caselli et al., 2020). The oral microbiome is maintained in homeostasis in the healthy state of the host (Lamont et al., 2018), but the occurrence of host diseases will lead to the imbalance of oral flora, indicating that the oral microbiome can directly reflect the host health conditions (Hakansson et al., 2018).
Effects of oral microbiome on Helicobacter pylori
The oral and gastric environments are linked together by saliva and digested food (Freitas et al., 2018). A recent study indicated that oral microbiome was the main source of gastric microbes and was closely related to the infection and transmission of H. pylori (Wu et al., 2021) (Table 1), and oral microorganisms can impact the transmission and colonization of H. pylori (Kivi and Tindberg, 2006). The main interaction patterns are coaggregation, symbiotic biofilm formation, endosymbiosis, etc. (Nobbs and Jenkinson, 2015; Chen et al., 2021b) (Figure 1). Fusobacterium nucleatum and Porphyromonas gingivalis, key bacteria in periodontal diseases, can aggregate with H. pylori cells and the coaggregation was inhibited by EDTA, lysine, or arginine in vitro, indicating the potential promotion of H. pylori oral-to-stomach colonization by oral bacteria (Okuda et al., 2003; Park et al., 2016). Streptococcus mutans, the major cariogenic bacterium, can form a symbiotic biofilm with H. pylori to increase its survival in the unsuitable environment of the mouth (Nomura et al., 2020).
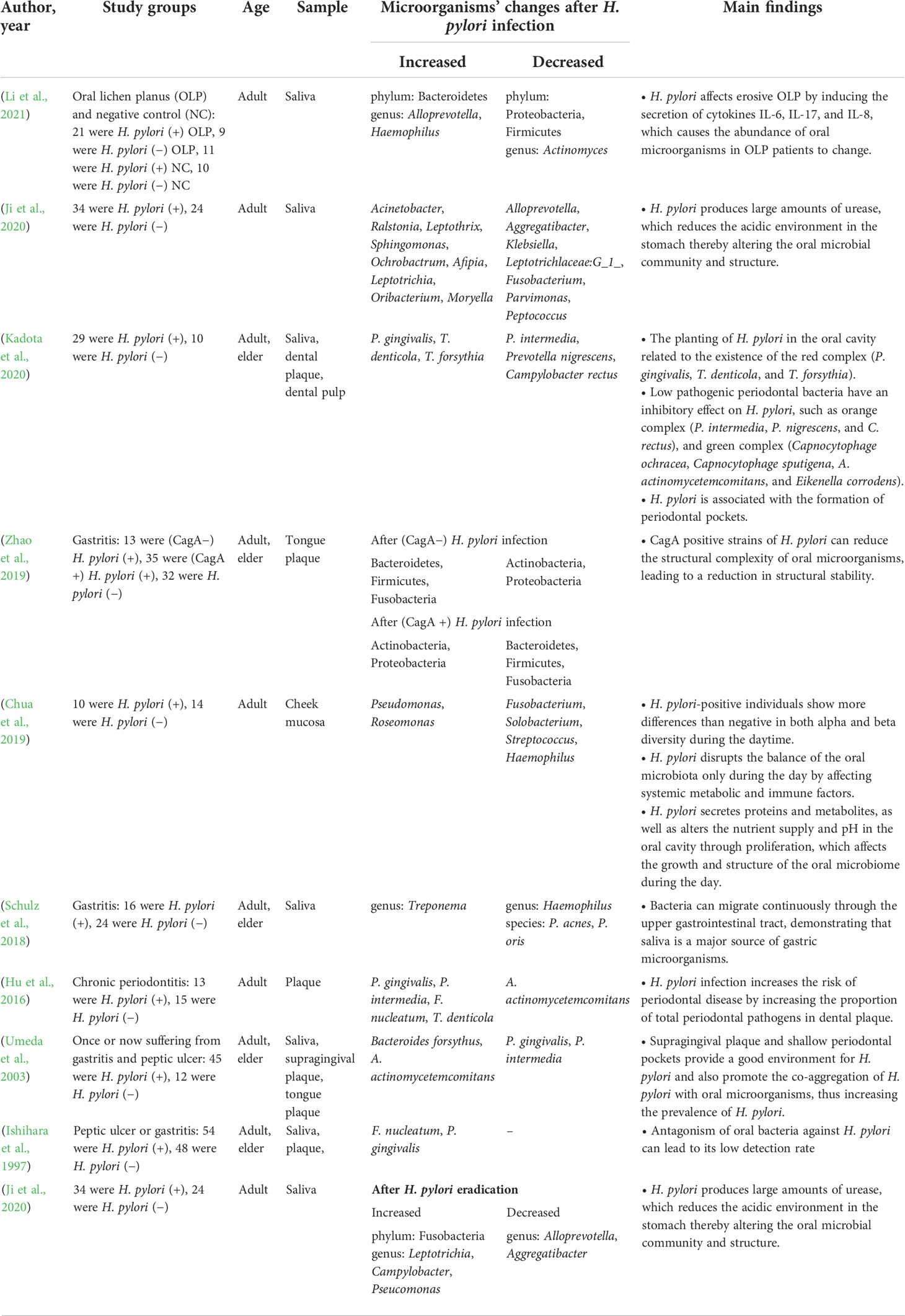
Table 1 Studies assessing the influence of Helicobacter pylori (H. pylori) infection on oral microbiota.
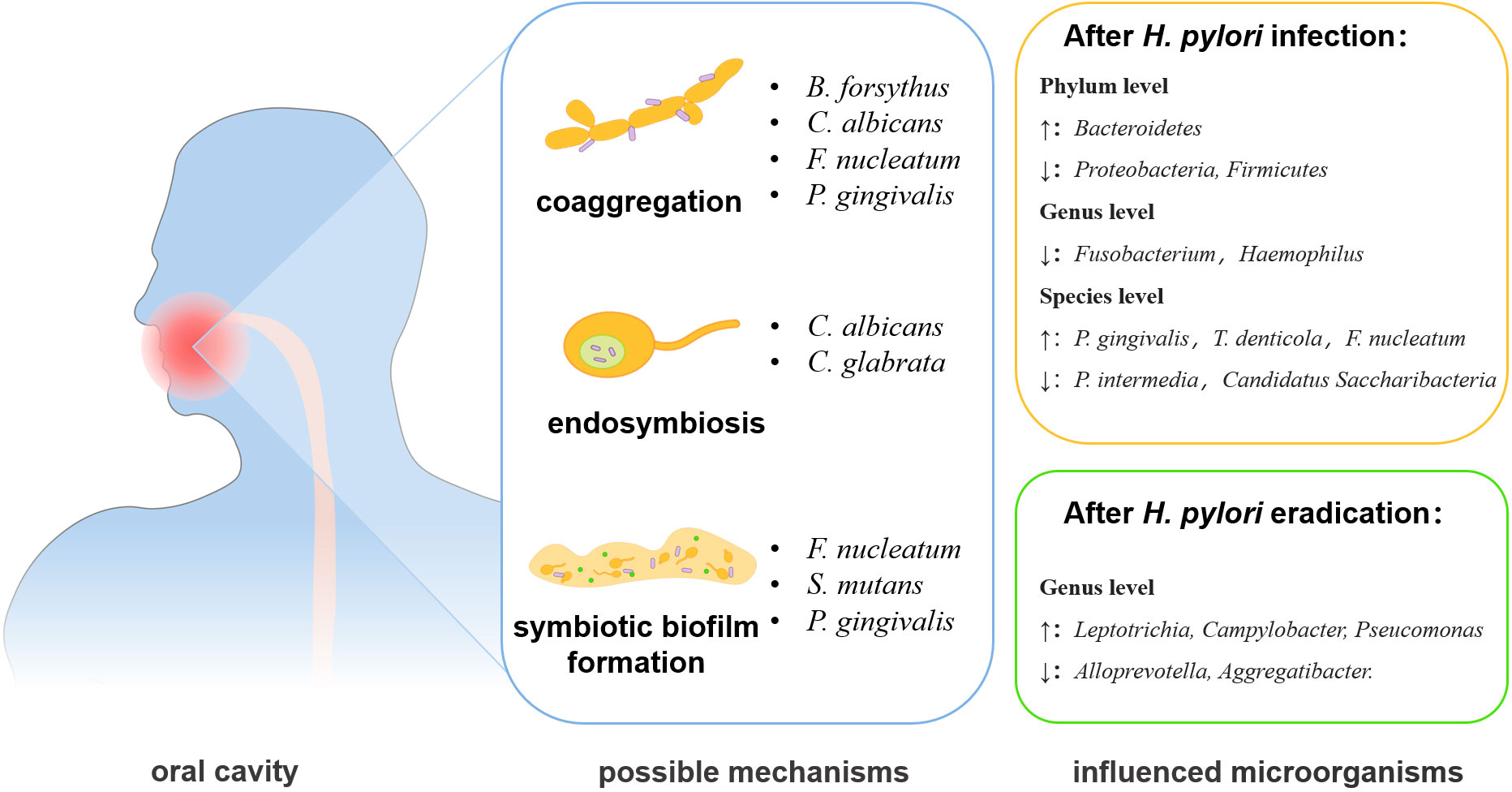
Figure 1 The changes and interactional mechanisms of H. pylori and oral microbiota. The interactions between H. pylori and oral microbiome may act through co-aggregation, endosymbiosis, and formation of symbiotic biofilm. The eradication of H. pylori infection can also affect the oral microbiota.
Candida albicans is the most common fungus in the human body and its main habitats are the oral cavity, upper respiratory tract, and intestinal tract (D'Enfert et al., 2020). C. albicans can synergize with H. pylori to enhance its survival in an unfavorable living environment and promote its colonization and the infection (Chen et al., 2021b). H. pylori was found to enter C. albicans yeast cells in the oral cavity and vagina, while the intracellular H. pylori showed active motility even under high temperature, dryness, and antibiotics conditions (Saniee et al., 2013), indicating that the internalization synergistic relationship can protect H. pylori from unsuitable conditions. H. pylori can also anchor on the surface of the C. albicans and aggregate with C. albicans to form a mixed biofilm (Palencia et al., 2022).
Besides the synergistic interaction between H. pylori and oral microorganisms, some oral bacterial strains showed a hostile relationship with H. pylori, such as S. mutans JP2 and Ingbritt, Streptococcus sobrinus 6715, and three Prevotella species significantly inhibited the growth of H. pylori in vitro, and this growth inhibitory activity was affected by heat and protease treatments (Ishihara et al., 1997).
Effect of Helicobacter pylori on the oral microbiome
H. pylori infection can disrupt oral microbiome homeostasis through the interplay with multiple members of the oral microbial community, such as H. pylori supernatant could inhibit S. mutans and Streptococcus sanguinis dual-species biofilm formation and their EPS production in vitro studies, but enhance the acid production of S. mutans to increase the abundance of S. mutans in this acidic condition as S. mutans is more acid resistant than S. sanguinis (Zhang et al., 2018). However, H. pylori-induced oral microbiome changes may differ under different oral samples and various host health conditions. Without oral and gastrointestinal diseases, several studies have determined the different changes of the microflora caused by H. pylori from different oral ecological niches. By sequencing the bacterial 16S rRNA gene V3-V4 hypervariable regions in saliva samples, the alpha diversity of H. pylori-infected subjects was similar to that of uninfected subjects, but for buccal swab samples, α and β diversity changed significantly in H. pylori-positive individuals compared to H. pylori-negative individuals (Ji et al., 2020). H. pylori infection had a significant effect on the abundance of both Pseudomonas and Rosemonas genera and significantly decreased the abundance of Haemophilus, and Streptococcus in cheek mucosa samples, but the saliva samples showed no significant changes (Chua et al., 2019). These results indicate that H. pylori infection showed different effects in oral niches.
The interaction between H. pylori and oral microorganisms can differ from that of asymptomatic H. pylori-positive people with oral disease or gastrointestinal disease. The increase of certain oral bacteria was positively correlated with the colonization of H. pylori. For example, the Bacteroidetes at phylum level increased in Oral lichen planus (OLP) patients with H. pylori infection, while there was a positive correlation between H. pylori infection and the relative abundance of Haemophilus and Alloprevotella at the genus level (Li et al., 2021). When H. pylori infection was accompanied by gastrointestinal diseases, such as gastritis, an increase in Treponema at the genus level was detected in the oral cavity (Schulz et al., 2018), but some oral microorganisms showed opposite changes in abundance, such as the decreasing trend of Haemophilus at the genus level, Propionibacterium acnes, Prevotella oris, P. gingivalis, and Prevotella intermedia at the species level were also observed in gastrointestinal disorders patients with H. pylori infection, indicating the different interaction between H. pylori and gastrointestinal diseases on oral microbiome (Umeda et al., 2003; Schulz et al., 2018).
The expression of virulence factors of H. pylori may also affect the oral microbiome. The abundance of Actinobacteria and Proteobacteria was increased, while the abundance of Bacteroidetes, Firmicutes, and Fusobacteria was decreased from the tongue plaque samples of CagA-positive H. pylori-infected patients, but these changes were totally reversed in the CagA-negative H. pylori-infected patients (Zhao et al., 2019).
The H. pylori eradication can also cause the changes of oral microbiome. In saliva samples from patients without severe oral diseases, such as periodontitis and OLP, the eradication treatment of H. pylori decreased the salivary bacterial diversity, but the genera Lautropia, Burkholderiales, Burkholderiaceae, and Actinomyces were enriched (Ji et al., 2022). Another study found that the eradication of H. pylori was followed by a relative increase of most oral bacteria, including Ralstonia, Leptotrichia, Sphingomonas, Leptothrix, Oribacterium, and Acinetobacter, except for Ochrobactrum (Ji et al., 2020). More importantly, the experiment also revealed that H. pylori eradication exacerbates the changes in oral microorganisms caused by H. pylori infection, for example, Alloprevotella, Aggregatibacter, Leptotrichlaceae:G_1_, Parvimonas, and Fusobacterium would further decrease in number (Ji et al., 2020). In conclusion, the infection/eradication of H. pylori can change the structure of oral microorganisms, and thus may affect the development and progress of oral diseases.
Oral diseases and Helicobacter pylori
The infection of H. pylori is highly correlated with oral diseases (Perez-Perez et al., 2004; Anand et al., 2006), and the shift of oral microbial community induced by H. pylori is one of the potent reasons for the oral diseases (Martin and Solnick, 2014). Periodontitis, a common microbiome-driven inflammatory disease (Hajishengallis, 2022), was highly related to H. pylori infection. P. gingivalis, as an established pathogenic agent of periodontitis (Miller and Scott, 2021), had a positive correlation with H. pylori. In chronic periodontitis patients with H. pylori infection, the red complex associated with periodontal disease was significantly increased in plaque, including P. gingivalis, Treponema denticola, Tannerella forsythia (Hu et al., 2016), indicating that H. pylori infection may promote periodontal disease. However, the orange and green complex showed low abundance in H. pylori-positive individuals (Kadota et al., 2020). H. pylori and increased P. gingivalis due to H. pylori infection, can both produce heat shock protein 60 (HSP60), which can target human HSP60 and aggravate the progression of periodontitis (Matsuura et al., 2008; Rizzo et al., 2012). H. pylori infection was also closely correlated to the erosive OLP (Li et al., 2021) as H. pylori infection increased the production of inflammatory cytokines IL-6 and IL-8, while these inflammatory cytokines may regulate the oral immune microenvironment through blood to exacerbate the inflammatory response in oral cavity (Du Teil Espina et al., 2019). There was also a strong correlation between H. pylori infection and halitosis, but the mechanism was not clear as it was difficult to determine whether the halitosis was induced by H. pylori in the stomach or caused by the changes of oral microflora induced by H. pylori infection (Anbari et al., 2019). A study analyzed the correlation between Behçet’s syndrome (BS) and H. pylori infection and found that BS patients had a higher rate of H. pylori infection, and the clinical symptoms including oral ulceration, genital ulceration, and cutaneous lesions could be improved after H. pylori eradication (Yu et al., 2019). However, its specific mechanism remains to be explored.
H. pylori infection is closely related to a variety of oral diseases, but traditional antibiotic therapy is increasingly difficult to eradicate H. pylori in the stomach, however, periodontal therapy, adjunctive treatment of traditional antibiotic therapy, had been shown to play an important role in the eradication of gastric H. pylori (Ren et al., 2016). It can also effectively reduce the oral pathogenic bacteria enriched by H. pylori infection to achieve the prevention and treatment of oral diseases (Okuda et al., 2003).
Helicobacter pylori and gut microbiome
Gut microbiome
The gut is the largest microbial ecosystem in the human body which contains approximately 500 to 1000 species in more than 50 different phyla (Qin et al., 2010). The human gut microbiome is established early in life and can be altered by host diet, lifestyle factors, and health status (Shanahan et al., 2021). The childhood/adolescent gut communities are enriched in Bifidobacterium spp., Faecalibacterium spp., and members of the Lachnospiraceae family (Hollister et al., 2015). In contrast, the adult gut microbiome is more stable, and it is seemed that the environmental factors play a much greater role than genetic factors (Spor et al., 2011). The gut microbiota, mainly anaerobic, consists of five major phyla including Actinobacteria, Bacteroidetes, Firmicutes, Proteobacteria, and Verrucomicrobia, while Cyanobacteria and Fusobacteria are presented in a minor proportion (Sekirov et al., 2010; Ghosh and Pramanik, 2021). Various disease states are directly related to the gut microbial diversity and their functions (Baruch et al., 2021; Davar et al., 2021), therefore, gut microbiota can reflect host age, health conditions, behaviors, and lifestyles (Zmora et al., 2019).
Effect of Helicobacter pylori on the gut microbiome
As an important member of the gut ecosystem, H. pylori can influence the gastrointestinal microbiota through host-microbial or microbial-microbial interactions (Schulz et al., 2018; Noto et al., 2019) (Table 2). H. pylori can regulate the gut microenvironment in different ways (Figure 2). The virulence factors secreted by H. pylori may affect the gut microbiota. In a transgenic Drosophila model heterologously expressed the H. pylori virulence factor CagA, the expression of CagA alone shifted the gut microbial community, such as the proliferation of Lactobacillus brevis was significantly increased (Jones et al., 2017). H. pylori infection could also induce the secretion of different gastrointestinal hormones (He et al., 2016). The increased gastrin release was found in H. pylori-positive subjects and the hormone levels remodeled the intestinal metabolism, thereby affecting the gut microbiome, such as the level of leptin was positively correlated with the quantity of Bifidobacterium and Lactobacillus (Mohammadi et al., 2020). H. pylori may also influence the abundance of Lactobacillus, Allobaculum, Turicibacter, and Anaeroplasma by elevating the secretion of ghrelin (Kienesberger et al., 2016). H. pylori can affect the gut microbiota by changing the pH of the colonic environment. H. pylori decreased the acidity of the colon to cause a decrease in Bacteroidetes and an increase in Firmicutes and Proteobacteria in the fecal microbiota (Gao et al., 2018; Hold and Hansen, 2019). The infection of H. pylori was accompanied by an increase of Lactobacillus salivarius and a decrease of Lactobacillus acidophilus, which was also related to the decrease of gastric acid secretion (Iino et al., 2018). H. pylori infection may also modulate the immune response to affect the gut microbiota (Ge et al., 2018). H. pylori-positive patients exhibited a decreased number of short-chain fatty acids (SCFAs) producing gut bacteria, which played important roles in modulating intestinal homeostasis (Smith et al., 2013), leading to the enrichment of Prevotella copri (Sitkin et al., 2022). In addition, H. pylori infection also affected the growth of many gut microorganisms, commonly including Desulfovibrio, Prevotella, Haemophilus, Bacteroides, Parasutterella, Pseudoflavonifractor at the genus level, Candida glabrata, Enterobacter cloacae, Klebsiella pneumoniae, Sutterella wadsworthensis, Bacteroides vulgatus, Escherichia coli at the species level (Dash et al., 2019; Frost et al., 2019; Martín-Núñez et al., 2019; Wang et al., 2019), but the mechanisms remain to be explored.
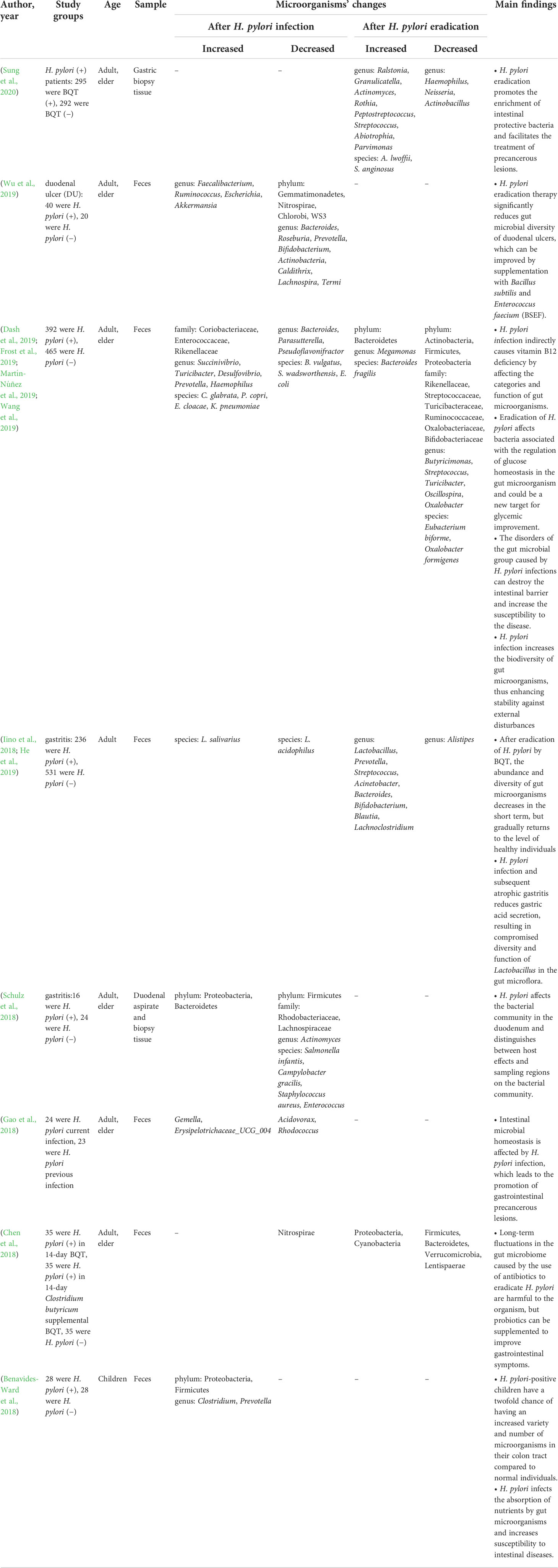
Table 2 Studies assessing the influence of Helicobacter pylori (H. pylori) infection on gut microbiota.
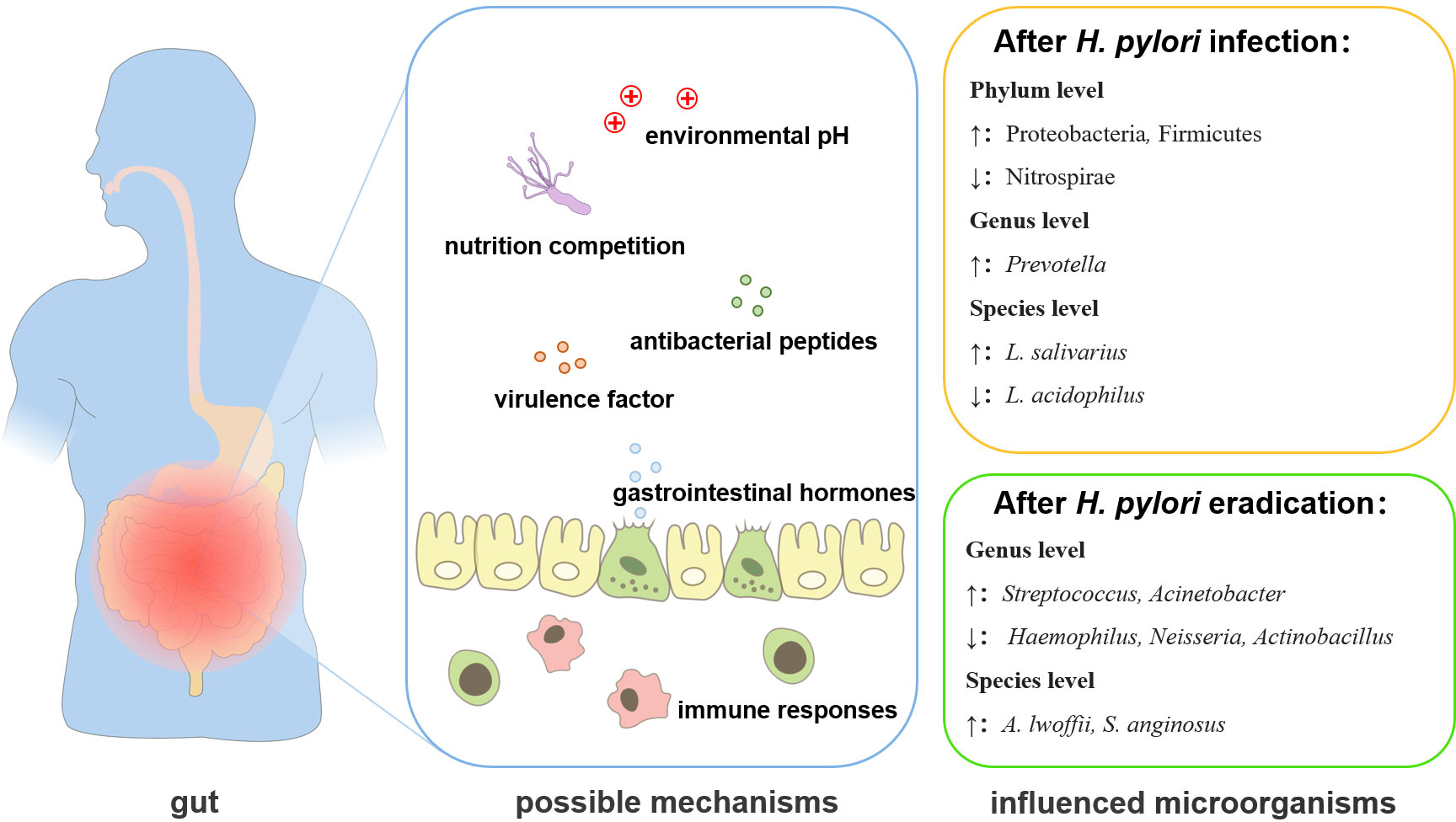
Figure 2 The changes and interactional mechanisms of H. pylori and gut microbiota. H. pylori infection can regulate the gut microbiota through 1. secretion of virulence factors; 2. mobilizing antibacterial peptides; 3. nutrition competition; 4. inducing or reducing the secretion of gastrointestinal hormones; 5. changing the pH of the environment; 6. affecting the immune response.7. eradication of infection.
H. pylori eradication also affects gut bacteria through different mechanisms. For example, H. pylori may compete for the nutrition to affect the gut microbiota, as the capacities of nutrient metabolism were restored after the eradication of H. pylori, and the abundance of Actinobacteria, Bacteroidetes, Firmicutes, and Fusobacteria at the phylum level, Lactobacillus, and Bifidobacterium at the genus level were increased significantly (Tao et al., 2020). After the eradication of H. pylori, the pH in the stomach significantly decreased, which led to the decrease in the Bacteroidetes-to-Firmicutes ratio and the enrichment of Bifidobacterium-related taxa in gastrointestinal microbiota (Guo et al., 2020). The eradication of H. pylori was usually achieved by bismuth-containing quadruple therapy (BQT) (Rimbara et al., 2011). However, H. pylori eradication with BQT also reshaped the structure of the gut microbiota, including changes in bacterial abundance at the genus level and species level (He et al., 2019; Martín-Núñez et al., 2019; Sung et al., 2020). After the BQT, most of the changed bacteria returned to normal levels, except for those belonging to Ruminococcaceae, Lachnospiraceae, and Eubacterium, as some beneficial bacteria belonged to Lachnospiraceae and Ruminococcaceae, and the major butyrate-producing bacteria were still kept in a decreased level (Chen et al., 2018). Some pathogenic bacteria such as Acinetobacter baumannii NIPH60, Klebsiella sp., and Haemophilus sp. were also increased After the BQT (Chen et al., 2018). It is also worth noting that probiotic supplementation in BQT for H. pylori eradication, reversed the long-term influence in the gut microbiome caused by the use of antibiotics and improved gastrointestinal symptoms, although no significant differences in eradication rates were observed (Chen et al., 2018). However, whether the changes in gut microbiology after H. pylori eradication are related to H. pylori itself or BQT is still unclear and needs to be further investigated.
Gut diseases and Helicobacter pylori
The disturbance of the oral-gut axis microbiota is closely related to the occurrence and development of gut diseases, including inflammatory bowel disease (IBD) and colorectal cancer (CRC), etc. (Park et al., 2021), while H. pylori infection enriched some bacteria played key roles in gut diseases, such as F. nucleatum, P. gingivalis, and so on. IBD, including Crohn’s disease (CD) and ulcerative colitis (UC), is closely linked with oral-gut axis microbiome dysbiosis (Lee and Chang, 2021). IBD patients exhibited the increase of intestinal epithelial permeability due to the impacts on mucosal barrier (Sharma et al., 2018a). F. nucleatum, a common bacterium in oral cavity, which can be increased after H. pylori infection, was significantly enriched in the gut of IBD patients, but rarely in the healthy individuals (Lavelle and Sokol, 2020). It can exacerbate colitis by disrupting the epithelial barrier and inducing aberrant inflammation (Liu et al., 2020). Meanwhile, oral dysbiosis in patients with periodontitis can directly modulate the pathogenesis of IBD through the recruitment of the oral-gut axis (She et al., 2020). Specifically, P. gingivalis, a key pathogen of periodontitis, destroyed the gut barrier function by inducing the depression of tight junction proteins and causing dramatic alterations in the gut microbiome, including the enrichment of the Clostridiaceae family, thus promoting the gut and systemic inflammation (Kato et al., 2018; Kobayashi et al., 2020).
The imbalance of intestinal microecology due to H. pylori infection may also be associated with a series of other systemic diseases. Numerous retrospective cohort studies indicated that there was a correlation between positive H. pylori serological test, gut flora disturbance, and the incidence of Alzheimer’s disease (Baj et al., 2021). The gut microbiota creates a natural protective barrier, and secrets numerous neurotransmitters and neuromodulators, such as serotonin, γ-aminobutyric acid, dopamine, or SCFA including acetate, propionate, and butyrate to defend against microorganisms and endotoxin translocation (Paone and Cani, 2020), however, the H. pylori infection could remodel the gut microbiota, which may affect the function of the nervous system by inducing the degeneration and loss of neurons (Baj et al., 2021). Other systematic diseases related to gut microbiota, such as inflammation, dyslipidemia, hyperglycemia, arteriosclerosis, and hypertension could also be affected by H. pylori infection through its impacts on the changes in gut microbiota (Beydoun et al., 2018). However, its specific mechanism of action remains to be explored.
Probiotics appear to be an effective way to prevent and treat related diseases by regulating the balance of microbiota. Probiotics are defined as living microbial species, including bacteria and yeast, with the capabilities of regulating the host immune functions or by preserving the balance of intestinal flora, promoting nutrient absorption and maintaining intestinal health (Chee et al., 2020; Da Silva et al., 2021). They can be used to treat gastrointestinal disorders, sometimes in combination with other drugs (Domingo, 2017), to improve the balance of gut microbiota, enhance the production of short-chain fatty acids, and interact with host cells such as immune, nerve, and endocrine cells in the gastrointestinal tract (Hori et al., 2020). Therefore, for H. pylori-infected patients, probiotics may be an effective alternative to the antibiotic eradication therapy of H. pylori. The mechanisms of probiotics acting on H. pylori are mainly due to the following ways: 1. Probiotics secreted various antibacterial substances such as lactic acid, acetic acid, and hydrogen peroxide to inhibit the growth of H. pylori (Kim et al., 2003); 2. Probiotics can adhere to the receptor through non-specific competition to inhibit the adhesion of H. pylori on the gastric epithelial cells (Johnson-Henry et al., 2004); 3. Probiotics can restore the secretion of gastric mucus, which was significantly reduced in the patients’ gastritis because of the gastric epithelium damage or proliferation (Mack et al., 2003); 4. Probiotics can interact with epithelial cells and promote the secretion of anti-inflammatory cytokines to reduce the host’s immune response (Gill, 2003). Therefore, probiotics can be used as new agents to regulate H. pylori infection and its effects on gut microbiome by providing effective prevention and control of gastrointestinal diseases.
Oral–gut microbiota in gastric disease and cancer
The pathogenicity of H. pylori mainly depends on its flagella, helical structure, lipopolysaccharide, cytotoxin-related protein, vacuolar toxin, and other pathogenic factors (Yamaoka, 2010), and its infection is closely related to chronic gastritis, peptic ulcer, gastric mucosa-associated lymphoid tissue lymphoma, gastric cancer, and other diseases. H. pylori also showed synergistic effect on the oral-gut axis commensal microbiota (Wong et al., 2019). Bacteria in the oral cavity and gut may be associated with the gastric cancer and be served as a diagnostic biomarker for gastric cancer (Park et al., 2021). For example, oropharyngeal or intestinal commensals such as Streptococcus, Bifidobacterium, Lactobacillus, Veilonella, Klebsiella, Escherichia, Pseudomonas, Neisseria, Staphylococcus, and Bacillus were all related to the development of gastric cancer as patients with gastric cancer had higher bacterial counts of these species than patients with other gastric diseases (Chen et al., 2021a).
The oral and gut are anatomically belonged to the digestive tract and are well-linked physically and chemically (Park et al., 2021). Under healthy conditions, the microbiota of these two habitats is separated, but under pathological conditions, they may enhance their communications (Seedorf et al., 2014). Common oral flora, such as Porphyromonas, Fusobacterium, Pseudomonas, Haemophilus, and Veillonella, can be detected in the gut of the elderly and patients with low gastric acid (Odamaki et al., 2016; Iwauchi et al., 2019). The stomach is anatomically located between the oral cavity and the gut. The gastric diseases are also significantly related to the microbiome of the oral-gut axis (Olsen and Yamazaki, 2019). In the oral cavity of chronic gastritis caused by H. pylori infection, low levels of F. nucleatum and P. gingivalis were detected (Contaldo et al., 2021). In the oral cavity of gastric cancer patients, pro-inflammatory taxa such as Corynebacterium and Streptococcus were enriched, but Haemophilus, Neisseria, Parvimonas, Peptostreptococcus, Porphyromonas, and Prevotella were reduced, indicating that the salivary microbiota was involved in gastric cancer pathogenesis by inducing the accumulation of pro-inflammatory bacteria and the reduction of carcinogenic N-nitroso compounds as Haemophilus and Neisseria can reduce the nitrite (Huang et al., 2021). Antibiotics and proton pump inhibitors which are used to treat H. pylori infection can reduce the diversity of microorganisms in the stomach and reduce gastric acid secretion to increase the translocation of microorganisms from the oral-gut axis, such as more oral predominant flora can be found in the stomach (Khor et al., 2021). However, the specific mechanisms remain to be further explored.
Conclusion
H. pylori infection has a great impact on the microbiome of the oral-gut axis and has played important roles in the maintenance of host health and the development of oral, gut, and systematic diseases (The Integrative HMP (iHmp) Research Network Consortium, 2019; Park et al., 2021). However, their interactions are dynamic due to the possible reasons: 1. the growth conditions are different between in vivo and in vitro experiments; 2. the body’s immune defense and microbial composition are diverse under different host health conditions; 3. Different strains from the same genus or species may show different pathogenicity. Therefore, the interaction between H. pylori and oral-gut axis microbiota under different conditions is important for the prediction, prevention, and treatment of diseases, but the evaluations of detailed mechanisms are still needed.
Author contributions
XC: Investigation, Writing – Original Draft Preparation, Validation. NW: Investigation, Writing – Original Draft Preparation. JW: Investigation. BL: Investigation. LC: Conceptualization, Funding Acquisition, Project Administration, Supervision. BR: Conceptualization, Funding Acquisition, Project Administration, Supervision, Writing – Review & Editing. All authors contributed to the article and approved the submitted version.
Funding
This research received funding from the National Natural Science Foundation of China, Grant numbers: 81870778, 81600858, 81870759, 81870754, Applied Basic Research Programs of Sichuan Province, Grant number 2020YJ0227, Sichuan Province Key R&D Program, Grant number 2021YFQ0064. The funders had no role in the study design, data collection, and analysis, decision to publish, or preparation of the manuscript.
Conflict of interest
The authors declare that the research was conducted in the absence of any commercial or financial relationships that could be construed as a potential conflict of interest.
Publisher’s note
All claims expressed in this article are solely those of the authors and do not necessarily represent those of their affiliated organizations, or those of the publisher, the editors and the reviewers. Any product that may be evaluated in this article, or claim that may be made by its manufacturer, is not guaranteed or endorsed by the publisher.
References
Acharya, C., Sahingur, S. E., Bajaj, J. S. (2017). Microbiota, cirrhosis, and the emerging oral-gut-liver axis. JCI Insight 2 (19), e94416. doi: 10.1172/jci.insight.94416
Albuquerque-Souza, E., Sahingur, S. E. (2022). Periodontitis, chronic liver diseases, and the emerging oral-gut-liver axis. Periodontol 89 (1), 125–141. 2000. doi: 10.1111/prd.12427
Anand, P. S., Nandakumar, K., Shenoy, K. (2006). Are dental plaque, poor oral hygiene, and periodontal disease associated with helicobacter pylori infection? J. periodontol 77, 692–698. doi: 10.1902/jop.2006.050163
Anbari, F., Moghaddam, A. A., Sabeti, E., Khodabakhshi, A. (2019). Halitosis: Helicobacter pylori or oral factors. Helicobacter 24, e12556. doi: 10.1111/hel.12556
Baj, J., Forma, A., Flieger, W., Morawska, I., Michalski, A., Buszewicz, G., et al. (2021). Helicobacter pylori infection and extragastric diseases-a focus on the central nervous system. Cells 10 (9), 2191. doi: 10.3390/cells10092191
Baquero, F., Coque, T. M., Galán, J. C., Martinez, J. L. (2021). The origin of niches and species in the bacterial world. Front. Microbiol. 12, 657986. doi: 10.3389/fmicb.2021.657986
Baruch, E. N., Youngster, I., Ben-Betzalel, G., Ortenberg, R., Lahat, A., Katz, L., et al. (2021). Fecal microbiota transplant promotes response in immunotherapy-refractory melanoma patients. Science 371, 602–609. doi: 10.1126/science.abb5920
Benavides-Ward, A., Vasquez-Achaya, F., Silva-Caso, W., Aguilar-Luis, M. A., Mazulis, F., Urteaga, N., et al. (2018). Helicobacter pylori and its relationship with variations of gut microbiota in asymptomatic children between 6 and 12 years. BMC Res. Notes 11, 468. doi: 10.1186/s13104-018-3565-5
Beydoun, M. A., Beydoun, H. A., Elbejjani, M., Dore, G. A., Zonderman, A. B. (2018). Helicobacter pylori seropositivity and its association with incident all-cause and alzheimer's disease dementia in large national surveys. Alzheimers Dement 14, 1148–1158. doi: 10.1016/j.jalz.2018.04.009
Camilo, V., Sugiyama, T., Touati, E. (2017). Pathogenesis of helicobacter pylori infection. Helicobacter 22 (Suppl 1) e12405. doi: 10.1111/hel.12405
Caselli, E., Fabbri, C., D'Accolti, M., Soffritti, I., Bassi, C., Mazzacane, S., et al. (2020). Defining the oral microbiome by whole-genome sequencing and resistome analysis: the complexity of the healthy picture. BMC Microbiol. 20, 120. doi: 10.1186/s12866-020-01801-y
Chee, W. J. Y., Chew, S. Y., Than, L. T. L. (2020). Vaginal microbiota and the potential of lactobacillus derivatives in maintaining vaginal health. Microbial Cell factories 19, 1–24. doi: 10.1186/s12934-020-01464-4
Chen, C. C., Liou, J. M., Lee, Y. C., Hong, T. C., El-Omar, E. M., Wu, M. S. (2021a). The interplay between helicobacter pylori and gastrointestinal microbiota. Gut Microbes 13, 1–22. doi: 10.1080/19490976.2021.1909459
Chen, L., Xu, W., Lee, A., He, J., Huang, B., Zheng, W., et al. (2018). The impact of helicobacter pylori infection, eradication therapy and probiotic supplementation on gut microenvironment homeostasis: An open-label, randomized clinical trial. EBioMedicine 35, 87–96. doi: 10.1016/j.ebiom.2018.08.028
Chen, X., Zhou, X., Liao, B., Zhou, Y., Cheng, L., Ren, B. (2021b). The cross-kingdom interaction between helicobacter pylori and candida albicans. PloS Pathog. 17, e1009515. doi: 10.1371/journal.ppat.1009515
Chua, E. G., Chong, J. Y., Lamichhane, B., Webberley, K. M., Marshall, B. J., Wise, M. J., et al. (2019). Gastric helicobacter pylori infection perturbs human oral microbiota. PeerJ 7, e6336. doi: 10.7717/peerj.6336
Contaldo, M., Fusco, A., Stiuso, P., Lama, S., Gravina, A. G., Itro, A., et al. (2021). Oral microbiota and salivary levels of oral pathogens in gastro-intestinal diseases: Current knowledge and exploratory study. Microorganisms 9 (5), 1064. doi: 10.3390/microorganisms9051064
D'Enfert, C., Kaune, A. K., Alaban, L. R., Chakraborty, S., Cole, N., Delavy, M., et al. (2020). The impact of the fungus-Host-Microbiota interplay upon candida albicans infections: current knowledge and new perspectives. FEMS Microbiol. Rev.45 (3), fuaa060. doi: 10.1093/femsre/fuaa060
Dash, N. R., Khoder, G., Nada, A. M., Al Bataineh, M. T. (2019). Exploring the impact of helicobacter pylori on gut microbiome composition. PloS One 14, e0218274. doi: 10.1371/journal.pone.0218274
Da Silva, T. F., Casarotti, S. N., De Oliveira G. L. V. & Penna, A. L. B. (2021). The impact of probiotics, prebiotics, and synbiotics on the biochemical, clinical, and immunological markers, as well as on the gut microbiota of obese hosts. Crit. Rev. Food Sci. Nutr. 61, 337–355. doi: 10.1080/10408398.2020.1733483
Davar, D., Dzutsev, A. K., Mcculloch, J. A., Rodrigues, R. R., Chauvin, J. M., Morrison, R. M., et al. (2021). Fecal microbiota transplant overcomes resistance to anti-PD-1 therapy in melanoma patients. Science 371, 595–602. doi: 10.1126/science.abf3363
Domingo, J. J. S. (2017). Review of the role of probiotics in gastrointestinal diseases in adults. Gastroenterología y Hepatolía 40, 417–429. doi: 10.1016/j.gastrohep.2016.12.003
Du Teil Espina, M., Gabarrini, G., Harmsen, H. J. M., Westra, J., Van Winkelhoff, A. J., Van Dijl, J. M. (2019). Talk to your gut: the oral-gut microbiome axis and its immunomodulatory role in the etiology of rheumatoid arthritis. FEMS Microbiol. Rev. 43, 1–18. doi: 10.1093/femsre/fuy035
Freitas, D., Le Feunteun, S., Panouillé, M., Souchon, I. (2018). The important role of salivary α-amylase in the gastric digestion of wheat bread starch. Food Funct. 9, 200–208. doi: 10.1039/C7FO01484H
Frost, F., Kacprowski, T., Rühlemann, M., Bang, C., Franke, A., Zimmermann, K., et al. (2019). Helicobacter pylori infection associates with fecal microbiota composition and diversity. Sci. Rep. 9, 1–10. doi: 10.1038/s41598-019-56631-4
Gao, J. J., Zhang, Y., Gerhard, M., Mejias-Luque, R., Zhang, L., Vieth, M., et al. (2018). Association between gut microbiota and helicobacter pylori-related gastric lesions in a high-risk population of gastric cancer. Front. Cell Infect. Microbiol. 8, 202. doi: 10.3389/fcimb.2018.00202
Ge, Z., Sheh, A., Feng, Y., Muthupalani, S., Ge, L., Wang, C., et al. (2018). Helicobacter pylori-infected C57BL/6 mice with different gastrointestinal microbiota have contrasting gastric pathology, microbial and host immune responses. Sci. Rep. 8, 8014. doi: 10.1038/s41598-018-25927-2
Ghosh, S., Pramanik, S. (2021). Structural diversity, functional aspects and future therapeutic applications of human gut microbiome. Arch. Microbiol. 203, 5281–5308. doi: 10.1007/s00203-021-02516-y
Gill, H. S. (2003). Probiotics to enhance anti-infective defences in the gastrointestinal tract. Best Pract. Res. Clin. Gastroenterol. 17, 755–773. doi: 10.1016/s1521-6918(03)00074-x
Guo, Y., Zhang, Y., Gerhard, M., Gao, J. J., Mejias-Luque, R., Zhang, L., et al. (2020). Effect of helicobacter pylori on gastrointestinal microbiota: a population-based study in linqu, a high-risk area of gastric cancer. Gut 69, 1598–1607. doi: 10.1136/gutjnl-2019-319696
Hajishengallis, G. (2022). Interconnection of periodontal disease and comorbidities: Evidence, mechanisms, and implications. Periodontol 2000 89 (1), 9–18. doi: 10.1111/prd.12430
Hakansson, A. P., Orihuela, C. J., Bogaert, D. (2018). Bacterial-host interactions: Physiology and pathophysiology of respiratory infection. Physiol. Rev. 98, 781–811. doi: 10.1152/physrev.00040.2016
He, C., Peng, C., Wang, H., Ouyang, Y., Zhu, Z., Shu, X., et al. (2019). The eradication of helicobacter pylori restores rather than disturbs the gastrointestinal microbiota in asymptomatic young adults. Helicobacter 24, e12590. doi: 10.1111/hel.12590
He, C., Yang, Z., Lu, N. (2016). Imbalance of gastrointestinal microbiota in the pathogenesis of helicobacter pylori-associated diseases. Helicobacter 21, 337–348. doi: 10.1111/hel.12297
Hold, G. L., Hansen, R. (2019). Impact of the gastrointestinal microbiome in health and disease: Co-evolution with the host immune system. Curr. Top. Microbiol. Immunol. 421, 303–318. doi: 10.1007/978-3-030-15138-6_12
Hollister, E. B., Riehle, K., Luna, R. A., Weidler, E. M., Rubio-Gonzales, M., Mistretta, T. A., et al. (2015). Structure and function of the healthy pre-adolescent pediatric gut microbiome. Microbiome 3, 36. doi: 10.1186/s40168-015-0101-x
Hori, T., Matsuda, K., Oishi, K. (2020). Probiotics: A dietary factor to modulate the gut microbiome, host immune system, and gut-brain interaction. Microorganisms 8 (9), 1401. doi: 10.3390/microorganisms8091401
Hou, J. J., Wang, X., Wang, Y. M., Wang, B. M. (2021). Interplay between gut microbiota and bile acids in diarrhoea-predominant irritable bowel syndrome: a review. Crit. Rev. Microbiol. 1–18. doi: 10.1080/1040841X.2021.2018401
Huang, K., Gao, X., Wu, L., Yan, B., Wang, Z., Zhang, X., et al. (2021). Salivary microbiota for gastric cancer prediction: An exploratory study. Front. Cell Infect. Microbiol. 11, 640309. doi: 10.3389/fcimb.2021.640309
Hu, Z., Zhang, Y., Li, Z., Yu, Y., Kang, W., Han, Y., et al. (2016). Effect of helicobacter pylori infection on chronic periodontitis by the change of microecology and inflammation. Oncotarget 7, 66700–66712. doi: 10.18632/oncotarget.11449
Iino, C., Shimoyama, T., Chinda, D., Arai, T., Chiba, D., Nakaji, S., et al. (2018). Infection of helicobacter pylori and atrophic gastritis influence lactobacillus in gut microbiota in a Japanese population. Front. Immunol. 9, 712. doi: 10.3389/fimmu.2018.00712
Ishihara, K., Miura, T., Kimizuka, R., Ebihara, Y., Mizuno, Y., Okuda, K. (1997). Oral bacteria inhibit helicobacter pylori growth. FEMS Microbiol. Lett. 152, 355–361. doi: 10.1111/j.1574-6968.1997.tb10452.x
Iwauchi, M., Horigome, A., Ishikawa, K., Mikuni, A., Nakano, M., Xiao, J. Z., et al. (2019). Relationship between oral and gut microbiota in elderly people. Immun. Inflammation Dis. 7, 229–236. doi: 10.1002/iid3.266
Ji, Y., Liang, X., Lu, H. (2020). Analysis of by high-throughput sequencing: Helicobacter pylori infection and salivary microbiome. BMC Oral. Health 20, 84. doi: 10.1186/s12903-020-01070-1
Ji, J., Zhang, Z., Gao, H., Zhou, W., Li, Y., Shi, X. (2022). Modulation of oral microflora during helicobacter pylori eradication. J. Coll. Physicians Surg. Pak 32, 308–312. doi: 10.29271/jcpsp.2022.03.308
Johnson-Henry, K. C., Mitchell, D. J., Avitzur, Y., Galindo-Mata, E., Jones, N. L., Sherman, P. M. (2004). Probiotics reduce bacterial colonization and gastric inflammation in h. pylori-infect mice. Dig Dis. Sci. 49, 1095–1102. doi: 10.1023/b:ddas.0000037794.02040.c2
Jones, T. A., Hernandez, D. Z., Wong, Z. C., Wandler, A. M., Guillemin, K. (2017). The bacterial virulence factor CagA induces microbial dysbiosis that contributes to excessive epithelial cell proliferation in the drosophila gut. PloS Pathog. 13, e1006631. doi: 10.1371/journal.ppat.1006631
Kadota, T., Hamada, M., Nomura, R., Ogaya, Y., Okawa, R., Uzawa, N., et al. (2020). Distribution of helicobacter pylori and periodontopathic bacterial species in the oral cavity. Biomedicines 8 (6), 161. doi: 10.3390/biomedicines8060161
Kang, J., Blaser, M. J. (2006). Bacterial populations as perfect gases: genomic integrity and diversification tensions in helicobacter pylori. Nat. Rev. Microbiol. 4, 826–836. doi: 10.1038/nrmicro1528
Kato, T., Yamazaki, K., Nakajima, M., Date, Y., Kikuchi, J., Hase, K., et al. (2018). Oral administration of porphyromonas gingivalis alters the gut microbiome and serum metabolome. mSphere 3 (5), e00460-18. doi: 10.1128/mSphere.00460-18
Khor, B., Snow, M., Herrman, E., Ray, N., Mansukhani, K., Patel, K. A., et al. (2021). Interconnections between the oral and gut microbiomes: Reversal of microbial dysbiosis and the balance between systemic health and disease. Microorganisms 9 (3), 496. doi: 10.3390/microorganisms9030496
Kienesberger, S., Cox, L. M., Livanos, A., Zhang, X. S., Chung, J., Perez-Perez, G. I., et al. (2016). Gastric helicobacter pylori infection affects local and distant microbial populations and host responses. Cell Rep. 14, 1395–1407. doi: 10.1016/j.celrep.2016.01.017
Kim, T. S., Hur, J. W., Yu, M. A., Cheigh, C. I., Kim, K. N., Hwang, J. K., et al. (2003). Antagonism of helicobacter pylori by bacteriocins of lactic acid bacteria. J. Food Prot 66, 3–12. doi: 10.4315/0362-028X-66.1.3
Kivi, M., Tindberg, Y. (2006). Helicobacter pylori occurrence and transmission: a family affair? Scand J. Infect. Dis. 38, 407–417. doi: 10.1080/00365540600585131
Kobayashi, R., Ogawa, Y., Hashizume-Takizawa, T., Kurita-Ochiai, T. (2020). Oral bacteria affect the gut microbiome and intestinal immunity. Pathog. Dis. 78 (3), ftaa024. doi: 10.1093/femspd/ftaa024
Lamont, R. J., Koo, H., Hajishengallis, G. (2018). The oral microbiota: dynamic communities and host interactions. Nat. Rev. Microbiol. 16, 745–759. doi: 10.1038/s41579-018-0089-x
Lavelle, A., Sokol, H. (2020). Gut microbiota-derived metabolites as key actors in inflammatory bowel disease. Nat. Rev. Gastroenterol. Hepatol. 17, 223–237. doi: 10.1038/s41575-019-0258-z
Lee, M., Chang, E. B. (2021). Inflammatory bowel diseases (IBD) and the microbiome-searching the crime scene for clues. Gastroenterology 160, 524–537. doi: 10.1053/j.gastro.2020.09.056
Lee, Y. C., Dore, M. P., Graham, D. Y. (2022). Diagnosis and treatment of helicobacter pylori infection. Annu. Rev. Med. 73, 183–195. doi: 10.1146/annurev-med-042220-020814
Liu, H., Hong, X. L., Sun, T. T., Huang, X. W., Wang, J. L., Xiong, H. (2020). Fusobacterium nucleatum exacerbates colitis by damaging epithelial barriers and inducing aberrant inflammation. J. Dig Dis. 21, 385–398. doi: 10.1111/1751-2980.12909
Li, S., Zhang, Y., Yang, Z., Li, J., Li, Y., LI, H., et al. (2021). Helicobacter pylori infection is correlated with the incidence of erosive oral lichen planus and the alteration of the oral microbiome composition. BMC Microbiol. 21, 122. doi: 10.1186/s12866-021-02188-0
Mack, D. R., Ahrne, S., Hyde, L., Wei, S., Hollingsworth, M. A. (2003). Extracellular MUC3 mucin secretion follows adherence of lactobacillus strains to intestinal epithelial cells in vitro. Gut 52, 827–833. doi: 10.1136/gut.52.6.827
Mark Welch, J. L., Dewhirst, F. E., Borisy, G. G. (2019). Biogeography of the oral microbiome: The site-specialist hypothesis. Annu. Rev. Microbiol. 73, 335–358. doi: 10.1146/annurev-micro-090817-062503
Martín-Núñez, G. M., Cornejo-Pareja, I., Coin-Aragüez, L., Roca-Rodríguez, M. D. M., Muñoz-Garach, A., Clemente-Postigo, M., et al. (2019). H. pylori eradication with antibiotic treatment causes changes in glucose homeostasis related to modifications in the gut microbiota. PloS One 14, e0213548. doi: 10.1371/journal.pone.0213548
Martin, M. E., Solnick, J. V. (2014). The gastric microbial community, helicobacter pylori colonization, and disease. Gut Microbes 5, 345–350. doi: 10.4161/gmic.28573
Matsuura, E., Kobayashi, K., Lopez, L. R. (2008). Preventing autoimmune and infection triggered atherosclerosis for an enduring healthful lifestyle. Autoimmun Rev. 7, 214–222. doi: 10.1016/j.autrev.2007.11.008
Mégraud, F. (1995). Transmission of helicobacter pylori: faecal-oral versus oral-oral route. Aliment Pharmacol. Ther. 9 Suppl 2, 85–91. doi: 10.1111/j.1365-2036.1995.tb00357.x
Miller, D. P., Scott, D. A. (2021). Inherently and conditionally essential protein catabolism genes of porphyromonas gingivalis. Trends Microbiol. 29, 54–64. doi: 10.1016/j.tim.2020.09.002
Mladenova, I., Durazzo, M. (2018). Transmission of helicobacter pylori. Minerva Gastroenterol e dietologica 64, 251–254. doi: 10.23736/S1121-421X.18.02480-7
Mohammadi, S. O., Yadegar, A., Kargar, M., Mirjalali, H., Kafilzadeh, F. (2020). The impact of helicobacter pylori infection on gut microbiota-endocrine system axis; modulation of metabolic hormone levels and energy homeostasis. J. Diabetes Metab. Disord. 19, 1855–1861. doi: 10.1007/s40200-020-00608-y
Nobbs, A. H., Jenkinson, H. F. (2015). Interkingdom networking within the oral microbiome. Microbes infection 17, 484–492. doi: 10.1016/j.micinf.2015.03.008
Nomura, R., Kadota, T., Ogaya, Y., Matayoshi, S., Iwashita, N., Okawa, R., et al. (2020). Contribution of streptococcus mutans to helicobacter pylori colonisation in oral cavity and gastric tissue. Sci. Rep. 10, 12540. doi: 10.1038/s41598-020-69368-2
Noto, J. M., Zackular, J. P., Varga, M. G., Delgado, A., Romero-Gallo, J., Scholz, M. B., et al. (2019). Modification of the gastric mucosal microbiota by a strain-specific helicobacter pylori oncoprotein and carcinogenic histologic phenotype. mBio 10 (3), e00955-19. doi: 10.1128/mBio.00955-19
Odamaki, T., Kato, K., Sugahara, H., Hashikura, N., Takahashi, S., Xiao, J. Z., et al. (2016). Age-related changes in gut microbiota composition from newborn to centenarian: a cross-sectional study. BMC Microbiol. 16, 90. doi: 10.1186/s12866-016-0708-5
Okuda, K., Kimizuka, R., Katakura, A., Nakagawa, T., Ishihara, K. (2003). Ecological and immunopathological implications of oral bacteria in helicobacter pylori-infected disease. J. periodontol 74, 123–128. doi: 10.1902/jop.2003.74.1.123
Olsen, I., Yamazaki, K. (2019). Can oral bacteria affect the microbiome of the gut? J. Oral. Microbiol. 11, 1586422. doi: 10.1080/20002297.2019.1586422
Palencia, S. L., García, A., Palencia, M. (2022). Multiple surface interaction mechanisms direct the anchoring, co-aggregation and formation of dual-species biofilm between candida albicans and helicobacter pylori. J. Advanced Res. 35, 169–185. doi: 10.1016/j.jare.2021.03.013
Paone, P., Cani, P. D. (2020). Mucus barrier, mucins and gut microbiota: the expected slimy partners? Gut 69, 2232–2243. doi: 10.1136/gutjnl-2020-322260
Park, S. Y., Hwang, B. O., Lim, M., Ok, S. H., Lee, S. K., Chun, K. S., et al. (2021). Oral-gut microbiome axis in gastrointestinal disease and cancer. Cancers (Basel) 13 (9), 2124. doi: 10.3390/cancers13092124.
Park, J., Shokeen, B., Haake, S. K., Lux, R. (2016). Characterization of fusobacterium nucleatum ATCC 23726 adhesins involved in strain-specific attachment to porphyromonas gingivalis. Int. J. Oral. Sci. 8, 138–144. doi: 10.1038/ijos.2016.27
Perez-Perez, G. I., Rothenbacher, D., Brenner, H. (2004). Epidemiology of helicobacter pylori infection. Helicobacter 9, 1–6. doi: 10.1111/j.1083-4389.2004.00248.x
Qin, J., Li, R., Raes, J., Arumugam, M., Burgdorf, K. S., Manichanh, C., et al. (2010). A human gut microbial gene catalogue established by metagenomic sequencing. nature 464, 59–65. doi: 10.1038/nature08821
Ren, Q., Yan, X., Zhou, Y., Li, W. X. (2016). Periodontal therapy as adjunctive treatment for gastric helicobacter pylori infection. Cochrane Database Syst. Rev. 2, Cd009477. doi: 10.1002/14651858.CD009477.pub2
Rimbara, E., Fischbach, L. A., Graham, D. Y. (2011). Optimal therapy for helicobacter pylori infections. Nat. Rev. Gastroenterol. Hepatol. 8, 79–88. doi: 10.1038/nrgastro.2010.210
Rizzo, M., Cappello, F., Marfil, R., Nibali, L., Marino Gammazza, A., Rappa, F., et al. (2012). Heat-shock protein 60 kDa and atherogenic dyslipidemia in patients with untreated mild periodontitis: a pilot study. Cell Stress Chaperones 17, 399–407. doi: 10.1007/s12192-011-0315-1
Saniee, P., Siavoshi, F., Nikbakht Broujeni, G., Khormali, M., Sarrafnejad, A., Malekzadeh, R. (2013). Localization of h.pylori within the vacuole of candida yeast by direct immunofluorescence technique. Arch. Iranian Med. 16, 705–710. doi: 0131612/aim.005
Schulz, C., Schütte, K., Koch, N., Vilchez-Vargas, R., Wos-Oxley, M. L., Oxley, A. P. A., et al. (2018). The active bacterial assemblages of the upper GI tract in individuals with and without helicobacter infection. Gut 67, 216–225. doi: 10.1136/gutjnl-2016-312904
Seedorf, H., Griffin, N. W., Ridaura, V. K., Reyes, A., Cheng, J., Rey, F. E., et al. (2014). Bacteria from diverse habitats colonize and compete in the mouse gut. Cell 159, 253–266. doi: 10.1016/j.cell.2014.09.008
Sekirov, I., Russell, S. L., Antunes, L. C., Finlay, B. B. (2010). Gut microbiota in health and disease. Physiol. Rev. 90, 859–904. doi: 10.1152/physrev.00045.2009
Shanahan, F., Ghosh, T. S., O’Toole, P. W. (2021). The healthy microbiome–what is the definition of a healthy gut microbiome? Gastroenterology 160, 483–494. doi: 10.1053/j.gastro.2020.09.057
Sharma, N., Bhatia, S., Sodhi, A. S., Batra, N. (2018b). Oral microbiome and health. AIMS Microbiol. 4, 42–66. doi: 10.3934/microbiol.2018.1.42
Sharma, D., Malik, A., Guy, C. S., Karki, R., Vogel, P., Kanneganti, T. D. (2018a). Pyrin inflammasome regulates tight junction integrity to restrict colitis and tumorigenesis. Gastroenterology 154, 948–964.e8. doi: 10.1053/j.gastro.2017.11.276
She, Y. Y., Kong, X. B., Ge, Y. P., Liu, Z. Y., Chen, J. Y., Jiang, J. W., et al. (2020). Periodontitis and inflammatory bowel disease: a meta-analysis. BMC Oral. Health 20, 67. doi: 10.1186/s12903-020-1053-5
Sitkin, S., Lazebnik, L., Avalueva, E., Kononova, S., Vakhitov, T. (2022). Gastrointestinal microbiome and helicobacter pylori: Eradicate, leave it as it is, or take a personalized benefit-risk approach? World J. Gastroenterol. 28, 766–774. doi: 10.3748/wjg.v28.i7.766
Smith, P. M., Howitt, M. R., Panikov, N., Michaud, M., Gallini, C. A., Bohlooly, Y. M., et al. (2013). The microbial metabolites, short-chain fatty acids, regulate colonic treg cell homeostasis. Science 341, 569–573. doi: 10.1126/science.1241165
Spor, A., Koren, O., Ley, R. (2011). Unravelling the effects of the environment and host genotype on the gut microbiome. Nat. Rev. Microbiol. 9, 279–290. doi: 10.1038/nrmicro2540
Sung, J. J. Y., Coker, O. O., Chu, E., Szeto, C. H., Luk, S. T. Y., Lau, H. C. H., et al. (2020). Gastric microbes associated with gastric inflammation, atrophy and intestinal metaplasia 1 year after helicobacter pylori eradication. Gut 69, 1572–1580. doi: 10.1136/gutjnl-2019-319826
Tao, Z. H., Han, J. X., Fang, J. Y. (2020). Helicobacter pylori infection and eradication: Exploring their impacts on the gastrointestinal microbiota. Helicobacter 25, e12754. doi: 10.1111/hel.12754
The Integrative HMP (iHmp) Research Network Consortium (2019). The integrative human microbiome project. Nature 569, 641–648. doi: 10.1038/s41586-019-1238-8
Tsay, F. W., Hsu, P. I. (2018). H. pylori infection and extra-gastroduodenal diseases. J. BioMed. Sci. 25, 65. doi: 10.1186/s12929-018-0469-6
Umeda, M., Kobayashi, H., Takeuchi, Y., Hayashi, J., Morotome-Hayashi, Y., Yano, K., et al. (2003). High prevalence of helicobacter pylori detected by PCR in the oral cavities of periodontitis patients. J. Periodontol 74, 129–134. doi: 10.1902/jop.2003.74.1.129
Vasapolli, R., Schütte, K., Schulz, C., Vital, M., Schomburg, D., Pieper, D. H., et al. (2019). Analysis of transcriptionally active bacteria throughout the gastrointestinal tract of healthy individuals. Gastroenterology 157, 1081–1092.e3. doi: 10.1053/j.gastro.2019.05.068
Wang, D., Li, Y., Zhong, H., Ding, Q., Lin, Y., Tang, S., et al. (2019). Alterations in the human gut microbiome associated with helicobacter pylori infection. FEBS Open Bio 9, 1552–1560. doi: 10.1002/2211-5463.12694
Wong, S. H., Kwong, T. N., Wu, C.-Y., Yu, J. (2019). Clinical applications of gut microbiota in cancer biology. Semin. Cancer Biol. 55, 28–36. doi: 10.1016/j.semcancer.2018.05.003
Wu, L., Wang, Z., Sun, G., Peng, L., Lu, Z., Yan, B., et al. (2019). Effects of anti-h. pylori triple therapy and a probiotic complex on intestinal microbiota in duodenal ulcer. Sci. Rep. 9, 12874. doi: 10.1038/s41598-019-49415-3
Wu, Z. F., Zou, K., Xiang, C. J., Jin, Z. J., Ding, H. H., Xu, S., et al. (2021). Helicobacter pylori infection is associated with the co-occurrence of bacteria in the oral cavity and the gastric mucosa. Helicobacter 26, e12786. doi: 10.1111/hel.12786
Yamaoka, Y. (2010). Mechanisms of disease: Helicobacter pylori virulence factors. Nat. Rev. Gastroenterol. Hepatol. 7, 629–641. doi: 10.1038/nrgastro.2010.154
Yu, Y., Yao, X., Liang, J., Lu, C., Yan, T., Lin, J. (2019). Is helicobacter pylori associated with behçet's syndrome? A meta-analysis. Helicobacter 24, e12663. doi: 10.1111/hel.12663
Zhang, W., Deng, X., Zhou, X., Hao, Y., Li, Y. (2018). Influence of helicobacter pylori culture supernatant on the ecological balance of a dual-species oral biofilm. J. Appl. Oral. Sci. 26, e20170113. doi: 10.1590/1678-7757-2017-0113
Zhao, Y., Gao, X., Guo, J., Yu, D., Xiao, Y., Wang, H., et al. (2019). Helicobacter pylori infection alters gastric and tongue coating microbial communities. Helicobacter 24, e12567. doi: 10.1111/hel.12567
Keywords: Helicobacter pylori, oral-gut axis, oral microbiota, gut microbiota, interactions between microorganisms
Citation: Chen X, Wang N, Wang J, Liao B, Cheng L and Ren B (2022) The interactions between oral-gut axis microbiota and Helicobacter pylori. Front. Cell. Infect. Microbiol. 12:914418. doi: 10.3389/fcimb.2022.914418
Received: 06 April 2022; Accepted: 14 July 2022;
Published: 03 August 2022.
Edited by:
Yi Hu, The First Affiliated Hospital of Nanchang University, ChinaReviewed by:
Cong He, The First Affiliated Hospital of Nanchang University, ChinaDurgesh Narain Singh, Institute of Science, Banaras Hindu University, India
Copyright © 2022 Chen, Wang, Wang, Liao, Cheng and Ren. This is an open-access article distributed under the terms of the Creative Commons Attribution License (CC BY). The use, distribution or reproduction in other forums is permitted, provided the original author(s) and the copyright owner(s) are credited and that the original publication in this journal is cited, in accordance with accepted academic practice. No use, distribution or reproduction is permitted which does not comply with these terms.
*Correspondence: Lei Cheng, chengleidentist@163.com; Biao Ren, renbiao@scu.edu.cn
†These authors have contributed equally to this work