Abnormal Serotonin Levels During Perinatal Development Lead to Behavioral Deficits in Adulthood
- 1Emotional Brain Institute, Nathan Kline Institute for Psychiatric Research, Orangeburg, NY, United States
- 2CNRS UMR 5292 – INSERM U1028, Lyon Neuroscience Research Center, Université Lyon 1, Lyon, France
- 3Department of Child and Adolescent Psychiatry, Hassenfeld Children’s Hospital at NYU Langone, New York, NY, United States
- 4Division of Clinical Research, Nathan Kline Institute for Psychiatric Research, Orangeburg, NY, United States
Serotonin (5-HT) is one of the best-studied modulatory neurotransmitters with ubiquitous presynaptic release and postsynaptic reception. 5-HT has been implicated in a wide variety of brain functions, ranging from autonomic regulation, sensory perception, feeding and motor function to emotional regulation and cognition. The role of this neuromodulator in neuropsychiatric diseases is unquestionable with important neuropsychiatric medications, e.g., most antidepressants, targeting this system. Importantly, 5-HT modulates neurodevelopment and changes in its levels during development can have life-long consequences. In this mini-review, we highlight that exposure to both low and high serotonin levels during the perinatal period can lead to behavioral deficits in adulthood. We focus on three exogenous factors that can change 5-HT levels during the critical perinatal period: dietary tryptophan depletion, exposure to serotonin-selective-reuptake-inhibitors (SSRIs) and poor early life care. We discuss the effects of each of these on behavioral deficits in adulthood.
Introduction
Serotonin (5-hydroxytryptamine, 5-HT) is a classic and well-studied neuromodulator. In addition to being implicated in various functions ranging from autonomic regulation to emotions in the adult, 5-HT also has a key role in neurodevelopment. Indeed, in the mammalian brain, 5-HT is one of first neurotransmitters to emerge. In rodents, serotonergic neurons appear on embryonic day (E) 12 (Lauder and Bloom, 1974), and start releasing 5-HT at E13 (Lidov and Molliver, 1982a,b; Lambe et al., 2000). Levels of 5-HT peak during the first postnatal week, declining thereafter to adult levels by postnatal day (P) 15 (Hohmann et al., 1988). The expression of 5-HT receptors starts between E12 and E17 with variations and shifts across the different subtypes and regions (Waeber et al., 1994; Beique et al., 2004; Bonnin et al., 2006; for review: Booij et al., 2015).
In humans, 5-HT neurons start to appear when the embryo is 5 weeks old and proliferate until gestational week 10 (Sundstrom et al., 1993; Levallois et al., 1997; Gingrich et al., 2017). Levels of 5-HT increase during the first 2 years of age and slowly decline to reach adult levels by age 5 (Sodhi and Sanders-Bush, 2004).
Importantly, 5-HT is involved in neural crest stem cell migration and proliferation (Vichier-Guerre et al., 2017). Notably, 5-HT can shape neuronal microcircuitry by acting on Reelin secretion, a protein involved in neuronal migration and positioning during development (Chameau et al., 2009). 5-HT is also critical in cell survival, growth and differentiation (Lavdas et al., 1997; Fricker et al., 2005; Khozhai and Otellin, 2012) as well as synaptogenesis (Khozhai and Otellin, 2012). For reviews see (Gaspar et al., 2003; Daubert and Condron, 2010).
Plasticity during the perinatal period is essential for the developing brain to adapt to a changing environment but opens a window where external factors can derail neuronal circuits and lead to maladaptive behaviors. This perinatal time window is a critical period when serotonergic activity can shape the development of neuronal circuitry and specifically emotional neurocircuitry (Lauder, 1990; Brummelte et al., 2017). In addition to genetic variants in key serotonergic genes [e.g., SERT, MAO; see (Murphy et al., 2008; Suri et al., 2014)], several exogenous factors change 5-HT levels during development: stress (Papaioannou et al., 2002), physical abuse (Raineki et al., 2015; Rincon-Cortes et al., 2015), food intake (Zhang et al., 2006; Serfaty et al., 2008; Zoratto et al., 2013), pharmaceutical and recreational drugs (Xu et al., 2004; Suri et al., 2014) as well as maternal inflammation (Goeden et al., 2016; St-Pierre et al., 2016) and maternal separation (Shannon et al., 2005). Importantly, manipulations that either increase or decrease 5-HT levels during development have been shown to lead to behavioral deficits in the adult (Table 1). Together, these studies suggest that optimal levels of 5-HT must be maintained during development and that any deviations from these optimal levels, in either direction, can lead to long-lasting behavioral deficits (Figure 1). In this mini-review, we focus on three distinct exogenous factors, diet, pharmacological exposure and early-life care, which affect serotonin levels during the perinatal period and have behavioral consequences in adulthood.
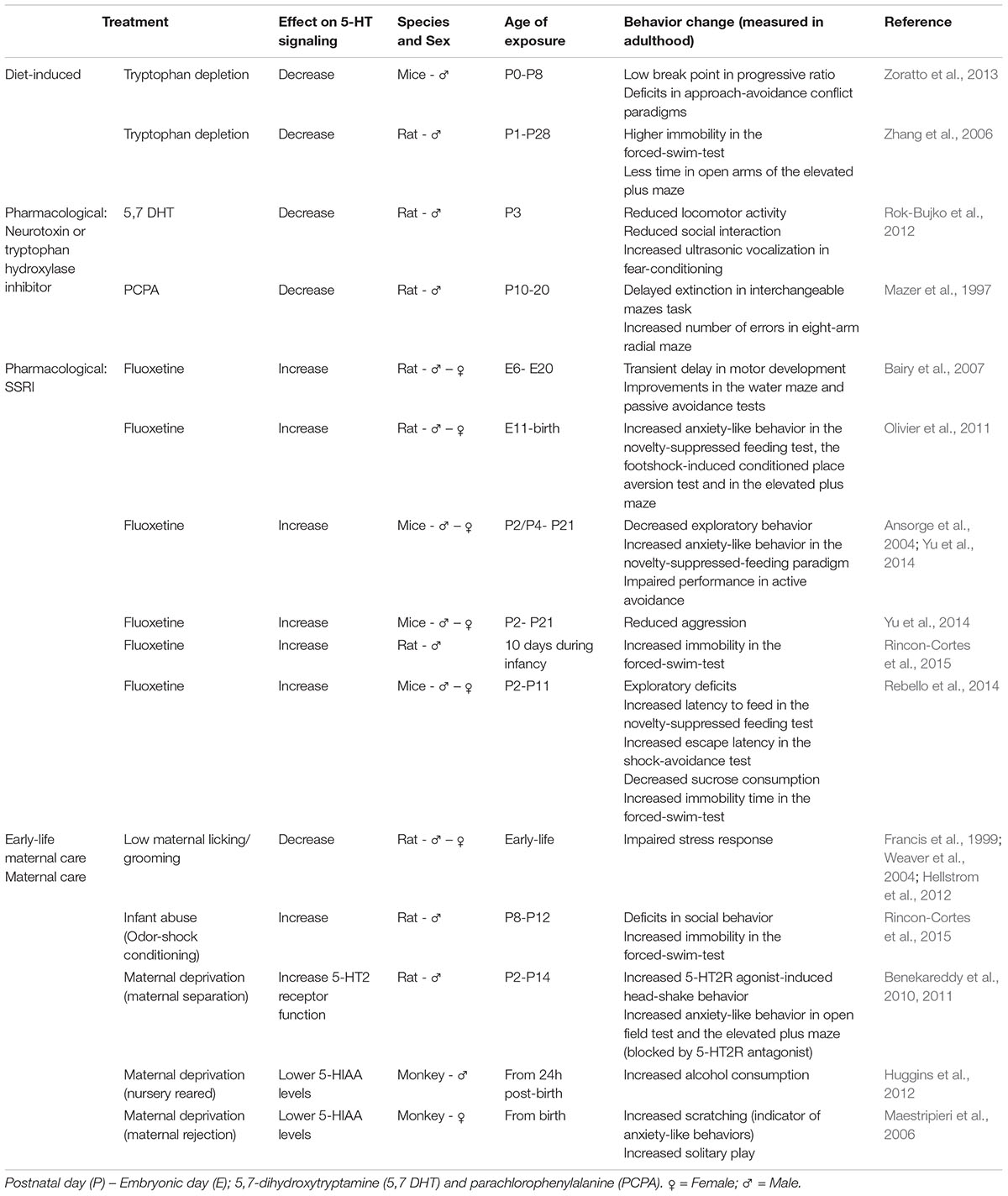
TABLE 1. Manipulations of the serotonergic system during development lead to behavioral deficits observed during adulthood: Examples of diet, pharmacological agents and early-life maternal care effects.
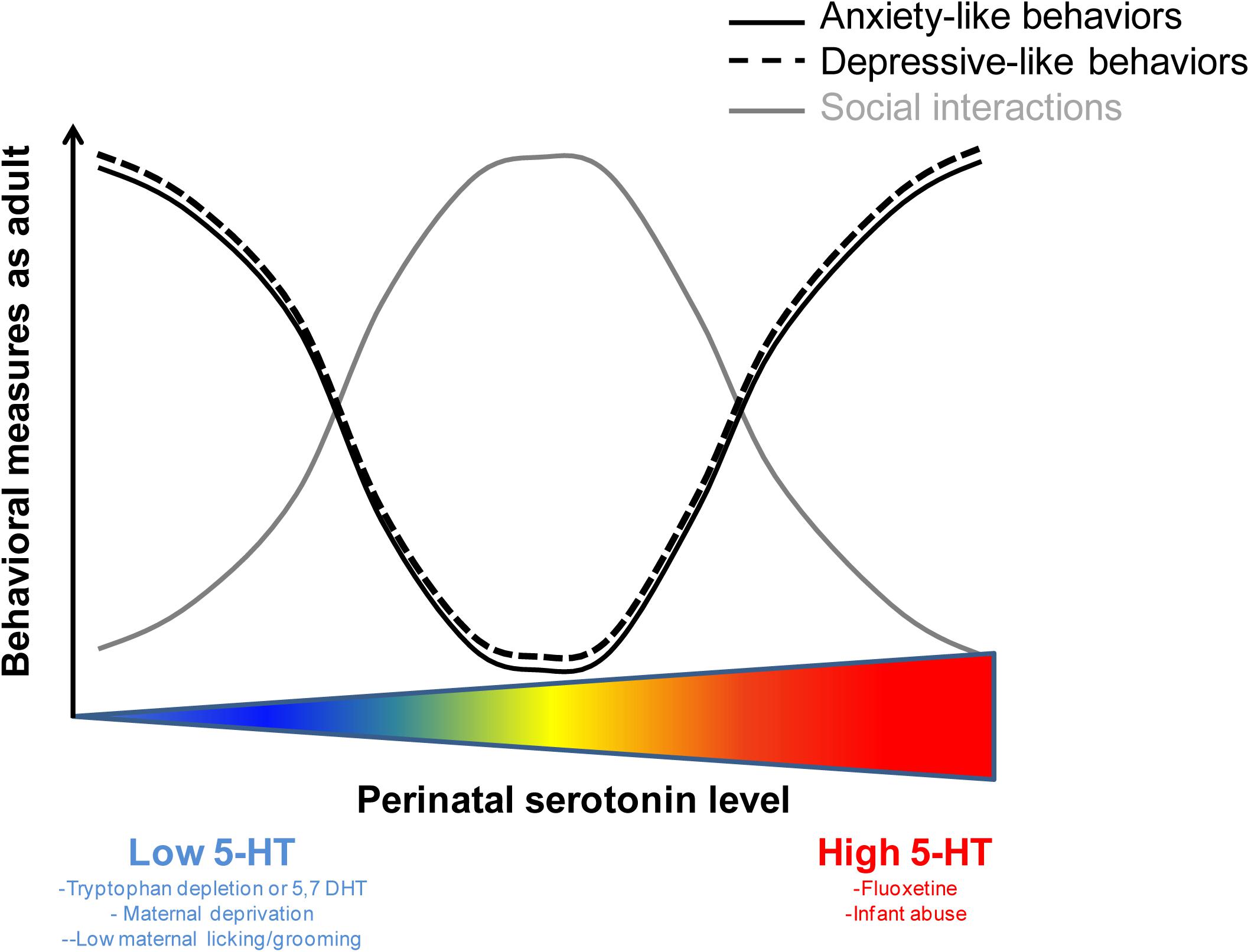
FIGURE 1. The relation between perinatal levels of serotonin and behavioral performance as an adult is non-linear. U-shapped and inversed U-shape curves illustrating the optimal levels of perinatal serotonin sustaining normal behavioral performance in adulthood. Deviation from these optimal levels (either above or below) can lead to behavioral deficits in the adult.
Diet-Induced Disruption of 5-Ht Synthesis: Tryptophan Depletion
Tryptophan is a 5-HT precursor and reducing its availability substantially reduces 5-HT synthesis (Biggio et al., 1974; Moja et al., 1989; Fadda et al., 2000). Since tryptophan cannot be internally synthesized, diets low in tryptophan have been used to decrease 5-HT synthesis.
For ethical reasons, tryptophan depletion studies in humans have only been performed in adults. Such diets have been found to induce depressive symptoms in healthy individuals with a family history of depression and lead to relapse in depressed patients who were previously treated with selective-serotonin-reuptake-inhibitors (SSRIs) (Benkelfat et al., 1994; Delgado et al., 1999; Klaassen et al., 1999; Quintin et al., 2001). For an in-depth review of the behavioral consequences of acute, adult, tryptophan depletion in humans see (Faulkner and Deakin, 2014).
In rodents, only a few studies have examined the long-term effects of low tryptophan diet during early development. Early studies (Segall and Timiras, 1976; Segall et al., 1983) found that female rats starting a diet low in tryptophan at an early developmental age (weaning age-3 weeks) displayed a delay in growth, ovarian function and sexual maturation. Those effects were suggested to be related to low 5-HT in the pituitary-pineal glands. More recently, administration of a low tryptophan diet to lactating dams was found to reduce maternal care. Importantly, this intervention also induced anxiety-like and anhedonia-related behaviors in the adult offspring (Zoratto et al., 2013). Moreover, early life administration of a maize-based diet, containing approximately 20% of tryptophan of regular diets led to increased immobility in the forced-swim-test, reduced time in the open arms of the elevated plus maze, decreased neurogenesis and abnormal dendritic development in the dentate gyrus at 4 weeks of age (Serfaty et al., 2008).
Tryptophan depletion and pharmacologically disrupting 5-HT synthesis using a selective neurotoxin, 5,7-dihydroxytryptamine (5,7 DHT), greatly differ given that the latter leads to permanent neuronal loss. However, the long-term effects of a low-tryptophan diet can be compared to the effects of disrupting 5-HT synthesis with 5,7 DHT. Indeed, administering this neurotoxin during development also disrupts behavior in the adult. 5,7 DHT administration at P3 in rats led to reduced locomotor activity, attenuated social interaction and increased ultrasonic vocalization during fear-conditioning (Rok-Bujko et al., 2012). In addition, depletion of serotonin (P10-20) using the tryptophan hydroxylase inhibitor (parachlorophenylalanine, PCPA) decreases dendritic density (microtubule-associated protein) in hippocampus up to P62 and produces deficits in spatial learning and delayed extinction interpreted as a deficit in normal response inhibition (Mazer et al., 1997). The effects in adulthood of a transient 5-HT disruption during development highlight the importance of precise regulation of serotonergic levels during critical developmental periods.
In summary, reducing 5-HT synthesis during perinatal life, through tryptophan depletion or 5,7 DHT administration, leads to maladaptive behavioral phenotypes including depressive-like and anxiety-like symptoms as well as defective social interactions.
Pharmacological Modifications of Perinatal 5-Ht Levels: Exposure to SSRIs
SSRIs are the most prescribed treatments for depression due to their safety and mild-to-moderate adverse effects, with several being approved for use in children and adolescents (Henry et al., 2012). Pregnancy is a risk factor for depression and SSRIs are taken by a substantial proportion of women (∼ 10%) during pregnancy (Cooper et al., 2007; Huybrechts et al., 2013) possibly exposing the fetus to increased 5-HT levels. SSRIs cross the placenta barrier (Hendrick et al., 2003; Rampono et al., 2009; Sit et al., 2011) and can also be passed to the baby via breast milk (Kristensen et al., 1999). Notably, due to the possibly devastating effects of depression, including potential suicide, discontinuation of antidepressant treatment during pregnancy is often problematic.
Because of the altricial nature of rodents, the 3rd trimester of pregnancy in humans corresponds in terms of development of the serotonergic system to the first postnatal weeks in rodents (Suri et al., 2014). This is the period during which the serotonergic system’s development is at its peak (Suri et al., 2014), which presumably makes it more vulnerable to disruption. To test the influence of SSRIs during the perinatal stage in rodents, two approaches have been taken. These are exposure to SSRIs in utero or during the early-postnatal period (Kepser and Homberg, 2015). In-depth reviews on the effects of developmental exposure to antidepressant medications can be found in (Olivier et al., 2013; Kepser and Homberg, 2015).
The offspring of pregnant rats exposed to the prototypic SSRI, fluoxetine, given orally at 12 mg/kg, from E6 to E20 showed a decrease in birth weight, a transient delay in motor development and improvements in performance in the water maze and passive avoidance tests tested post-weaning (Bairy et al., 2007). In Olivier et al. (2011), pregnant rats were injected daily with 12 mg/kg of fluoxetine from E11 until birth. Adult offspring exhibited increased anxiety-like behaviors in the novelty-suppressed feeding test, the footshock-induced conditioned place aversion test and in the elevated plus maze (Olivier et al., 2011). In Rincon-Cortes et al. (2015), daily administration of 8 mg/kg of fluoxetine to rat pups for 10 days during infancy produced depressive-like behaviors in the adult, i.e., increased immobility in the forced-swim-test (Rincon-Cortes et al., 2015). Postnatal exposure to 10 mg/kg fluoxetine from P2 to P21 in mice led to decreased exploratory behavior, increased anxiety-like behavior in the novelty-suppressed feeding paradigm and impaired active avoidance performance at adulthood (Ansorge et al., 2004; Yu et al., 2014). Furthermore, this treatment reduced aggression when the mice were tested as adults (Yu et al., 2014). Interestingly, the detrimental effects of fluoxetine were restricted to a critical period from P2 to P11 (Rebello et al., 2014). Indeed, fluoxetine administration during this period, but not after, was sufficient to cause exploratory deficits, increased latency to feed in the novelty-suppressed feeding test and increases in escape latency in the shock-avoidance test (Rebello et al., 2014). Furthermore, these mice showed depression-like behaviors with decreased sucrose consumption and increased immobility time in the forced-swim-test (Rebello et al., 2014). Interestingly, these deleterious effects were specific to SSRIs. Postnatal treatment with fluoxetine, clomipramine and citalopram all produced emotional behavioral deficits in mice while administration of the norepinephrine transporter inhibitor, desipramine, did not (Ansorge et al., 2008).
Concerning the possible neurobiological mechanisms underlying the lasting effects of fluoxetine administered during the critical P2-P11 period, modulation of the serotonergic system during this period has been shown to lead to changes in prefrontal cortex pyramidal neuron morphology and reduced excitability (Rebello et al., 2014). 5-HT2 receptors are major modulators of cortical serotonergic signaling. 5-HT2 activation results in neuronal depolarization and increases excitatory postsynaptic currents (Celada et al., 2013b). Interestingly, blocking 5-HT2 signaling concomitantly with fluoxetine administration in pups prevents the behavioral deficits observed with fluoxetine administration alone (Sarkar et al., 2014), suggesting that 5-HT2 receptors may mediate serotonergic effects during this period.
In humans, although fewer studies exist than in rodents, there is also some evidence that perinatal exposure to SSRIs can lead to behavioral deficits. In a study using Finnish national registry data, the incidence of depression by age 14.9 years in offspring exposed prenatally to SSRIs was 8.2% (N = 15,729) compared to 1.9% (N = 9,651) in the control group with psychiatric disorders but no medication (Malm et al., 2016). In addition to possible effects in mood-related development, exposure to SSRIs during development has been associated with an increased incidence of autism in children (Boukhris et al., 2015), which is congruent with the observation of hyperserotonemia in close to one third of autistic children (Hranilovic et al., 2009). An in depth review of the effects of perinatal SSRI administration in humans and animal models can be found in (Olivier et al., 2013).
In summary, increasing 5-HT signaling during a critical period of perinatal life, via exposure to SSRIs, has been shown to lead to emotional deficits in adulthood (e.g., increased anxiety-like and depressive-like behaviors).
Early-Life Maternal Care: Relationship With the Serotonergic System and Long-Term Behavioral Consequences
Emotional deficits related to poor quality of care during early-life are a major social issue. The prevalence of child maltreatment is alarmingly high (Finkelhor et al., 2009) with important societal consequences (U.S. Department of Health and Human Services, 2010). Indeed, early life experience is critical for life-long mental health as it is a period of high plasticity (Chaudhury et al., 2016). One of the major environmental inputs during early life is the caregiver. Importantly, in both humans and rodents, fear and stress responses (Routh et al., 1978; Hellstrom et al., 2012; Rincon-Cortes et al., 2015) as well as changes in mood (Vetulani, 2013) and drug consumption (Vetulani, 2013) are regulated by the quality of maternal care during early life.
Numerous studies have demonstrated the link between maternal care, serotonergic signaling and later vulnerability to mental health issues. Seminal work by Meaney and colleagues describe an increase in basal glucocorticoid levels, as well as in the secretion of glucocorticoids in response to stress in rats that were less groomed and licked by their mothers (Hellstrom et al., 2012). This effect was found to be mediated through the serotonergic system (Mitchell et al., 1990, 1992; Laplante et al., 2002; Hellstrom et al., 2012). Indeed, Hellstrom et al. (2012) suggest that 5-HT, resulting from licking/grooming, acts via the 5-HT7 receptor to activate a signaling cascade that in turn leads to the activation of transcription factors such as NGFI-A (nerve growth factor-inducible factor A) leading to demethylation of the glucocorticoid receptor promoter (Laplante et al., 2002; Hellstrom et al., 2012).
In monkeys, early-life maternal separation reduced serotonin transporter binding (Ichise et al., 2006) and lowered the levels of cerebrospinal 5-HIAA (a 5-HT metabolite) (Shannon et al., 2005; Maestripieri et al., 2006, 2009). Importantly, naturalistic maternal rejection, associated with lower cerebrospinal fluid 5-HIAA levels, was associated with anxiety-like behaviors later in life (Maestripieri et al., 2006). Furthermore, 5-HIAA in the putamen and 5-HIAA/5-HT ratio measures of 5-HT turnover in the hippocampus (and at trend levels in caudate, Substantia nigra and putamen) were significantly lower in nursery reared compared to mother-reared animals; ethanol drinking was also higher in nursery reared monkeys (Huggins et al., 2012).
In rodents, maternal separation has been shown to affect the tissue level of both 5-HT and 5-HIAA in different brain structures. Arborelius and Eklund (2007) found that maternal separation (P1-P13) modified the tissue levels of 5-HT and 5-HIAA in the dorsal raphe nucleus (both increased during long maternal separation) and in the amygdala (decreased during brief maternal separation) of adult female rats. Moreover, the 5-HIAA/5-HT ratio in the rat prefrontal cortex was decreased by maternal separation (Xue et al., 2013; Gonzalez-Mariscal and Melo, 2017). Analysis of the metabolite/monoamine turnover ratio is a useful estimator of serotonergic activity. An increase in this ratio indicates increased serotonergic turnover usually associated with higher rates of 5-HT release into the synapse (Shannon et al., 1986).
Maternal separation also affects 5-HT receptor expression in adulthood. Indeed, adult animals subjected to maternal separation display modifications of 5-HT1A receptor mRNA expression with a decrease in the dorsal raphe nucleus and an increase in the amygdala (Bravo et al., 2014). Postsynaptic 5-HT1A receptor activation in corticolimbic areas appears anxiolytic, while high density of presynaptic 5-HT1A receptors increases susceptibility to mood disorders and activation of these presynaptic receptors can reduce raphe signaling through a negative-feedback system (Celada et al., 2013a). However, an increase in post-synaptic 5-HT1A in the amygdala can also produce anxiogenic behaviors (Gonzalez et al., 1996). The behavioral endpoint of the two distinct regional alterations in 5-HT1A receptor levels may be dictated by the differential density of this receptor in each structure.
In addition, maternal separation also leads to a dysregulation of 5-HT2 receptor function in the prefrontal cortex (Benekareddy et al., 2010). Behaviorally, 5-HT2A agonist-induced head-twitch responses, a model for psychosis and hallucinations in rodents, are potentiated by maternal separation (Benekareddy et al., 2010). Moreover, maternal separation increases anxiety-like behaviors (measured in the open field and the elevated-plus-maze test) and 5-HT2 receptor expression. These effects have been shown to be blocked by treatment with the 5-HT2 antagonist, ketanserin (Benekareddy et al., 2011). Developmental physical abuse as modeled by paired odor-shock conditioning, has also been shown to increase 5-HT levels and lead to deficits in social behavior and increased immobility in the forced swim-test in the adult (Rincon-Cortes et al., 2015). Importantly, both deficits can be rescued by increasing amygdala 5-HT and suppressing corticosterone (Rincon-Cortes et al., 2015), an effect that may be paralleled to the aforementioned work of Hellstrom et al. (2012).
In summary, early life maternal separation or abuse induce modifications of serotonergic signaling (for example increased 5-HT level or increased 5-HT2 receptor expression) that are correlated with later-on maladaptive behaviors such as deficits in social interactions and anxiety-like and depressive-like behaviors in adulthood.
Discussion
In this mini-review, we highlighted the striking and enduring effects on adult behavior of both low and high 5-HT levels during the perinatal period. However, many questions remain. Notably, how do both low and high 5-HT levels during development lead to similar behavioral deficits? One hypothesis is that both can lead to a reduction in serotonergic signaling in the adult. For instance, tryptophan depletion may directly impair the development of the serotonergic system (Mazer et al., 1997) while excessive serotonergic signaling may reduce serotonergic activity/development through negative feedback. Serotonergic innervation peaks during the perinatal period. Perhaps excessive serotonin during this period falsely signals a sufficiency of serotonergic growth leading to stunted development. In fact, maternal inflammation was recently found to lead to increased levels of 5-HT in the fetal brain which subsequently inhibits serotonergic development (Goeden et al., 2016). Furthermore, the firing rate of serotonergic cells in the dorsal raphe of postnatal fluoxetine-treated animals decreases dramatically during adulthood (Teissier et al., 2015). Future experiments, taking advantage of the ability to apply opto- and chemo-genetics tools in neonatal models (Bitzenhofer et al., 2017) can further help dissect how both high and low 5-HT levels can disrupt the development of the serotonergic system and affect behavior in the adult.
This review highlights the critical period when any deviations of 5-HT levels can lead to later maladaptive behavior. For example, Rebello et al. (2014) demonstrated that vulnerability to fluoxetine is restricted to P2-P11. This apparent critical period can be explained by (1) the timeline of brain developmental processes such as proliferation of neurons and glia, migration, apoptosis, and synaptogenesis encompassing both the embryonic stages as well as the first postnatal weeks in rats (Rice and Barone, 2000) and (2) the ontogeny of serotonergic system development [for review see (Booij et al., 2015)]. Indeed, while the serotonergic system continues to mature after birth, there are also critical shifts of receptor expression and activity during this period. For example, from P6 to P19, pyramidal neurons change from being depolarized to being hyperpolarized by 5-HT through different 5-HT receptor subtypes (Beique et al., 2004).
Another question that remains unsettled related to whether the vulnerability to abnormal 5-HT during the perinatal period leading to maladaptive behaviors in adulthood differs in males and females. Indeed, as shown in Table 1, except for the early exposure to SSRIs, most prior studies have only used male animals. While sex differences in animal models of vulnerability to psychiatric disorder and early life stress are increasingly demonstrated (Dalla et al., 2010; Reincke and Hanganu-Opatz, 2017) and a sexual dimorphism in serotonergic system activity in adults is known (Carlsson and Carlsson, 1988; Haleem et al., 1990), the systematic study of the effects of 5-HT level variations during the perinatal period in both sexes remains a high priority.
In this review, we chose to present examples of three exogenous factors supporting the hypothesis of a non-linear relationship between serotonin-levels during early-life and adult behavior. In addition to the models on which we focused, other exogenous factors and genetic models/variants support the hypothesis that both high and low levels of 5-HT during development lead to behavioral deficits, and more specifically to emotional deficits. MAO-A/B-KO mice, which have increased monoamine levels, show a significantly decreased distance traveled in the open-field, and a reduction in the time spent in open-arms in the elevated-plus-maze (Chen et al., 2004). Similarly, constitutive SERT-KO mice, with elevated 5-HT levels, present anxiety-like and depression-like behaviors such as reduced locomotor activity in the open-field, increased latency-to-feed in the novelty-suppressed-feeding test and social-interaction deficits (Lira et al., 2003; Ansorge et al., 2004; Kalueff et al., 2007). Hyposerotonergic function in Lmx1b-raphe specific-KO mice, leads to increased freezing in the fear conditioning test and impaired water-maze memory retrieval (Dai et al., 2008). Constitutively reduced serotonergic levels in a model of SERT-overexpression resulted in reduced anxiety-like phenotypes in the elevated-plus-maze and in the hyponeophagia tests (Jennings et al., 2006). Although the latter study suggests that hyposerotonergic function may be protective of anxiety-like behaviors, other studies using mutant mice with severe 5-HT ablations point to the inverse. While Pet1-KO mice, which only retain residual serotonergic innervation, exhibited reduced anxiety-like behaviors and increased fear responses in one study (Kiyasova et al., 2011), Pet1-KO mice showed increased anxiety-like behaviors and increased aggression in another (Hendricks et al., 2003). Furthermore, Tph2- R441H-KI mice, in which 5-HT brain levels are approximately 80% of wildtype, exhibit increased immobility in the tail suspension test and increased latency to cross to the light chamber in the Dark-Light test (Beaulieu et al., 2008).
Finally, these studies raise the urgent question of whether the behavioral deficits caused by altered 5-HT levels during development can be reversed in adulthood. In Lmx1b-raphe specific-KO mice, plasticity deficits were rescued with a bath application of 5-HT (Dai et al., 2008). Interestingly, Tph2- R441H-KI behavioral deficits could be rescued by administration of a GSK3-β inhibitor (Beaulieu et al., 2008). However, it is striking that adult behavior is impaired in both the SERT-KO mouse (lifelong impaired serotonergic transporter) and the temporally restricted administration of a transporter blocker during perinatal-life (fluoxetine from P4-P21) (Ansorge et al., 2004). This demonstrates that increased serotonergic tone in the adult SERT-KO is not sufficient to rescue the deficits caused by increased serotonergic signaling during development.
Conclusion
High perinatal plasticity allows the brain to adapt to its environment but also marks a period of increased vulnerability. The regulation of serotonin levels during development is extremely important for typical brain development. Both low and high levels of serotonergic signaling seem to be detrimental, leading to multiple behavioral deficits. Many exogenous factors can affect 5-HT levels during development. Future studies need to address the boundary conditions and underlying mechanisms of optimal 5-HT levels during the critical perinatal period to optimize long-term behavioral and emotional outcomes.
Author Contributions
All authors listed have made a substantial, direct and intellectual contribution to the work, and approved it for publication.
Funding
This work was supported by NICHD grant R03HD094978 to CT.
Conflict of Interest Statement
The authors declare that the research was conducted in the absence of any commercial or financial relationships that could be construed as a potential conflict of interest.
References
Ansorge, M. S., Morelli, E., and Gingrich, J. A. (2008). Inhibition of serotonin but not norepinephrine transport during development produces delayed, persistent perturbations of emotional behaviors in mice. J. Neurosci. 28, 199–207. doi: 10.1523/JNEUROSCI.3973-07.2008
Ansorge, M. S., Zhou, M., Lira, A., Hen, R., and Gingrich, J. A. (2004). Early-life blockade of the 5-HT transporter alters emotional behavior in adult mice. Science 306, 879–881. doi: 10.1126/science.1101678
Arborelius, L., and Eklund, M. B. (2007). Both long and brief maternal separation produces persistent changes in tissue levels of brain monoamines in middle-aged female rats. Neuroscience 145, 738–750. doi: 10.1016/j.neuroscience.2006.12.007
Bairy, K. L., Madhyastha, S., Ashok, K. P., Bairy, I., and Malini, S. (2007). Developmental and behavioral consequences of prenatal fluoxetine. Pharmacology 79, 1–11. doi: 10.1159/000096645
Beaulieu, J.-M., Zhang, X., Rodriguiz, R. M., Sotnikova, T. D., Cools, M. J., Wetsel, W. C., et al. (2008). Role of GSK3β in behavioral abnormalities induced by serotonin deficiency. Proc. Natl. Acad. Sci. U.S.A. 105, 1333–1338. doi: 10.1073/pnas.0711496105
Beique, J. C., Campbell, B., Perring, P., Hamblin, M. W., Walker, P., Mladenovic, L., et al. (2004). Serotonergic regulation of membrane potential in developing rat prefrontal cortex: coordinated expression of 5-hydroxytryptamine (5-HT)1A, 5-HT2A, and 5-HT7 receptors. J. Neurosci. 24, 4807–4817. doi: 10.1523/JNEUROSCI.5113-03.2004
Benekareddy, M., Goodfellow, N. M., Lambe, E. K., and Vaidya, V. A. (2010). Enhanced function of prefrontal serotonin 5-HT(2) receptors in a rat model of psychiatric vulnerability. J. Neurosci. 30, 12138–12150. doi: 10.1523/JNEUROSCI.3245-10.2010
Benekareddy, M., Vadodaria, K. C., Nair, A. R., and Vaidya, V. A. (2011). Postnatal serotonin type 2 receptor blockade prevents the emergence of anxiety behavior, dysregulated stress-induced immediate early gene responses, and specific transcriptional changes that arise following early life stress. Biol. Psychiatry 70, 1024–1032. doi: 10.1016/j.biopsych.2011.08.005
Benkelfat, C., Ellenbogen, M. A., Dean, P., Palmour, R. M., and Young, S. N. (1994). Mood-lowering effect of tryptophan depletion. Enhanced susceptibility in young men at genetic risk for major affective disorders. Arch. Gen. Psychiatry 51, 687–697. doi: 10.1001/archpsyc.1994.03950090019003
Biggio, G., Fadda, F., Fanni, P., Tagliamonte, A., and Gessa, G. L. (1974). Rapid depletion of serum tryptophan, brain tryptophan, serotonin and 5-hydroxyindoleacetic acid by a tryptophan-free diet. Life Sci. 14, 1321–1329. doi: 10.1016/0024-3205(74)90440-8
Bitzenhofer, S. H., Ahlbeck, J., and Hanganu-Opatz, I. L. (2017). Methodological approach for optogenetic manipulation of neonatal neuronal networks. Front. Cell. Neurosci. 11:239. doi: 10.3389/fncel.2017.00239
Bonnin, A., Peng, W., Hewlett, W., and Levitt, P. (2006). Expression mapping of 5-HT1 serotonin receptor subtypes during fetal and early postnatal mouse forebrain development. Neuroscience 141, 781–794. doi: 10.1016/j.neuroscience.2006.04.036
Booij, L., Tremblay, R. E., Szyf, M., and Benkelfat, C. (2015). Genetic and early environmental influences on the serotonin system: consequences for brain development and risk for psychopathology. J. Psychiatry Neurosci. 40, 5–18. doi: 10.1503/jpn.140099
Boukhris, T., Sheehy, O., Mottron, L., and Berard, A. (2015). Antidepressant use during pregnancy and the risk of autism spectrum disorder in children. JAMA Pediatr. 170, 117–124. doi: 10.1001/jamapediatrics.2015.3356
Bravo, J. A., Dinan, T. G., and Cryan, J. F. (2014). Early-life stress induces persistent alterations in 5-HT1A receptor and serotonin transporter mRNA expression in the adult rat brain. Front. Mol. Neurosci. 7:24. doi: 10.3389/fnmol.2014.00024
Brummelte, S., Mc Glanaghy, E., Bonnin, A., and Oberlander, T. F. (2017). Developmental changes in serotonin signaling: implications for early brain function, behavior and adaptation. Neuroscience 342, 212–231. doi: 10.1016/j.neuroscience.2016.02.037
Carlsson, M., and Carlsson, A. (1988). A regional study of sex differences in rat brain serotonin. Prog. Neuropsychopharmacol. Biol. Psychiatry 12, 53–61.
Celada, P., Bortolozzi, A., and Artigas, F. (2013a). Serotonin 5-HT1A receptors as targets for agents to treat psychiatric disorders: rationale and current status of research. CNS Drugs 27, 703–716. doi: 10.1007/s40263-013-0071-0
Celada, P., Puig, M. V., and Artigas, F. (2013b). Serotonin modulation of cortical neurons and networks. Front. Integr. Neurosci. 7:25. doi: 10.3389/fnint.2013.00025
Chameau, P., Inta, D., Vitalis, T., Monyer, H., Wadman, W. J., and van Hooft, J. A. (2009). The N-terminal region of reelin regulates postnatal dendritic maturation of cortical pyramidal neurons. Proc. Natl. Acad. Sci. U.S.A. 106, 7227–7232. doi: 10.1073/pnas.0810764106
Chaudhury, S., Sharma, V., Kumar, V., Nag, T. C., and Wadhwa, S. (2016). Activity-dependent synaptic plasticity modulates the critical phase of brain development. Brain Dev. 38, 355–363. doi: 10.1016/j.braindev.2015.10.008
Chen, K., Holschneider, D. P., Wu, W., Rebrin, I., and Shih, J. C. (2004). A spontaneous point mutation produces monoamine oxidase A/B knock-out mice with greatly elevated monoamines and anxiety-like behavior. J. Biol. Chem. 279, 39645–39652. doi: 10.1074/jbc.M405550200
Cooper, W. O., Willy, M. E., Pont, S. J., and Ray, W. A. (2007). Increasing use of antidepressants in pregnancy. Am. J. Obstet. Gynecol. 196, 544.e1–544.e5. doi: 10.1016/j.ajog.2007.01.033
Dai, J.-X., Han, H.-L., Tian, M., Cao, J., Xiu, J.-B., Song, N.-N., et al. (2008). Enhanced contextual fear memory in central serotonin-deficient mice. Proc. Natl. Acad. Sci. U.S.A. 105, 11981–11986. doi: 10.1073/pnas.0801329105
Dalla, C., Pitychoutis, P. M., Kokras, N., and Papadopoulou-Daifoti, Z. (2010). Sex differences in animal models of depression and antidepressant response. Basic Clin. Pharmacol. Toxicol. 106, 226–233. doi: 10.1111/j.1742-7843.2009.00516.x
Daubert, E. A., and Condron, B. G. (2010). Serotonin: a regulator of neuronal morphology and circuitry. Trends Neurosci. 33, 424–434. doi: 10.1016/j.tins.2010.05.005
Delgado, P. L., Miller, H. L., Salomon, R. M., Licinio, J., Krystal, J. H., Moreno, F. A., et al. (1999). Tryptophan-depletion challenge in depressed patients treated with desipramine or fluoxetine: implications for the role of serotonin in the mechanism of antidepressant action. Biol. Psychiatry 46, 212–220. doi: 10.1016/S0006-3223(99)00014-1
Fadda, F., Cocco, S., and Stancampiano, R. (2000). A physiological method to selectively decrease brain serotonin release. Brain Res. Brain Res. Protoc. 5, 219–222. doi: 10.1016/S1385-299X(00)00016-7
Faulkner, P., and Deakin, J. F. (2014). The role of serotonin in reward, punishment and behavioural inhibition in humans: insights from studies with acute tryptophan depletion. Neurosci. Biobehav. Rev. 46(Pt 3), 365–378. doi: 10.1016/j.neubiorev.2014.07.024
Finkelhor, D., Turner, H., Ormrod, R., and Hamby, S. L. (2009). Violence, abuse, and crime exposure in a national sample of children and youth. Pediatrics 124, 1411–1423. doi: 10.1542/peds.2009-0467
Francis, D., Diorio, J., Liu, D., and Meaney, M. J. (1999). Nongenomic transmission across generations of maternal behavior and stress responses in the rat. Science 286, 1155–1158. doi: 10.1126/science.286.5442.1155
Fricker, A. D., Rios, C., Devi, L. A., and Gomes, I. (2005). Serotonin receptor activation leads to neurite outgrowth and neuronal survival. Brain Res. Mol. Brain Res. 138, 228–235. doi: 10.1016/j.molbrainres.2005.04.016
Gaspar, P., Cases, O., and Maroteaux, L. (2003). The developmental role of serotonin: news from mouse molecular genetics. Nat. Rev. Neurosci. 4, 1002–1012. doi: 10.1038/nrn1256
Gingrich, J. A., Malm, H., Ansorge, M. S., Brown, A., Sourander, A., Suri, D., et al. (2017). New insights into how serotonin selective reuptake inhibitors shape the developing brain. Birth Defects Res. 109, 924–932. doi: 10.1002/bdr2.1085
Goeden, N., Velasquez, J., Arnold, K. A., Chan, Y., Lund, B. T., Anderson, G. M., et al. (2016). Maternal inflammation disrupts fetal neurodevelopment via increased placental output of serotonin to the fetal brain. J. Neurosci. 36, 6041–6049. doi: 10.1523/JNEUROSCI.2534-15.2016
Gonzalez, L. E., Andrews, N., and File, S. E. (1996). 5-HT1A and benzodiazepine receptors in the basolateral amygdala modulate anxiety in the social interaction test, but not in the elevated plus-maze. Brain Res. 732, 145–153. doi: 10.1016/0006-8993(96)00517-3
Gonzalez-Mariscal, G., and Melo, A. I. (2017). Bidirectional effects of mother-young contact on the maternal and neonatal brains. Adv. Exp. Med. Biol. 1015, 97–116. doi: 10.1007/978-3-319-62817-2_6
Haleem, D. J., Kennett, G. A., and Curzon, G. (1990). Hippocampal 5-hydroxytryptamine synthesis is greater in female rats than in males and more decreased by the 5-HT1A agonist 8-OH-DPAT. J. Neural Transm. Gen. Sect. 79, 93–101. doi: 10.1007/BF01251004
Hellstrom, I. C., Dhir, S. K., Diorio, J. C., and Meaney, M. J. (2012). Maternal licking regulates hippocampal glucocorticoid receptor transcription through a thyroid hormone–serotonin–NGFI-A signalling cascade. Philos. Trans. R. Soc. B 367, 2495–2510. doi: 10.1098/rstb.2012.0223
Hendrick, V., Stowe, Z. N., Altshuler, L. L., Hwang, S., Lee, E., and Haynes, D. (2003). Placental passage of antidepressant medications. Am. J. Psychiatry 160, 993–996. doi: 10.1176/appi.ajp.160.5.993
Hendricks, T. J., Fyodorov, D. V., Wegman, L. J., Lelutiu, N. B., Pehek, E. A., Yamamoto, B., et al. (2003). Pet-1 ETS gene plays a critical role in 5-HT neuron development and is required for normal anxiety-like and aggressive behavior. Neuron 37, 233–247. doi: 10.1016/S0896-6273(02)01167-4
Henry, A., Kisicki, M. D., and Varley, C. (2012). Efficacy and safety of antidepressant drug treatment in children and adolescents. Mol. Psychiatry 17, 1186–1193. doi: 10.1038/mp.2011.150
Hohmann, C. F., Hamon, R., Batshaw, M. L., and Coyle, J. T. (1988). Transient postnatal elevation of serotonin levels in mouse neocortex. Brain Res. 471, 163–166. doi: 10.1016/0165-3806(88)90163-0
Hranilovic, D., Bujas-Petkovic, Z., Tomicic, M., Bordukalo-Niksic, T., Blazevic, S., and Cicin-Sain, L. (2009). Hyperserotonemia in autism: activity of 5HT-associated platelet proteins. J. Neural Transm. 116, 493–501. doi: 10.1007/s00702-009-0192-2
Huggins, K. N., Mathews, T. A., Locke, J. L., Szeliga, K. T., Friedman, D. P., Bennett, A. J., et al. (2012). Effects of early life stress on drinking and serotonin system activity in rhesus macaques: 5-hydroxyindoleacetic acid in cerebrospinal fluid predicts brain tissue levels. Alcohol 46, 371–376. doi: 10.1016/j.alcohol.2011.11.003
Huybrechts, K. F., Palmsten, K., Mogun, H., Kowal, M., Avorn, J., Setoguchi-Iwata, S., et al. (2013). National trends in antidepressant medication treatment among publicly insured pregnant women. Gen. Hosp. Psychiatry 35, 265–271. doi: 10.1016/j.genhosppsych.2012.12.010
Ichise, M., Vines, D. C., Gura, T., Anderson, G. M., Suomi, S. J., Higley, J. D., et al. (2006). Effects of early life stress on [11C]DASB positron emission tomography imaging of serotonin transporters in adolescent peer- and mother-reared rhesus monkeys. J. Neurosci. 26, 4638–4643. doi: 10.1523/JNEUROSCI.5199-05.2006
Jennings, K. A., Loder, M. K., Sheward, W. J., Pei, Q., Deacon, R. M., Benson, M. A., et al. (2006). Increased expression of the 5-HT transporter confers a low-anxiety phenotype linked to decreased 5-HT transmission. J. Neurosci. 26, 8955–8964. doi: 10.1523/JNEUROSCI.5356-05.2006
Kalueff, A. V., Fox, M. A., Gallagher, P. S., and Murphy, D. L. (2007). Hypolocomotion, anxiety and serotonin syndrome-like behavior contribute to the complex phenotype of serotonin transporter knockout mice. Genes Brain Behav. 6, 389–400. doi: 10.1111/j.1601-183X.2006.00270.x
Kepser, L. J., and Homberg, J. R. (2015). The neurodevelopmental effects of serotonin: a behavioural perspective. Behav. Brain Res. 277, 3–13. doi: 10.1016/j.bbr.2014.05.022
Khozhai, L. I., and Otellin, V. A. (2012). [Synaptogenesis in the dorsal raphe nucleus of rat medulla oblongata in serotonin deficiency]. Morfologiia 142, 20–24.
Kiyasova, V., Fernandez, S. P., Laine, J., Stankovski, L., Muzerelle, A., Doly, S., et al. (2011). A genetically defined morphologically and functionally unique subset of 5-HT neurons in the mouse raphe nuclei. J. Neurosci. 31, 2756–2768. doi: 10.1523/JNEUROSCI.4080-10.2011
Klaassen, T., Riedel, W. J., van Someren, A., Deutz, N. E., Honig, A., and van Praag, H. M. (1999). Mood effects of 24-hour tryptophan depletion in healthy first-degree relatives of patients with affective disorders. Biol. Psychiatry 46, 489–497. doi: 10.1016/S0006-3223(99)00082-7
Kristensen, J. H., Ilett, K. F., Hackett, L. P., Yapp, P., Paech, M., and Begg, E. J. (1999). Distribution and excretion of fluoxetine and norfluoxetine in human milk. Br. J. Clin. Pharmacol. 48, 521–527. doi: 10.1046/j.1365-2125.1999.00040.x
Lambe, E. K., Krimer, L. S., and Goldman-Rakic, P. S. (2000). Differential postnatal development of catecholamine and serotonin inputs to identified neurons in prefrontal cortex of rhesus monkey. J. Neurosci. 20, 8780–8787. doi: 10.1523/JNEUROSCI.20-23-08780.2000
Laplante, P., Diorio, J., and Meaney, M. J. (2002). Serotonin regulates hippocampal glucocorticoid receptor expression via a 5-HT7 receptor. Brain Res. Dev. Brain Res. 139, 199–203. doi: 10.1016/S0165-3806(02)00550-3
Lauder, J. M. (1990). Ontogeny of the serotonergic system in the rat: serotonin as a developmental signal. Ann. N. Y. Acad. Sci. 600, 297–313; discussion314. doi: 10.1111/j.1749-6632.1990.tb16891.x
Lauder, J. M., and Bloom, F. E. (1974). Ontogeny of monoamine neurons in the locus coeruleus, Raphe nuclei and substantia nigra of the rat. I. Cell differentiation. J. Comp. Neurol. 155, 469–481. doi: 10.1002/cne.901550407
Lavdas, A. A., Blue, M. E., Lincoln, J., and Parnavelas, J. G. (1997). Serotonin promotes the differentiation of glutamate neurons in organotypic slice cultures of the developing cerebral cortex. J. Neurosci. 17, 7872–7880. doi: 10.1523/JNEUROSCI.17-20-07872.1997
Levallois, C., Valence, C., Baldet, P., and Privat, A. (1997). Morphological and morphometric analysis of serotonin-containing neurons in primary dissociated cultures of human rhombencephalon: a study of development. Brain Res. Dev. Brain Res. 99, 243–252. doi: 10.1016/S0165-3806(97)00026-6
Lidov, H. G., and Molliver, M. E. (1982a). An immunohistochemical study of serotonin neuron development in the rat: ascending pathways and terminal fields. Brain Res. Bull. 8, 389–430.
Lidov, H. G., and Molliver, M. E. (1982b). Immunohistochemical study of the development of serotonergic neurons in the rat CNS. Brain Res. Bull. 9, 559–604.
Lira, A., Zhou, M., Castanon, N., Ansorge, M. S., Gordon, J. A., Francis, J. H., et al. (2003). Altered depression-related behaviors and functional changes in the dorsal raphe nucleus of serotonin transporter-deficient mice. Biol. Psychiatry 54, 960–971. doi: 10.1016/S0006-3223(03)00696-6
Maestripieri, D., Hoffman, C. L., Anderson, G. M., Carter, C. S., and Higley, J. D. (2009). Mother-infant interactions in free-ranging rhesus macaques: relationships between physiological and behavioral variables. Physiol. Behav. 96, 613–619. doi: 10.1016/j.physbeh.2008.12.016
Maestripieri, D., McCormack, K., Lindell, S. G., Higley, J. D., and Sanchez, M. M. (2006). Influence of parenting style on the offspring’s behaviour and CSF monoamine metabolite levels in crossfostered and noncrossfostered female rhesus macaques. Behav. Brain Res. 175, 90–95. doi: 10.1016/j.bbr.2006.08.002
Malm, H., Brown, A. S., Gissler, M., Gyllenberg, D., Hinkka-Yli-Salomaki, S., McKeague, I. W., et al. (2016). Gestational exposure to selective serotonin reuptake inhibitors and offspring psychiatric disorders: a national register-based study. J. Am. Acad. Child Adolesc. Psychiatry 55, 359–366. doi: 10.1016/j.jaac.2016.02.013
Mazer, C., Muneyyirci, J., Taheny, K., Raio, N., Borella, A., and Whitaker-Azmitia, P. (1997). Serotonin depletion during synaptogenesis leads to decreased synaptic density and learning deficits in the adult rat: a possible model of neurodevelopmental disorders with cognitive deficits. Brain Res. 760, 68–73. doi: 10.1016/S0006-8993(97)00297-7
Mitchell, J. B., Betito, K., Rowe, W., Boksa, P., and Meaney, M. J. (1992). Serotonergic regulation of type II corticosteroid receptor binding in hippocampal cell cultures: evidence for the importance of serotonin-induced changes in cAMP levels. Neuroscience 48, 631–639. doi: 10.1016/0306-4522(92)90407-S
Mitchell, J. B., Rowe, W., Boksa, P., and Meaney, M. J. (1990). Serotonin regulates type II corticosteroid receptor binding in hippocampal cell cultures. J. Neurosci. 10, 1745–1752. doi: 10.1523/JNEUROSCI.10-06-01745.1990
Moja, E. A., Cipolla, P., Castoldi, D., and Tofanetti, O. (1989). Dose-response decrease in plasma tryptophan and in brain tryptophan and serotonin after tryptophan-free amino acid mixtures in rats. Life Sci. 44, 971–976. doi: 10.1016/0024-3205(89)90497-9
Murphy, D. L., Fox, M. A., Timpano, K. R., Moya, P. R., Ren-Patterson, R., Andrews, A. M., et al. (2008). How the serotonin story is being rewritten by new gene-based discoveries principally related to SLC6A4, the serotonin transporter gene, which functions to influence all cellular serotonin systems. Neuropharmacology 55, 932–960. doi: 10.1016/j.neuropharm.2008.08.034
Olivier, J. D., Valles, A., van Heesch, F., Afrasiab-Middelman, A., Roelofs, J. J., Jonkers, M., et al. (2011). Fluoxetine administration to pregnant rats increases anxiety-related behavior in the offspring. Psychopharmacology 217, 419–432. doi: 10.1007/s00213-011-2299-z
Olivier, J. D. A., Åkerud, H., Kaihola, H., Pawluski, J. L., Skalkidou, A., Högberg, U., et al. (2013). The effects of maternal depression and maternal selective serotonin reuptake inhibitor exposure on offspring. Front. Cell. Neurosci. 7:73. doi: 10.3389/fncel.2013.00073
Papaioannou, A., Dafni, U., Alikaridis, F., Bolaris, S., and Stylianopoulou, F. (2002). Effects of neonatal handling on basal and stress-induced monoamine levels in the male and female rat brain. Neuroscience 114, 195–206. doi: 10.1016/S0306-4522(02)00129-X
Quintin, P., Benkelfat, C., Launay, J. M., Arnulf, I., Pointereau-Bellenger, A., Barbault, S., et al. (2001). Clinical and neurochemical effect of acute tryptophan depletion in unaffected relatives of patients with bipolar affective disorder. Biol. Psychiatry 50, 184–190. doi: 10.1016/S0006-3223(01)01140-4
Raineki, C., Sarro, E., Rincon-Cortes, M., Perry, R., Boggs, J., Holman, C. J., et al. (2015). Paradoxical neurobehavioral rescue by memories of early-life abuse: the safety signal value of odors learned during abusive attachment. Neuropsychopharmacology 40, 906–914. doi: 10.1038/npp.2014.266
Rampono, J., Simmer, K., Ilett, K. F., Hackett, L. P., Doherty, D. A., Elliot, R., et al. (2009). Placental transfer of SSRI and SNRI antidepressants and effects on the neonate. Pharmacopsychiatry 42, 95–100. doi: 10.1055/s-0028-1103296
Rebello, T. J., Yu, Q., Goodfellow, N. M., Caffrey Cagliostro, M. K., Teissier, A., Morelli, E., et al. (2014). Postnatal day 2 to 11 constitutes a 5-HT-sensitive period impacting adult mPFC function. J. Neurosci. 34, 12379–12393. doi: 10.1523/JNEUROSCI.1020-13.2014
Reincke, S. A., and Hanganu-Opatz, I. L. (2017). Early-life stress impairs recognition memory and perturbs the functional maturation of prefrontal-hippocampal-perirhinal networks. Sci. Rep. 7:42042. doi: 10.1038/srep42042
Rice, D., and Barone, S. Jr. (2000). Critical periods of vulnerability for the developing nervous system: evidence from humans and animal models. Environ. Health Perspect. 108(Suppl. 3), 511–533. doi: 10.1289/ehp.00108s3511
Rincon-Cortes, M., Barr, G. A., Mouly, A. M., Shionoya, K., Nunez, B. S., and Sullivan, R. M. (2015). Enduring good memories of infant trauma: rescue of adult neurobehavioral deficits via amygdala serotonin and corticosterone interaction. Proc. Natl. Acad. Sci. U.S.A. 112, 881–886. doi: 10.1073/pnas.1416065112
Rok-Bujko, P., Krzascik, P., Szyndler, J., Kostowski, W., and Stefanski, R. (2012). The influence of neonatal serotonin depletion on emotional and exploratory behaviours in rats. Behav. Brain Res. 226, 87–95. doi: 10.1016/j.bbr.2011.08.030
Routh, D. K., Walton, M. D., and Padan-Belkin, E. (1978). Development of activity level in children revisited: effects of mother presence. Dev. Psychol. 14, 571–581. doi: 10.1037/0012-1649.14.6.571
Sarkar, A., Chachra, P., and Vaidya, V. A. (2014). Postnatal fluoxetine-evoked anxiety is prevented by concomitant 5-HT2A/C receptor blockade and mimicked by postnatal 5-HT2A/C receptor stimulation. Biol. Psychiatry 76, 858–868. doi: 10.1016/j.biopsych.2013.11.005
Segall, P. E., and Timiras, P. S. (1976). Patho-physiologic findings after chronic tryptophan deficiency in rats: a model for delayed growth and aging. Mech. Ageing Dev. 5, 109–124. doi: 10.1016/0047-6374(76)90012-9
Segall, P. E., Timiras, P. S., and Walton, J. R. (1983). Low tryptophan diets delay reproductive aging. Mech. Ageing Dev. 23, 245–252. doi: 10.1016/0047-6374(83)90024-6
Serfaty, C. A., Oliveira-Silva, P., Faria Melibeu Ada, C., and Campello-Costa, P. (2008). Nutritional tryptophan restriction and the role of serotonin in development and plasticity of central visual connections. Neuroimmunomodulation 15, 170–175. doi: 10.1159/000153421
Shannon, C., Schwandt, M. L., Champoux, M., Shoaf, S. E., Suomi, S. J., Linnoila, M., et al. (2005). Maternal absence and stability of individual differences in CSF 5-HIAA concentrations in rhesus monkey infants. Am. J. Psychiatry 162, 1658–1664. doi: 10.1176/appi.ajp.162.9.1658
Shannon, N. J., Gunnet, J. W., and Moore, K. E. (1986). A comparison of biochemical indices of 5-hydroxytryptaminergic neuronal activity following electrical stimulation of the dorsal raphe nucleus. J. Neurochem. 47, 958–965. doi: 10.1111/j.1471-4159.1986.tb00704.x
Sit, D., Perel, J. M., Wisniewski, S. R., Helsel, J. C., Luther, J. F., and Wisner, K. L. (2011). Mother-infant antidepressant concentrations, maternal depression, and perinatal events. J. Clin. Psychiatry 72, 994–1001. doi: 10.4088/JCP.10m06461
Sodhi, M. S., and Sanders-Bush, E. (2004). Serotonin and brain development. Int. Rev. Neurobiol. 59, 111–174. doi: 10.1016/S0074-7742(04)59006-2
St-Pierre, J., Laurent, L., King, S., and Vaillancourt, C. (2016). Effects of prenatal maternal stress on serotonin and fetal development. Placenta 48(Suppl. 1), S66–S71. doi: 10.1016/j.placenta.2015.11.013
Sundstrom, E., Kolare, S., Souverbie, F., Samuelsson, E. B., Pschera, H., Lunell, N. O., et al. (1993). Neurochemical differentiation of human bulbospinal monoaminergic neurons during the first trimester. Brain Res. Dev. Brain Res. 75, 1–12. doi: 10.1016/0165-3806(93)90059-J
Suri, D., Teixeira, C. M., Cagliostro, M. K., Mahadevia, D., and Ansorge, M. S. (2014). Monoamine-sensitive developmental periods impacting adult emotional and cognitive behaviors. Neuropsychopharmacology 40, 88–112. doi: 10.1038/npp.2014.231
Teissier, A., Chemiakine, A., Inbar, B., Bagchi, S., Ray Russell, S., Palmiter Richard, D., et al. (2015). Activity of raphé serotonergic neurons controls emotional behaviors. Cell Rep. 13, 1965–1976. doi: 10.1016/j.celrep.2015.10.061
U.S. Department of Health and Human Services (2010). Child Maltreatment 2009. Washington DC: US Government Printing Office.
Vetulani, J. (2013). Early maternal separation: a rodent model of depression and a prevailing human condition. Pharmacol. Rep. 65, 1451–1461. doi: 10.1016/S1734-1140(13)71505-6
Vichier-Guerre, C., Parker, M., Pomerantz, Y., Finnell, R. H., and Cabrera, R. M. (2017). Impact of selective serotonin reuptake inhibitors on neural crest stem cell formation. Toxicol. Lett. 281, 20–25. doi: 10.1016/j.toxlet.2017.08.012
Waeber, C., Sebben, M., Nieoullon, A., Bockaert, J., and Dumuis, A. (1994). Regional distribution and ontogeny of 5-HT4 binding sites in rodent brain. Neuropharmacology 33, 527–541. doi: 10.1016/0028-3908(94)90084-1
Weaver, I. C., Cervoni, N., Champagne, F. A., D’Alessio, A. C., Sharma, S., Seckl, J. R., et al. (2004). Epigenetic programming by maternal behavior. Nat. Neurosci. 7, 847–854. doi: 10.1038/nn1276
Xu, Y., Sari, Y., and Zhou, F. C. (2004). Selective serotonin reuptake inhibitor disrupts organization of thalamocortical somatosensory barrels during development. Brain Res. Dev. Brain Res. 150, 151–161. doi: 10.1016/j.devbrainres.2003.02.001
Xue, X., Shao, S., Li, M., Shao, F., and Wang, W. (2013). Maternal separation induces alterations of serotonergic system in different aged rats. Brain Res. Bull. 95, 15–20. doi: 10.1016/j.brainresbull.2013.03.003
Yu, Q., Teixeira, C. M., Mahadevia, D., Huang, Y., Balsam, D., Mann, J. J., et al. (2014). Dopamine and serotonin signaling during two sensitive developmental periods differentially impact adult aggressive and affective behaviors in mice. Mol. Psychiatry 19, 688–698. doi: 10.1038/mp.2014.10
Zhang, L., Guadarrama, L., Corona-Morales, A. A., Vega-Gonzalez, A., Rocha, L., and Escobar, A. (2006). Rats subjected to extended L-tryptophan restriction during early postnatal stage exhibit anxious-depressive features and structural changes. J. Neuropathol. Exp. Neurol. 65, 562–570. doi: 10.1097/00005072-200606000-00004
Keywords: serotonin, development, SSRI, tryptophan depletion, perinatal, maternal separation
Citation: Shah R, Courtiol E, Castellanos FX and Teixeira CM (2018) Abnormal Serotonin Levels During Perinatal Development Lead to Behavioral Deficits in Adulthood. Front. Behav. Neurosci. 12:114. doi: 10.3389/fnbeh.2018.00114
Received: 26 March 2018; Accepted: 17 May 2018;
Published: 06 June 2018.
Edited by:
Bruno Poucet, Centre National de la Recherche Scientifique (CNRS), FranceReviewed by:
Erika Comasco, Uppsala University, SwedenEtienne Sibille, Centre for Addiction and Mental Health, Canada
Copyright © 2018 Shah, Courtiol, Castellanos and Teixeira. This is an open-access article distributed under the terms of the Creative Commons Attribution License (CC BY). The use, distribution or reproduction in other forums is permitted, provided the original author(s) and the copyright owner are credited and that the original publication in this journal is cited, in accordance with accepted academic practice. No use, distribution or reproduction is permitted which does not comply with these terms.
*Correspondence: Catia M. Teixeira, catia.teixeira@nki.rfmh.org; teixeira.catia@gmail.com
†These authors have contributed equally to this work.