Cancer Stem Cells in Neuroblastoma: Expanding the Therapeutic Frontier
- 1Department of Anatomy, Cell Biology and Physiological Sciences, Faculty of Medicine, American University of Beirut, Beirut, Lebanon
- 2Department of Pharmaceutical Sciences, School of Pharmacy, Lebanese American University, Byblos, Lebanon
Neuroblastoma (NB) is the most common extracranial solid tumor often diagnosed in childhood. Despite intense efforts to develop a successful treatment, current available therapies are still challenged by high rates of resistance, recurrence and progression, most notably in advanced cases and highly malignant tumors. Emerging evidence proposes that this might be due to a subpopulation of cancer stem cells (CSCs) or tumor-initiating cells (TICs) found in the bulk of the tumor. Therefore, the development of more targeted therapy is highly dependent on the identification of the molecular signatures and genetic aberrations characteristic to this subpopulation of cells. This review aims at providing an overview of the key molecular players involved in NB CSCs and focuses on the experimental evidence from NB cell lines, patient-derived xenografts and primary tumors. It also provides some novel approaches of targeting multiple drivers governing the stemness of CSCs to achieve better anti-tumor effects than the currently used therapeutic agents.
Introduction
Stem cells are a class of multipotent undifferentiated cells, able to give rise to all cells in a particular tissue, organ or organism. Stem cells are selectively characterized by their endogenous self-renewal ability, continuously maintaining a pool of undifferentiated cells that will further differentiate, during development or following injury, into fully functional cells (Reya et al., 2001). Stem cells were historically further classified into two subtypes: pluripotent embryonic stem cells and adult stem cells. The former arises from a fertilized egg and gives rise to whole-organisms, while the latter represents tissue-specific multipotent cells that reside in adult tissues, and maintain their homeostatic balance (Chen et al., 2015). Mainly, these cells regularly divide to replace old senescent cells, or they get rigorously activated following injury to repair damaged tissues (Gudjonsson and Magnusson, 2005; Daley, 2015).
Recently, a new subpopulation of stem cells was suggested to exist in tumors. This hypothesis originated based on the recurrent clinical course of most cancers, as well as their self-renewal ability and uninhibited growth pattern, two categorical markers of tumorigenesis. This subpopulation of cells is commonly referred to as CSC or TICs. The cancer stem cell was first introduced in leukemias, remarkably distinguishing a population of cells on top of the tumorigenesis hierarchy able to develop and generate malignant hematopoietic colonies (Moore et al., 1973). The heterogenic cellular composition of solid tumors, such as melanoma, further supported the existence of CSCs, thought to be responsible for cancer recurrence after therapy (Menaa et al., 2009). As their nomenclature implies, CSCs possess the ability to self-renew indefinitely, as well as the potential to differentiate into the different types of cells that form the tumor bulk. Nonetheless, while normal stem cells are tightly regulated by tumor-suppressor proteins that control their entry into the cell cycle, cellular division and differentiation, CSCs harbor critical genetic pro-oncogenic mutations that instigate uncontrolled cellular proliferation (Chen et al., 2015), promoting tumor progression and malignancy. Notably, in solid tumors such as gliomas, the self-renewal capacity of CSCs (CD133+ cells) correlated highly with the aggressiveness and clinical grade of these tumors (Singh et al., 2003).
Cancer stem cells are also highly resistant to conventional therapies used to treat cancerous tumors, such as chemotherapy and radiation (Reya et al., 2001; Singh et al., 2003). Despite major advances in the delivery, effectiveness and mechanisms involved in these cancer therapies, cancer recurrence and metastatic growth of an eradicated primary tumor are still serious unfortunate outcomes that are commonly seen in clinical settings. Resistance to chemotherapy and treatment relapse are recently being attributed to our failure to successfully target and suppress these CSCs. While common treatments effectively eliminate a large proportion of differentiated cells within the bulk tumor and hence greatly reducing its size and burden to subclinical levels that cannot be detected using conventional methods, it fails to eradicate the tumor-initiating cells, which further promote tumor growth and progression (Menaa et al., 2009).
The field of CSCs has spurred the attention of many researchers and triggered recent studies to focus on further characterizing these cells, on functional and molecular levels. The identification of molecular markers specific to these cells set the stage for the development of novel therapeutic drugs targeted against them, ultimately aiming at preventing tumor recurrence and treatment resistance. The subpopulation of CSCs is found in many types of malignancies including brain tumors (Dawood et al., 2014), which represent some of the most aggressive cancers found in both children and adults.
Neuroblastoma is the most common extracranial pediatric solid tumor. It often arises in infants and children up to 5 years old (Buhagiar and Ayers, 2015). It originates from embryonic neural crest cells destined to become the sympathetic ganglia of the autonomic nervous system, or the catecholamine-secreting cells of the adrenal glands. Thus, these tumors arise mainly in areas such as the adrenal medulla as well as the neck, chest, and spinal cord, among others (Buhagiar and Ayers, 2015). Interestingly, NB tumors with unfavorable prognosis have been shown to house a population of undifferentiated stem cells, responsible of their superior malignant state (Reya et al., 2001; Singh et al., 2003; Buhagiar and Ayers, 2015). In this review, we go through the latest discoveries that outline the molecular markers of the cancer stem cells in NB, as well as the current platform of CSC targeted novel therapies that achieve a higher therapeutic potential than currently used approaches.
Neuroblastoma Cancer Stem Cells: Molecular Signatures and Genetic Aberrations
Substantial research is aimed at elucidating the molecular signatures of CSCs and specific malignant drivers in order to devise more efficacious therapies. However, the molecular signatures and drivers of malignancy in CSCs seen in solid tumors in general, and NB in particular, are numerous, sometimes overlapping and dynamic. We have previously reported on the RAP in NB that may be rendering it highly malignant and chemotherapy-resistant, a mechanism by which NB CSCs maintain a dynamic ability to revert back-and-forth between anchorage-independent treatment-evasive tumor-spheres and anchorage-dependent, adherent cancer cells in response to environmental stressors, such as therapeutic agents, hypoxia, low pH levels and deprivation of growth factors (Chakrabarti et al., 2012).
Pathways that may be dysregulated by the CSCs have been reported to be involved in invasion and metastasis, uncontrolled proliferation, angiogenic potential, therapeutic resistance and self-renewal abilities (Chakrabarti et al., 2012). Ideal therapeutic strategies necessitate targeting several of these malignant pathways, taking into account the RAP character exhibited by this malignant tumor, in order to adequately cripple the cancer and prevent relapse with metastatic disease. The various, extensively studied molecular signatures pertaining to NB CSCs will be elaborated below (Table 1).
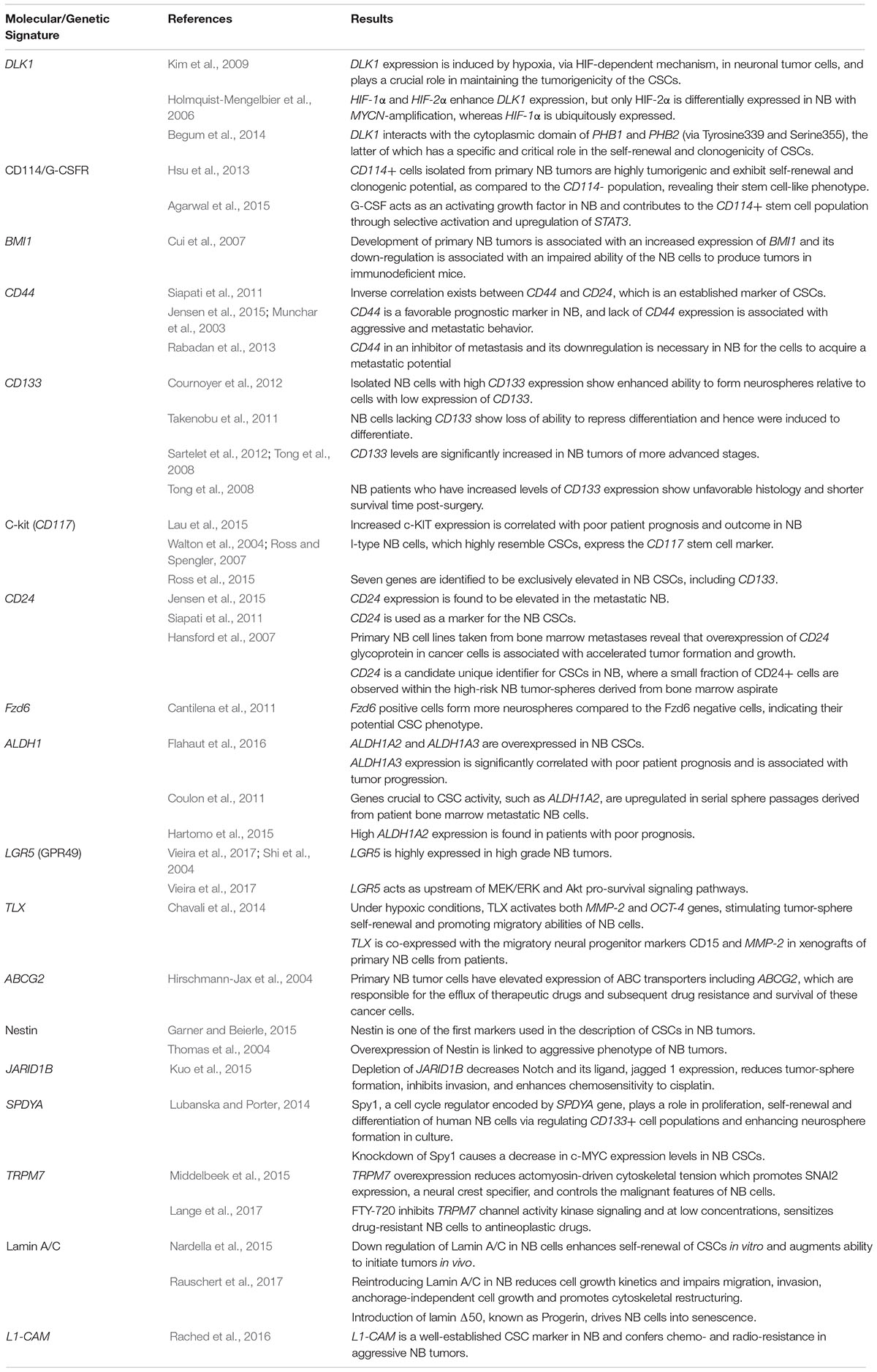
Table 1. Summary table of the major molecular signatures and genetic aberrations attributed to neuroblastoma cancer stem cells.
DLK1 and HIF
The DLK1 gene encodes Protein Delta Homolog 1, a transmembrane protein which belongs to the EGF-like homeotic protein family. This gene is highly expressed in different neuroendocrine tumors, including NB (van Limpt et al., 2000; Kim, 2010) and other nervous system tumors such as gliomas (Yin et al., 2006), and plays a critical role in differentiation processes (Laborda, 2000). DLK1 expression was shown to be induced by hypoxia, via the HIF-dependent mechanism (Kim et al., 2009), in neuronal tumor cells and plays a crucial role in maintaining the tumorigenicity of the CSCs (Kim et al., 2009; Begum et al., 2014). HIF-1α and HIF-2α both enhance DLK1 expression, however, only HIF-2α is differentially expressed in NB with MYCN amplification, whereas HIF-1α is ubiquitously expressed (Holmquist-Mengelbier et al., 2006; Kim et al., 2009). DLK1 also interacts with two molecules, PHB1 and PHB2, the latter of which has a specific and critical role in the self-renewal and clonogenicity of CSCs (Begum et al., 2014). In this sense, DLK1 interacts with the PHB complex through its cytoplasmic domain, and Tyrosine339 and Serine355 are specifically required for the maintenance of clonogenicity and tumorigenicity (Kim et al., 2009; Begum et al., 2014).
CD114/G-CSFR
Granulocyte-Colony Stimulation Factor Receptor expression is well-known as a marker of the CSC population in NB. CD114+ cells isolated from primary NB tumors were shown to be highly tumorigenic, compared to the CD114- population, and exhibited self-renewal and clonogenic potential, revealing their stem cell-like phenotype (Hsu et al., 2013). G-CSF acts as an activating growth factor in NB and contributes to the CD114+ stem cell population growth through selective activation and upregulation of STAT3 (Hsu et al., 2013; Agarwal et al., 2015), a pro-oncogenic transcription factor involved in critical cellular functions, such as growth, division and apoptosis. STAT3 activates G-CSFR transcription in a feed-forward manner, contributing to the maintenance and tumorigenicity of the CSC population (Agarwal et al., 2015). Moreover, G-CSF-STAT3 signaling loop induces the conversion of differentiated cancer cells into CSCs (Hsu et al., 2013). This signaling pathway is also involved in promoting EMT enhancing the migratory and invasive potential of tumor cells (Agarwal et al., 2015).
BMI1
The oncogene BMI1 is a transcription factor, member of the polycomb group family that is essential for the self-renewal capacity of stem cells, specifically in the nervous system (Molofsky et al., 2003; Nowak et al., 2006). Development of primary NB tumors is correlated with an increased expression of BMI1 (Nowak et al., 2006; Cui et al., 2007). On the contrary, BMI1 down-regulation is associated with an impaired ability of the NB cells to produce tumors in immunodeficient mice (Cui et al., 2007). Moreover, the use of transgenic mice, genetically modified with MYCN overexpression, facilitated the monitoring of NB development and revealed an increased expression of BMI1 (Cui et al., 2007).
Although aggressive NB tumors are associated with MYCN amplification, it is important to note that MYCN increases the susceptibility of cells to apoptosis (Lutz et al., 1998; Cui et al., 2007) through the ARF-p53 pathway (Cui et al., 2007). In this context, BMI1 gene mediates its action by inactivating the apoptotic pathway associated with the MYN-N gene amplification, acting as its oncogenic partner (Cui et al., 2007).
CD44
CD44 is a transmembrane glycoprotein involved in cell-cell and cell-ECM interactions (Siapati et al., 2011) and was identified as a CSC marker in numerous pediatric tumors, including NB (Mehrazma et al., 2013). Jensen et al. (2015) showed that metastatic NB was characterized with an increased expression of CD44 marker, compared to non-metastatic controls. Nonetheless, the lack of CD44 expression was associated with aggressive and metastatic behavior, as well as unfavorable outcomes (Munchar et al., 2003; Siapati et al., 2011; Rabadan et al., 2013; Nardella et al., 2015). CD44 is involved in cell-cell adhesion, promoting an antagonistic role vis-a-vis cancer metastasis: its downregulation is thought to be necessary in NB for the cells to acquire a metastatic potential (Rabadan et al., 2013). This was further supported with retrospective studies showing a correlation between increased expression of CD44 and favorable tumors on histology (Munchar et al., 2003). In vitro studies on NB cell lines further investigated the role of CD44 on cellular morphology: whereas CD44- cells had smaller size, lesser neuronal projections, and slower growth, CD44+ cells embraced a more flattened morphology and demonstrated a faster growth, with a protein expression pattern associated with uncontrolled cell cycle progression, immune evasion and lower ability to undergo apoptosis (Siapati et al., 2011). Moreover, one study showed the presence of an inverse correlation between this marker and CD24 (Siapati et al., 2011), a previously established marker of CSCs (Hansford et al., 2007). The importance of CD44 as a prognostic marker was challenged by a study conducted by Mehrazma et al. (2013) which failed to prove any correlation between CD44 expression levels and outcomes in pediatric solid tumors, including NB.
C-kit (CD117)
CD117 is an established marker of stem cells (Hirschmann-Jax et al., 2004; Walton et al., 2004) and proto-oncogene (Lau et al., 2015). It is specifically a factor receptor (Hirschmann-Jax et al., 2004) of the tyrosine kinase type (Lau et al., 2015). It was found that increased C-kit expression is correlated with poor patient prognosis and outcome in NB (Lau et al., 2015).
The heterogeneous nature of NB tumors has yielded the identification of 3 different phenotypic cell variants when placed in culture (Ross and Spengler, 2007): two phenotypically stable cell variants – the moderately malignant, weakly substrate-adherent N-type neuroblastic cells and the flattened, substrate-adherent non-neuronal S-type cells (Rettig et al., 1987; Ciccarone et al., 1989) – and a third stable cell type that is “intermediate” between N- and S-type cells, termed “I-type” and recognized to be a highly tumorigenic, malignant NB CSC (Ross et al., 1995). Interestingly, only the latter I-type NB cells, which resemble CSCs the most, express the CD117 stem cell marker (Walton et al., 2004; Ross and Spengler, 2007).
CD133
CD133, also known as prominin-1, is a 120 kD membrane glycoprotein containing five transmembrane domains. While its specific function remains unknown, this molecule is known to be strictly expressed on protrusions of the plasma membrane of epithelial cells among other cell types (Mizrak et al., 2008). It is well studied as a marker of human hematopoietic stem and progenitor cells (Miraglia et al., 1997; Yin et al., 1997), and of neural stem cells (Mizrak et al., 2008). In addition to its expression in normal stem cells, CD133 is also often used as a marker of CSCs in a variety of tumors, including NB.
In vitro studies have linked the expression of CD133 to tumor-initiating capacities in NB cell lines using the neurosphere formation assay, a functional assay that is used to isolate and assess neural stem cells. CD133+ cells showed an enhanced ability to form neurospheres compared to CD133- cells (Singh et al., 2003; Cournoyer et al., 2012), as well as an increase in average neurosphere size and an enhanced ability of serial passaging, a hallmark of self-renewal (Singh et al., 2003; Cournoyer et al., 2012). Moreover, CD133+ NB cells generated a significantly higher number of colonies in anchorage-independent conditions (Cournoyer et al., 2012). Furthermore, CD133+ cells injected into the adrenal gland were able to form tumors while low CD133- cells failed to do the same (Cournoyer et al., 2012). Many studies have used CD133 as a stem cell marker in order to track changes in the proportion of this population of cells upon exposure to certain conditions (Bhaskara et al., 2012; Chavali et al., 2014).
The role of this molecule was further investigated in cancer progression and tumorigenesis. CD133 knockdown experiments revealed significantly increased differentiation patterns within NB cell lines; CD133 turned out to play a key role inhibiting differentiation partially through regulating signal transduction downstream of RET tyrosine kinase (Takenobu et al., 2011). Moreover, upon exposure of NB CSCs to signals inducing cellular differentiation, CD133 expression levels were shown to decrease (Takenobu et al., 2011). On the clinical level, CD133 levels were seen to be significantly increased in tumors of advanced staging (Tong et al., 2008; Sartelet et al., 2012; Mehrazma et al., 2013). Specifically, patients who were found to have increased levels of CD133 expression also showed unfavorable histology and a shorter survival time post-surgery (Tong et al., 2008). In support of this, a microarray study was performed on several NB cell lines and revealed the presence of seven different genes whose expression was elevated in the highly tumorigenic “I-type” cells, one of which is CD133 (Ross et al., 2015). The presence of I-cells in NB tumors is currently recognized as an indicator of tumor malignancy (Walton et al., 2004).
CD24
CD24 is a cell adhesion molecule expressed on a variety of cells, such as neural cells and cells of the adrenal medulla, and in several cancers including NB (Akashi et al., 1994). CD24 expression was found to be elevated in the metastatic NB (Jensen et al., 2015) in one study, and was used as a marker for the NB CSCs in another (Siapati et al., 2011). Additionally, primary NB cell lines taken from bone marrow metastases revealed that overexpression of the CD24 glycoprotein in cancer cells is associated with accelerated tumor formation and growth (Hansford et al., 2007). Besides, CD24 had been shown to serve as a candidate unique identifier for CSCs in NB, where a small fraction of CD24+ cells were observed within the high-risk NB tumor-spheres derived from bone marrow aspirates (Hansford et al., 2007).
Fzd6
A study examining a possible correlation between expression of frizzled receptors and NB prognosis found that only Fzd6 was directly linked with poor survival in NB patients. Furthermore, Fzd6 positive cells within these tumors was found to be localized in the tumor hypoxic areas and expressed nuclear HIF-2α. The Fzd6 positive cells were found to form more neurospheres, and were highly invasive and therapy resistant, compared to the Fzd6 negative cells, indicating their potential CSC phenotype (Cantilena et al., 2011).
ALDH1 Isoenzymes
Another commonly used marker of stem cells is the subfamily of ALDH1, which is composed of ALDH1A1, ALDH1A2, and ALDH1A3 isoforms, and are known to be involved in RA synthesis (Koppaka et al., 2012). Specifically, ALDH1A2 and ALDH1A3 in NB were found to be overexpressed in a cellular subpopulation of NB, and their roles were further investigated (Flahaut et al., 2016). ALDH1A2 gene was found to be upregulated using microarray analysis of serial sphere passages derived from patient bone marrow metastatic NB cells, playing a critical role on the self-renewal and stemness of these cells (Coulon et al., 2011). Notably, an increase in ALDH1A2 expression in NB tumors was found to be correlated with poorer prognosis (Hartomo et al., 2015).
Similarly, ALDH1A3 expression was also found to be significantly increased in patients with worse outcome, playing a key role in tumor progression (Flahaut et al., 2016). Most importantly, an increase in ALDH activity is directly related to the tumor’s ability to resist conventional drugs and therapies (Abdullah and Chow, 2013; Flahaut et al., 2016).
LGR5 (GPR49)
The stem cell marker LGR5, also known as GPR49, is a receptor of R-spondins which a promote canonical Wnt signaling and act as growth factors for stem cells (de Lau et al., 2014). This stem cell marker was found to be overexpressed in several types of cancers, such as glioblastoma (Nakata et al., 2013), cervical (Cao et al., 2017), breast (Yang et al., 2015) and colorectal cancers (Hirsch et al., 2014; Yanai et al., 2017). In addition, LGR5 was found to be overexpressed in high grade NB (Forgham et al., 2015; Vieira et al., 2017), working upstream of the MEK/ERK and Akt pro-survival signaling pathways (Vieira et al., 2017) which are often triggered in primary NB tumors (Opel et al., 2007).
MMP
Highly malignant NB tumors acquire the ability to reach blood vessels and metastasize by releasing matrix metalloproteinases, commonly known as MMPs, which are enzymes that allow the cell to degrade its surrounding extracellular matrix (Chavali et al., 2014). In this context, MMP expression was found to be upregulated in advanced-stage NB tumors and more specifically the MMP-2 and MMP-9 (or gelatinase enzymes) (Jiang et al., 2011).
TLX
Nuclear orphan receptor TLX (Drosophila tailless homolog) maintains self-renewal capabilities of neural stem/progenitor cells (Shi et al., 2004) via hypoxia-mediated mechanisms (Chavali et al., 2011). In NB, it has been demonstrated that under hypoxic conditions, TLX can activate both MMP-2 and OCT-4 genes, stimulating self-renewal of tumor-spheres and promoting migratory abilities of NB cells (Chavali et al., 2014). In this same study by Chavali et al. (2014) TLX was co-expressed with the migratory neural progenitor markers CD15 and MMP-2 in xenografts of primary NB cells from patients.
ABCG2
ATP-binding cassette sub-family G member 2 is a marker of neural precursors and is one the first universal markers that were used to identify the CSC population in NB tumors (Ding et al., 2010). ABCG2 is a member of the ABC family of transporter proteins, which are ATP-dependent membrane-spanning proteins involved in the transport of substrates into and out of the cell (Vasiliou et al., 2009). The ABCG2 transporter was shown to localize in human neural stem/progenitor cells and was proposed to have a crucial role in the maintenance of these cells in an undifferentiated state (Islam et al., 2005).
In a study by Hirschmann-Jax et al. (2004) primary NB tumor cells showed an elevated expression of ABC transporters including ABCG2, which are responsible for the efflux of therapeutic drugs and therefore for the resistance and survival of these cancer cells. This was further validated by other studies, which showed the role of the ABCG2 transporter in protecting human neural stem/progenitor cells from toxic substances (Islam et al., 2005).
Even though the specific role of ABCG2 in cancer progression is not yet known, this protein was shown to be overexpressed in a variety of cancers and numerous reports support its role in the maintenance and tumorigenicity of CSCs. Thus, this marker could have important therapeutic implications in cancer therapies (Ding et al., 2010).
Nestin
Nestin is an intermediate filament protein that is known to be a marker of neural stem/progenitor cells (Suzuki et al., 2010). It is normally expressed in specific subsets of the mammalian CNS during development, but was also detected in a variety of solid tumors, including NB (Krupkova et al., 2011). A study by Krupkova et al. (2011) demonstrated the localization of the Nestin protein in nuclei of tumor cells. Along with ABCG2, it is also one of the first markers used in the description of CSCs in NB tumors (Garner and Beierle, 2015). The overexpression of Nestin was shown to be linked to the aggressive phenotype of NB tumors (Thomas et al., 2004).
JARID1B
JARID1B, also known as PLU1 or KDM5B, is a H3K4me3 histone lysine demethylase identified as an oncogene that is overexpressed in many cancer types (Li et al., 2011; Chicas et al., 2012; Sayegh et al., 2013). A study has associated between JARID1B and Notch signaling where depletion of JARID1B was found to decrease Notch and its ligand jagged 1 expression, reduce tumor-sphere formation, inhibit invasion, and enhance chemosensitivity to cisplatin (Kuo et al., 2015).
SPDYA
Spy1, a cell cycle regulator encoded by SPDYA gene, plays a role in proliferation, self-renewal and differentiation of human NB cells via regulating CD133+ cell populations and enhancing neurospheres formation in culture. This atypical cyclin-like protein Spy1, recently shown to be driving symmetric division of glioma stem cells, is a critical factor in the stem-like properties of NB CSC populations (Lubanska and Porter, 2014). On the other hand, knockdown of Spy1 causes a decrease in c-MYC expression levels in NB CSCs, a multifunctional transcription factor involved in cellular proliferation (Lubanska and Porter, 2014). In addition, when Spy1 was over-expressed, it hindered the retinoic acid-induced differentiation of NB CSCs. These interesting findings implicate a role for this protein in the regulation of c-MYC protein expression that may be driving tumor-sphere self-renewal and maintenance in NB tumors.
TRPM7
TRPM7, a mechanically regulated TRP channel with kinase activity, harbors a crucial role in maintaining progenitor-like gene expression program in human NB cell lines (Clark et al., 2006; Middelbeek et al., 2012). This protein is essential in embryogenesis and the maintenance of undifferentiated neural crest progenitors (Jin et al., 2008, 2012). In NB, TRPM7 overexpression reduces actomyosin-driven cytoskeletal tension which promotes SNAI2 expression, a neural crest specifier, and controls the malignant features of NB cells (Middelbeek et al., 2015). More recently a study showed that FTY-720 inhibited TRPM7 channel kinase activation and signaling and at low concentrations, sensitized drug-resistant NB cells to antineoplastic drugs (Lange et al., 2017). As such, FTY-720 may serve as a chemo-sensitizing agent when used in combination therapy.
Lamin A/C
Highly proliferative cancer cells have reduced or no expression of Lamin A/C, a major component of the nuclear lamina. This characteristic was verified by enhanced self-renewal of CSCs in vitro and increased ability to initiate tumors in vivo upon down regulation of Lamin A/C in NB cells (Nardella et al., 2015). More recently, Rauschert et al. (2017) reported that reintroducing Lamin A/C in NB reduced cell growth kinetics and impaired migration, invasion and anchorage-independent cell growth and led to cytoskeletal restructuring. Moreover, the introduction of lamin Δ50, known as Progerin, drove these NB cells into senescence.
L1-CAM
L1 cell adhesion molecule known as L1-CAM, is a well-established CSC marker in various tumors including gliomas (Bao et al., 2008) and NB (Rached et al., 2016) and has been shown to confer chemo- and radio-resistance (Held-Feindt et al., 2012; Rached et al., 2016) in these aggressive cancers. Bao et al. (2008) revealed co-segregation of CD133+ and L1-CAM+ glioma cells where CD133+ glioma cells expressed higher levels of L1-CAM compared to CD133- glioma cells. The L1-CAM over-expressing cells also exhibited upregulation of other stem markers including the transcription factor Olig-2. Furthermore, L1-CAM over-expression rendered the cancers highly tumorigenic with increased self-renewal and metastatic potential (Bao et al., 2008). Rached et al. (2016) found that MYCN-amplified NB cell line IMR-32, representing NB with poor prognosis and lack of response to treatment, revealed significant overexpression of L1-CAM compared to non-MYCN-amplified SK-N-SH cells. Moreover, the downregulation of L1-CAM transcription using siRNA transfection showed significant inhibition of proliferation, migration, and tumor sphere formation, hence suggesting a role in tumorigenicity and maintenance of the CSC sub-population.
Neuroblastoma Cancer Stem Cells: from Genes to Therapies
Neuroblastoma CSC Pathways and Proteins That Confer Therapeutic Resistance
Neuroblastoma CSCs have been found to exist in patient primary tumors as well as patient-derived xenograft samples and NB cell lines. This CSC sub-population exhibits high resistance to the currently available therapeutic approaches including chemo-, radio-, and small molecule inhibition therapy (Table 2). These strategies have been successful at eliminating the bulk, non-stem cancer cells, whereas leaving behind the highly aggressive, stem-like TICs.
Wnt/beta-Catenin Signaling and LRG5
One of the most studied pathways in NB affiliated with therapeutic-resistance is the Wnt/Beta-catenin pathway. Vangipuram et al. (2012) reported an over-expression of genes within the Wnt pathway as well as an increased protein expression of β-Catenin and p-GSK3β in CD133+ NB cells compared to CD133- cells. The increased expression of Wnt pathway activity conferred chemoresistance to the cancer stem-like CD133+ cells, while inhibition of Wnt pathway decreased the viability of these cells, ultimately suggesting a protective role for Wnt signaling in the CSC population of NB (Vangipuram et al., 2012). The involvement of Wnt/β-Catenin pathway in inducing therapeutic resistance was further supported by a recent study which showed pro-apoptotic and anti-proliferative effects in an in vivo NB model after GSK3 inhibition (Kunnimalaiyaan et al., 2018). This mechanism has also been suggested in other cancers including Wilms’ tumor (Pode-Shakked et al., 2011), colon cancer (Tenbaum et al., 2012), breast cancers (Hallett et al., 2012), pancreatic cancer (Cui et al., 2012) and glioblastoma (Kim et al., 2012).
Leucine-rich repeat-containing G-protein coupled receptors (LRGs) such as LRG5 is important for maintaining Wnt signaling by preventing its ubiquitination and degradation (Hao et al., 2012). Tumor samples from high-risk NB patients not only showed over-expression of Wnt signaling pathway, but also up-regulation of LRG5 mRNA when grown as tumor-spheres. These tumor-spheres exhibited high expression of Wnt target genes and higher in vivo tumorigenicity (Coulon et al., 2011). Moreover, Vieira et al. (2017) demonstrated the important role of LRG5 in regulating NB survival and proliferation via the MEK/ERK-Wnt/β-Catenin signaling pathways. In fact, MEK/ERK activity has been implicated in the maintenance of embryonic stem cells in the undifferentiated state as well as CSCs in glioma (Kwon et al., 2017), breast cancer (Baker et al., 2018), thyroid cancer (Wang K. et al., 2018), lung cancer (Ning et al., 2018), gastric cancer (Ning et al., 2018), glioblastoma (Wang F. et al., 2018) and rhabdomyosarcoma (Ciccarelli et al., 2016).
Considering the therapeutic implications of these findings, it is tempting to assume that multiple targeting of various tumorigenic pathways may prove beneficial in sensitizing the cancers by eradicating the CSCs within them. In fact, combined targeting of the Wnt signaling with chemotherapy further sensitized the malignant NB cell lines to the treatment, by inducing differentiation. The target genes of the inhibitor, identified by microarray analysis, included p21, p53, ubiquitin C, ZBED8, MDM2, CASP3, and FZD1 (Suebsoonthron et al., 2017), thereby explaining the enhanced sensitivity of the malignant NB cell lines to chemotherapy after Wnt signaling inhibition.
ABC Transporters
While therapeutic resistance in NB CSCs may be due to several cellular mechanisms, it is likely to be driven by the common MDR mechanism, which is mediated by the ATP-Binding Cassettes (ABC) drug efflux transporters such as MDR1, several MRP and BCRP (Gottesman et al., 2002). Various studies have implicated the role of ABC drug efflux transporters in therapeutic resistance of CSCs.
A recent study reported an increased sensitivity of NB stem cells to combined treatment of the antineoplastic drug (cisplatin) with transporter inhibitor (probenecid) (Campos-Arroyo et al., 2016). The combined treatment led to reduced colony forming capacity of the CSCs, increased apoptosis and decreased proliferation. The authors also reported enhanced caspase-3 activity and significant down-regulation of both mRNA and protein expression of MDR1, MRP2 and BCRP (Campos-Arroyo et al., 2016); therefore, suggesting that the transcriptional inhibition of these proteins prevented drug efflux and allowed the drug to remain longer within the CSCs, ultimately inducing the observed apoptotic effect. Moreover, probenecid decreased the side population of the NB CSCs and reduced the percentage of stem marker CD133 as well as EMT markers vimentin and snail (Campos-Arroyo et al., 2016). In brief, if CSCs are forced back into a “non-stem state” by inducing their differentiation or preventing their EMT progression, therapeutic resistance subsides.
Another study by Vella et al. (2015) demonstrated a reduced growth rate of NB nodules and an increased overall survival of the mice in an animal model treated with chemotherapy (cisplatin) combined with a drug that induces NB differentiation (perhexiline). The pharmacologic induction of NDM29 induced NB differentiation and decreased the protein expression of ABC transporters, specifically in TICs/CSCs thereby increasing the chemotherapeutic sensitivity of this malignant, treatment-evasive NB sub-population.
Other studies have also reported similar findings with NB CD133+ cells exhibiting up-regulated expression of the ABC transporters and leading to their therapeutic resistance. The possible mechanism by which the CD133+ cells resist the chemo-therapy may be via up-regulation of ABC transporters that efflux the drug out of the cells (Al-Dimassi et al., 2014), thereby hindering the drugs from inducing their apoptotic effects on the CSCs.
Signaling Cascades Involved in Tumorigenesis
AMPK
The AMP-activated protein kinase pathway has been affiliated with driving the malignant behaviors of many tumors including brain cancers (Rehman et al., 2014; Li et al., 2017). Drugs that target this pathway have been investigated to elucidate their role in eliminating cancerous growth. Stem-like TICs within the bulk of tumors have been found to respond to such therapies. A study by Mouhieddine et al. (2015) demonstrated that Metformin, an AMPK activator and Ara-a, an AMPK pathway inhibitor both significantly reduced NB and glioma CSC proliferation and survival in a 2D and 3D tumor-sphere model. The importance of this finding lies in the fact that while the two drugs work antagonistically to each other, their anticancer effects may be due to the inhibitory effects on the mTOR pathway and Akt achieved with Metformin, and the energy deprivation achieved with Ara-a-induced inhibition of AMPK, thereby starving the CSCs. Furthermore, being a nucleoside analog, Ara-a may induce anti-cancer effects by interfering with DNA synthesis in these cells. In fact, the authors report the higher potency of Ara-a on these cancers (Mouhieddine et al., 2015).
MAPK and PI3K/mTOR
Various studies have demonstrated the efficacy of mTOR pathway inhibition on reducing tumor growth and specifically inhibiting proliferation of CSCs. Smith et al. (2010) reported that rapamycin, an mTOR inhibitor, successfully decreased cell viability and proliferation in an in vitro, NB patient-derived CSCs model. This activity was mediated by dephosphorylation of mTOR downstream targets p70S6K and S6RP. We further validated this finding in a recent study from our lab, where triciribine and rapamycin were used to study the role of inhibiting two different points of the Akt/mTOR pathway in vitro on U251 (glioblastoma) and SH-SY5Y (NB) human cell lines and their CSCs (Bahmad et al., 2018). Both drugs decreased the SFU of glioblastoma and NB cells thus extinguishing their CSC populations (Bahmad et al., 2018). On the other hand, apoptotic markers, such as cleaved PARP and increased sub-2n DNA were observed after rapamycin treatment in NB CSCs (Smith et al., 2010). In addition, it has been reported that NB CSCs infected with mTOR shRNA demonstrated 70–80% growth inhibition compared to mock-infected NB CSCs. Daily in vivo rapamycin treatment of mice bearing NB tumors successfully reduced tumor weight by >70% compared to only 43.4% achieved with vinblastine treatment, the standard chemotherapeutic drug used for NB. Tumor-sphere formation in the NB CSCs was also reported to be significantly (7.75-fold) inhibited after rapamycin treatment compared to vehicle-treated CSCs, thereby implicating the CSC-targeting ability of rapamycin (Smith et al., 2010).
As previously well established, P53 functions to suppress mTOR pathway under stressful conditions in cells. When cells undergo stress, the functional P53 protein triggers transcription of proteins that negatively regulate the IGF/AKT/mTOR pathway to induce cell-cycle arrest, DNA repair, senescence or apoptosis, depending on the stressor and tumor type (Moreno-Smith et al., 2017). As such, combination therapy that stabilizes P53 expression along with mTOR inhibitors may prove more efficacious than single therapy that often leads to resistance. Moreno-Smith et al. (2017) reported on the enhanced effects of temsirolimus (mTOR inhibitor) when combined with P53 activators (Nutlin 3a, a first-generation and RG7388 a second-generation MDM2 inhibitor) in an in vivo model of NB. The combined therapy enhanced the anti-proliferative and pro-apoptotic effects of single-agent therapy, implicating the importance of P53 stabilization in inducing mTOR inhibition and subsequent cellular apoptosis. This was further tested in an orthotopic, in vivo pre-clinical model using both MYCN-amplified and non-amplified cell lines. In both cell lines used, the combination therapy was significantly more potent in anti-tumor activity compared to either monotherapy. More importantly, the combination therapy led to long-term cessation of tumor growth after treatment withdrawal by induced apoptosis (Moreno-Smith et al., 2017).
Hypoxia and Retinoic Acid
The common link between mTOR, PI3K and insulin-like growth factor (IGF) in NB is hypoxia (Påhlman and Mohlin, 2018). In fact, hypoxic regions of NB have been reported to express the hypoxia-inducible factors 1 and 2 (HIF1 and HIF2), which coincidently, confer stem-like features in these cells, including immature, neural-crest like cells with self-renewal potential. In addition, HIF2 and IGF co-expression is correlated in clinical NB specimens, and IGF regulates hypoxic expression of HIF2 (Påhlman and Mohlin, 2018). Moreover, IGF receptor binding induces PI3K signaling which subsequently drives tumorigenesis such as cancerous growth, survival and differentiation. PI3K and mTOR activation by growth factors also initiates HIF1 translation, therefore the PI3K/mTOR pathways are putative candidate mediators of IGF-driven HIF expression and activity in NB and CSC maintenance and proliferation (Påhlman and Mohlin, 2018).
Inducing differentiation of NB CSCs may render them more sensitive to therapeutic intervention. Retinoic acid is clinically used to induce cancer differentiation in patients that have undergone induction and consolidation chemotherapy for high-risk NB (Duffy et al., 2017). RA (13-cis-retinoic acid) is a well-known differentiating agent reported to reduce stemness characteristics in various tumors including NB (Craig et al., 2016). Cimmino et al. (2015) demonstrated that HIF1A silencing combined with ATRA treatment led to differentiation and senescence of NB cells into a more benign, glial lineage that would render them therapeutically responsive.
Interestingly, combined RA treatment with proteasome inhibition using MG132 led to growth arrest and differentiation, inhibition of tumor-sphere formation and apoptosis in NB CSCs. Stem markers Nestin, Sox2, and Oct-4 were all reduced with the combination therapy as compared to monotherapy (Hämmerle et al., 2013), exemplifying the importance of differentiation inducers used together with other agents to enhance the anti-CSC effect in the malignant, sub-population of these NB tumors.
Epigenetics
The vast majority of NB tumors in children arise due to somatic mutations, as opposed to germline mutations of the anaplastic lymphoma kinase (ALK) gene in familial neuroblastoma, accounting for 2% of the cases (Mosse et al., 2008). Nonetheless, epigenetic regulation has been the suspected culprit believed to play a critical role driving these malignancies. The heterogeneity of NB tumors is therefore believed to be driven by a combination of somatic mutations and epigenetically-regulated factors. In fact, epigenetic modifications have been reported to induce a stem-like cancer cell in NB (Muñoz et al., 2012; Kobayashi et al., 2013; Toh et al., 2017). Specifically, the epigenetically-induced NB CSCs exhibited high expression of stemness factors and stem cell markers, they had open chromatin structure, increased tumor-initiating ability and metastatic potential. Moreover, they had a highly undifferentiated histological appearance with over-expression of MYC/MYCN (Ikegaki et al., 2013).
MYCN has been shown to interact with epigenetic machinery (He et al., 2013) and Duffy et al. (2017) reported that epigenetic regulators, including HDACs and BRD4, were differentially activated in MYCN-amplified NB, affecting the RA-induced differentiation in these cells. The authors reported that RA inhibits HDAC functioning while MYCN overexpression activates it. An important regulator identified in this study was TGFB1, a ligand for TGF-β signaling pathway. Pharmacological activation of the TGF-β signaling pathway using KGN, a small molecule that indirectly enhances TGF-β signaling by regulating the activity of SMAD transcriptional effectors, was used in combination with RA to determine the effect of this dual therapy on NB differentiation and malignancy. Both compounds used as sole therapy yielded a modest differentiation response, but in combination, exerted a more potent response and reduced viability in the MYCN-amplified NB cells (Duffy et al., 2017), thereby signifying the importance of using combination therapy in MYCN-amplified, high-risk NB patients to reach better clinical outcomes.
Others have also shown that HDAC inhibition via vorinostat in MYCN-amplified NB cells reduced chemo-resistance (DOX response) and in vitro invasive potential, inhibited tumor-sphere formation and down-regulated stem marker expression in NB CSCs (Zheng et al., 2013). And yet another group reported on the synergistic effect of combining RA with the HDAC inhibitor CBHA in an in vivo, human xenograft NB model (Coffey et al., 2001). This was achieved via the inhibition of AKT signaling pathway and survivin (Shah et al., 2013), whose expression usually promote tumor cell proliferation and inhibit apoptosis.
Moreover, combined therapy with HDAC inhibitors and ATRA down-regulated c-Myc, the neuronal markers NeuN and β-3 tubulin, as well as the oncoprotein BMI1, and more potently reduced cellular proliferation in NB cell lines (Almeida et al., 2017). Knowing that BMI1 is a potent stem cell maintenance oncoprotein, this combination therapy may prove effective due to eliminating the CSC sub-population in the aggressive form of NB. As previously reported, BMI1 is capable of repressing P53 responses in NB precursors leading to NB initiation (Calao et al., 2012). Furthermore, BMI1 can directly bind to p53 in a complex with other Polycomb complex proteins, Ring1A or Ring1B, leading to increased p53 ubiquitination and degradation. Another study revealed that BMI1 expression positively correlated with a nucleotide binding protein BORIS/CTCFL, found to be aberrantly expressed in various malignancies and primarily re-expressed in CSCs (Garikapati et al., 2017). The authors of this study demonstrated an important cross-talk between BORIS/CTCFL and Wnt/β-catenin signaling pathways that led to enhanced expression of stemness proteins and increased EMT markers such as N-CAD, Vimentin and SNAIL. This intricate network of communication between BMI1, BORIS/CTCFL and Wnt signaling plays a crucial role that confers therapeutic resistance and malignancy in the aggressive MYCN-amplified NB cell line.
Delta-Like 1
Another important player in hypoxia-induced cancer stemness, as mentioned above, is the DLK1, which has been shown to be up-regulated after hypoxia induction in neuronal tumors (Kim et al., 2009). Its protein up-regulation enhances tumor stemness and tumorigenic growth in vivo, whereas its silencing drives cellular differentiation and reduces the tumorigenic potential in NB CSCs (Begum et al., 2014).
The combined treatment of NB cells with RA and DLK1 knock-down led to significantly higher levels of cellular differentiation compared to monotherapy with either agent. Interestingly, the DLK1 effects seem to be mediated via prohibition PHB1 and PHB2 interactions which have previously been reported to play roles in cellular proliferation, apoptosis, transcription, mitochondrial folding and cell signaling (Theiss and Sitaraman, 2011). The authors in Begum et al. (2014) demonstrated that the interaction between DLK1 and both PHB1 and PHB2 via its cytoplasmic domain, bring about NB cell stemness and tumor-sphere self-renewal. They showed that knock-down of either PHB or DLK1 reduces the clonogenic and self-renewal potential of NB CSCs. This interaction was a novel finding and thus, an important avenue to explore in multi-targeted approaches aimed at eliminating the CSCs within the bulk of the tumor along with the differentiated, non-stem cancer cells.
G-CSF/STAT3
Granulocyte-colony stimulation factor receptor positive or CD114+ NB cells have stem-like characteristics that render them highly tumorigenic, self-renewing, with a treatment evasive phenotype (Hsu et al., 2013). Acting via STAT3 stimulation and up-regulation, G-CSF presents itself as a very attractive target for NB CSC elimination and for increasing the potential long-term cancer-free survival in children. In fact, Agarwal et al. (2015) demonstrated that anti-G-CSF antibody or STAT3 inhibition led to depletion of CSC subpopulation within tumors which was correlated with tumor growth inhibition, decreased metastasis, and increased chemo-sensitivity in a pre-clinical NB animal model. Such an observation warrants the consideration of a multi-modality therapeutic approach that targets the G-CSF-STAT3 signaling in the NB CSCs together with the standard chemotherapeutic drugs in order to completely eradicate the high-risk NB.
Gheeya et al. (2009) had previously demonstrated that STAT3 inhibition using Cucurbitacin I induced an antitumor effect in various solid tumors including NB with and without MYCN-amplification. More recently, Honokiol, a biphenolic natural product isolated from the bark and leaves of Magnolia plant, and an inhibitor of the STAT3 signaling pathway was reported to inhibit cancerous growth in various tumors including NB (Prasad and Katiyar, 2016). Yet another natural inhibitor of STAT3 called curcumin or turmeric (diferuloylmethane), also known as an inhibitor of Akt, COX2 and NF-κB signaling, demonstrated anti-cancer properties which rendered it a chemo-sensitizing agent against various tumors including glioma, NB, cervical carcinoma, epidermal carcinoma, prostate cancer, and colon cancer (Goel and Aggarwal, 2010).
L1-CAM
One of the prominent players in driving tumorigenic invasion and motility is L1-CAM (Zaatiti et al., 2018) whose suppression was explored as a therapeutic strategy in various tumors. Bao et al. (2008) reported that shRNA knock-down of L1-CAM in CD133+ glioma cells significantly reduced neurosphere self-renewal potential, increased apoptosis, but inhibited proliferation of CSCs. L1-CAM silencing suppressed tumor growth and increased survival in an in vivo xenograft mouse model (Bao et al., 2008). This silencing was associated with reduced expression of Olig2, but increased expression of the p21 (WAF1/CIP1) tumor suppressor, suggesting their possible involvement in the CD133+ driven mechanistic effects in glioma cells.
The CE7 epitope of L1-CAM (CD171) has been explored in the context of CAR-T therapy in NB tumors (Hong et al., 2014; Kunkele et al., 2017) and other cancers, including lung, ovarian (Hong et al., 2016), and renal carcinomas as well as glioblastomas (Hong et al., 2014). One study concluded that CE7 epitope of L1-CAM may be amenable to CAR-T targeted therapy and thus serves as an invaluable tool in adoptive immunotherapy (Hong et al., 2014). Moreover, in a more recent pre-clinical assessment, (Kunkele et al. (2017) tested the safety of targeting the CE7 epitope CD171 with CE7-CAR T cells and determined whether bioactive CAR-T cells may be generated from heavily pretreated NB patients with recurrent or refractory disease. Fortunately, the authors confirmed the efficacy and safety of the CE7 epitope on CD171 to be used as a CAR-T cell target for NB patients with recurrent/refractory disease (Kunkele et al., 2017).
Polo-Like Kinase 1 (PLK1)
Neuroblastoma CSCs have been previously reported to highly express the serine/threonine kinase, Polo-like kinase 1, a known inducer of G2/M-phase transition. Grinshtein et al. (2011) demonstrated that the PLK1 inhibitor, BI 2536 significantly reduced tumor growth in an in vivo xenograft therapeutic model of NB CSCs when administered alone or in combination with irinotecan, which is typically used in patients with refractory NB.
More recently, Pajtler et al. (2017) investigated the tumorigenic effects of a PLK1 competitive inhibitor, GSK461364, in both in vitro and in vivo NB models. This treatment led to significant reduction in cell viability and proliferation and it also induced cell cycle arrest and apoptosis. In addition, the growth of established xenograft tumors in nude mice was significantly delayed, whereas survival time in the treatment group significantly increased (Pajtler et al., 2017). This further highlights the importance of targeting the CSC drivers in an attempt to achieve better anti-tumor effects of the currently used therapeutic agents.
Conclusion and Future Directions
A pressing challenge of nervous system tumors in general and NB, specifically, is to overcome the therapy-resistant nature of CSCs. The eminent role they play in aggravating chemoresistance and malignancy magnifies the need to identify (1) the molecular signatures and the genetic aberrations of CSCs in neuroblastoma and (2) the therapeutic intervention that has been used in targeting the stem cell dysregulations. Even though a unique set of CSC markers have not been identified yet, a group of molecules have been associated with a stem-like behavior in cells expressing them, on the molecular, cellular and functional levels. It is foreseeable that targeting the key players that confer stemness to CSCs can substantially eliminate the CSC-like phenotypes. Moreover, combination of multiple targeting strategies provides a potential therapeutic anti-cancer intervention that needs to be further tested and studied. In the field of neuroblastoma, the ongoing efforts in eradicating CSCs may allow for promoting the survival of the children in ways comparable to the progress seen with some leukemias (He et al., 2015; Al-Hussaini et al., 2016; Ruella et al., 2016; Desai et al., 2019).
Author Contributions
All authors worked on study conception and design, analyzed and interpreted the data, drafted the manuscript, and read and approved the final draft. HFB and WA-K screened titles for relevance and abstracted the data from the eligible full text articles. TA-A and WA-K critically revised the manuscript with input from the entire team.
Conflict of Interest Statement
The authors declare that the research was conducted in the absence of any commercial or financial relationships that could be construed as a potential conflict of interest.
Acknowledgments
We would like to thank all members in WA-K Laboratory (The WAK Lab) and TA-A Laboratory for their help on this work.
Abbreviations
ABC, adenosine triphosphate-binding cassette; ABCG2, ATP-binding cassette sub-family G member 2; ALDH1, aldehyde dehydrogenases 1; ATRA, all-trans retinoic acid; BCRP, breast cancer resistance protein; CBHA, carboxycinnamic acid bis-hydroxamide; CSC, cancer stem cell; DLK1, Delta-like 1; EMT, epithelial-to-mesenchymal transition; Fzd6, frizzled receptors 6; G-CSFR, Granulocyte-Colony Stimulation Factor Receptor; HIF, hypoxia-inducible factor; KGN, kartogenin; MDR, multidrug resistance; MDR1, P-glycoprotein; MMP, matrix metalloproteinase; MRP, multidrug resistance proteins; NB, neuroblastoma; NDM29, neuroblastoma differentiation marker 29; PHB1, prohibitin 1; PHB2, prohibitin 2; PLK1, Polo-Like Kinase 1; RA, retinoic acid; RAP, reversible adaptive plasticity; S6RP, S6 ribosomal protein; SFU, sphere-forming units; STAT3, Signal Transducer and Activator of Transcription 3; TIC, tumor-initiating cell.
References
Abdullah, L. N., and Chow, E. K.-H. (2013). Mechanisms of chemoresistance in cancer stem cells. Clin. Transl. Med. 2:3. doi: 10.1186/2001-1326-2-3
Agarwal, S., Lakoma, A., Chen, Z., Hicks, J., Metelitsa, L. S., Kim, E. S., et al. (2015). G-CSF promotes neuroblastoma tumorigenicity and metastasis via STAT3-dependent cancer stem cell activation. Cancer Res. 75, 2566–2579. doi: 10.1158/0008-5472.can-14-2946
Akashi, T., Shirasawa, T., and Hirokawa, K. (1994). Gene expression of CD24 core polypeptide molecule in normal rat tissues and human tumor cell lines. Virchows Arch. 425, 399–406.
Al-Dimassi, S., Abou-Antoun, T., and El-Sibai, M. (2014). Cancer cell resistance mechanisms: a mini review. Clin. Transl. Oncol. 16, 511–516. doi: 10.1007/s12094-014-1162-1
Al-Hussaini, M., Rettig, M. P., Ritchey, J. K., Karpova, D., Uy, G. L., Eissenberg, L. G., et al. (2016). Targeting CD123 in acute myeloid leukemia using a T-cell-directed dual-affinity retargeting platform. Blood 127, 122–131. doi: 10.1182/blood-2014-05-575704
Almeida, V. R., Vieira, I. A., Buendia, M., Brunetto, A. T., Gregianin, L. J., Brunetto, A. L., et al. (2017). Combined treatments with a retinoid receptor agonist and epigenetic modulators in human neuroblastoma cells. Mol. Neurobiol. 54, 7610–7619. doi: 10.1007/s12035-016-0250-3
Ayla, S., Bilir, A., Soner, B. C., Yilmaz-Dilsiz, O., Erguven, M., and Oktem, G. (2014). Notch signaling-related therapeutic strategies with novel drugs in neuroblastoma spheroids. J. Pediatr. Hematol. Oncol. 36, 37–44. doi: 10.1097/MPH.0b013e3182755c73
Bahmad, H. F., Mouhieddine, T. H., Chalhoub, R. M., Assi, S., Araji, T., Chamaa, F., et al. (2018). The Akt/mTOR pathway in cancer stem/progenitor cells is a potential therapeutic target for glioblastoma and neuroblastoma. Oncotarget 9, 33549–33561. doi: 10.18632/oncotarget.26088
Baker, A., Wyatt, D., Bocchetta, M., Li, J., Filipovic, A., Green, A., et al. (2018). Notch-1-PTEN-ERK1/2 signaling axis promotes HER2+ breast cancer cell proliferation and stem cell survival. Oncogene 37, 4489–4504. doi: 10.1038/s41388-018-0251-y
Bao, S., Wu, Q., Li, Z., Sathornsumetee, S., Wang, H., Mclendon, R. E., et al. (2008). Targeting cancer stem cells through L1CAM suppresses glioma growth. Cancer Res. 68, 6043–6048. doi: 10.1158/0008-5472.can-08-1079
Begum, A., Lin, Q., Yu, C., Kim, Y., and Yun, Z. (2014). Interaction of delta-like 1 homolog (Drosophila) with prohibitins and its impact on tumor cell clonogenicity. Mol. Cancer Res. 12, 155–164. doi: 10.1158/1541-7786.mcr-13-0360
Bhaskara, V. K., Mohanam, I., Rao, J. S., and Mohanam, S. (2012). Intermittent hypoxia regulates stem-like characteristics and differentiation of neuroblastoma cells. PLoS One 7:e30905. doi: 10.1371/journal.pone.0030905
Bilir, A., Erguven, M., Yazihan, N., Aktas, E., Oktem, G., and Sabanci, A. (2010). Enhancement of vinorelbine-induced cytotoxicity and apoptosis by clomipramine and lithium chloride in human neuroblastoma cancer cell line SH-SY5Y. J. Neurooncol. 100, 385–395. doi: 10.1007/s11060-010-0209-6
Buhagiar, A., and Ayers, D. (2015). Chemoresistance, cancer stem cells, and miRNA influences: the case for neuroblastoma. Anal. Cell. Pathol. 2015:150634. doi: 10.1155/2015/150634
Calao, M., Sekyere, E. O., Cui, H. J., Cheung, B. B., Thomas, W. D., Keating, J., et al. (2012). Direct effects of Bmi1 on p53 protein stability inactivates oncoprotein stress responses in embryonal cancer precursor cells at tumor initiation. Oncogene 32, 3616–3626. doi: 10.1038/onc.2012.368
Campos-Arroyo, D., Maldonado, V., Bahena, I., Quintanar, V., Patino, N., Carlos Martinez-Lazcano, J., et al. (2016). Probenecid sensitizes neuroblastoma cancer stem cells to cisplatin. Cancer Invest. 34, 155–166. doi: 10.3109/07357907.2016.1139717
Cantilena, S., Pastorino, F., Pezzolo, A., Chayka, O., Pistoia, V., Ponzoni, M., et al. (2011). Frizzled receptor 6 marks rare, highly tumourigenic stem-like cells in mouse and human neuroblastomas. Oncotarget 2, 976–983. doi: 10.18632/oncotarget.410
Cao, H.-Z., Liu, X.-F., Yang, W.-T., Chen, Q., and Zheng, P.-S. (2017). LGR5 promotes cancer stem cell traits and chemoresistance in cervical cancer. Cell Death Dis. 8:e3039. doi: 10.1038/cddis.2017.393
Carpentieri, A., Cozzoli, E., Scimeca, M., Bonanno, E., Sardanelli, A. M., and Gambacurta, A. (2016). Differentiation of human neuroblastoma cells toward the osteogenic lineage by mTOR inhibitor. Cell Death Dis. 7:e2202. doi: 10.1038/cddis.2016.60
Castelo-Branco, P., Zhang, C., Lipman, T., Fujitani, M., Hansford, L., Clarke, I., et al. (2011). Neural tumor-initiating cells have distinct telomere maintenance and can be safely targeted for telomerase inhibition. Clin. Cancer Res. 17, 111–121. doi: 10.1158/1078-0432.ccr-10-2075
Chakrabarti, L., Abou-Antoun, T., Vukmanovic, S., and Sandler, A. D. (2012). Reversible adaptive plasticity: a mechanism for neuroblastoma cell heterogeneity and chemo-resistance. Front. Oncol. 2:82. doi: 10.3389/fonc.2012.00082
Chavali, P. L., Saini, R. K., Matsumoto, Y., Agren, H., and Funa, K. (2011). Nuclear orphan receptor TLX induces Oct-3/4 for the survival and maintenance of adult hippocampal progenitors upon hypoxia. J. Biol. Chem. 286, 9393–9404. doi: 10.1074/jbc.M110.167445
Chavali, P. L., Saini, R. K., Zhai, Q., Vizlin-Hodzic, D., Venkatabalasubramanian, S., Hayashi, A., et al. (2014). TLX activates MMP-2, promotes self-renewal of tumor spheres in neuroblastoma and correlates with poor patient survival. Cell Death Dis. 5:e1502. doi: 10.1038/cddis.2014.449
Chen, X., Ye, S., and Ying, Q. L. (2015). Stem cell maintenance by manipulating signaling pathways: past, current and future. BMB Rep. 48, 668–676.
Chicas, A., Kapoor, A., Wang, X., Aksoy, O., Evertts, A. G., Zhang, M. Q., et al. (2012). H3K4 demethylation by Jarid1a and Jarid1b contributes to retinoblastoma-mediated gene silencing during cellular senescence. Proc. Natl. Acad. Sci. U.S.A. 109, 8971–8976. doi: 10.1073/pnas.1119836109
Choi, S. K., Kim, J. H., Park, J. K., Lee, K. M., Kim, E., and Jeon, W. B. (2013). Cytotoxicity and inhibition of intercellular interaction in N2a neurospheroids by perfluorooctanoic acid and perfluorooctanesulfonic acid. Food Chem. Toxicol. 60, 520–529. doi: 10.1016/j.fct.2013.07.070
Ciccarelli, C., Vulcano, F., Milazzo, L., Gravina, G. L., Marampon, F., Macioce, G., et al. (2016). Key role of MEK/ERK pathway in sustaining tumorigenicity and in vitro radioresistance of embryonal rhabdomyosarcoma stem-like cell population. Mol. Cancer 15:16. doi: 10.1186/s12943-016-0501-y
Ciccarone, V., Spengler, B. A., Meyers, M. B., Biedler, J. L., and Ross, R. A. (1989). Phenotypic diversification in human neuroblastoma cells: expression of distinct neural crest lineages. Cancer Res. 49, 219–225.
Cimmino, F., Pezone, L., Avitabile, M., Acierno, G., Andolfo, I., Capasso, M., et al. (2015). Inhibition of hypoxia inducible factors combined with all-trans retinoic acid treatment enhances glial transdifferentiation of neuroblastoma cells. Sci. Rep. 5:11158. doi: 10.1038/srep11158
Clark, K., Langeslag, M., Van Leeuwen, B., Ran, L., Ryazanov, A. G., Figdor, C. G., et al. (2006). TRPM7, a novel regulator of actomyosin contractility and cell adhesion. EMBO J. 25, 290–301. doi: 10.1038/sj.emboj.7600931
Coffey, D. C., Kutko, M. C., Glick, R. D., Butler, L. M., Heller, G., Rifkind, R. A., et al. (2001). The histone deacetylase inhibitor, CBHA, inhibits growth of human neuroblastoma xenografts in vivo, alone and synergistically with all-trans retinoic acid. Cancer Res. 61, 3591–3594.
Coulon, A., Flahaut, M., Muhlethaler-Mottet, A., Meier, R., Liberman, J., Balmas-Bourloud, K., et al. (2011). Functional sphere profiling reveals the complexity of neuroblastoma tumor-initiating cell model. Neoplasia 13, 991–1004.
Cournoyer, S., Nyalendo, C., Addioui, A., Belounis, A., Beaunoyer, M., Aumont, A., et al. (2012). Genotype analysis of tumor-initiating cells expressing CD133 in neuroblastoma. Genes Chromosomes Cancer 51, 792–804. doi: 10.1002/gcc.21964
Craig, B. T., Rellinger, E. J., Alvarez, A. L., Dusek, H. L., Qiao, J., and Chung, D. H. (2016). Induced differentiation inhibits sphere formation in neuroblastoma. Biochem. Biophys. Res. Commun. 477, 255–259. doi: 10.1016/j.bbrc.2016.06.053
Cui, H., Hu, B., Li, T., Ma, J., Alam, G., Gunning, W. T., et al. (2007). Bmi-1 is essential for the tumorigenicity of neuroblastoma cells. Am. J. Pathol. 170, 1370–1378. doi: 10.2353/ajpath.2007.060754
Cui, J., Jiang, W., Wang, S., Wang, L., and Xie, K. (2012). Role of Wnt/beta-catenin signaling in drug resistance of pancreatic cancer. Curr. Pharm. Des. 18, 2464–2471.
Daley, G. Q. (2015). Stem cells and the evolving notion of cellular identity. Philos. Trans. R. Soc. Lond. B Biol. Sci. 370:20140376. doi: 10.1098/rstb.2014.0376
Das, B., Tsuchida, R., Malkin, D., Koren, G., Baruchel, S., and Yeger, H. (2008). Hypoxia enhances tumor stemness by increasing the invasive and tumorigenic side population fraction. Stem Cells 26, 1818–1830. doi: 10.1634/stemcells.2007-0724
Dawood, S., Austin, L., and Cristofanilli, M. (2014). Cancer stem cells: implications for cancer therapy. Oncology 28, 1101–1107, 1110.
de Lau, W., Peng, W. C., Gros, P., and Clevers, H. (2014). The R-spondin/Lgr5/Rnf43 module: regulator of Wnt signal strength. Genes Dev. 28, 305–316. doi: 10.1101/gad.235473.113
Desai, A., Yan, Y., and Gerson, S. L. (2019). Concise reviews: cancer stem cell targeted therapies: toward clinical success. Stem Cells Transl. Med. 8, 75–81. doi: 10.1002/sctm.18-0123
Diaz-Carballo, D., Acikelli, A. H., Bardenheuer, W., Gustmann, S., Malak, S., Stoll, R., et al. (2014). Identification of compounds that selectively target highly chemotherapy refractory neuroblastoma cancer stem cells. Int. J. Clin. Pharmacol. Ther. 52, 787–801. doi: 10.5414/cp202154
Ding, X. W., Wu, J. H., and Jiang, C. P. (2010). ABCG2: a potential marker of stem cells and novel target in stem cell and cancer therapy. Life Sci. 86, 631–637. doi: 10.1016/j.lfs.2010.02.012
Duffy, D. J., Krstic, A., Halasz, M., Schwarzl, T., Konietzny, A., Iljin, K., et al. (2017). Retinoic acid and TGF-β signalling cooperate to overcome MYCN-induced retinoid resistance. Genome Med. 9:15. doi: 10.1186/s13073-017-0407-3
Farace, C., Oliver, J. A., Melguizo, C., Alvarez, P., Bandiera, P., Rama, A. R., et al. (2015). Microenvironmental modulation of decorin and lumican in temozolomide-resistant glioblastoma and neuroblastoma cancer stem-like cells. PLoS One 10:e0134111. doi: 10.1371/journal.pone.0134111
Flahaut, M., Jauquier, N., Chevalier, N., Nardou, K., Balmas Bourloud, K., Joseph, J. M., et al. (2016). Aldehyde dehydrogenase activity plays a Key role in the aggressive phenotype of neuroblastoma. BMC Cancer 16:781. doi: 10.1186/s12885-016-2820-1
Forgham, H., Johnson, D., Carter, N., Veuger, S., and Carr-Wilkinson, J. (2015). Stem cell markers in neuroblastoma-an emerging role for LGR5. Front. Cell Dev. Biol. 3:77. doi: 10.3389/fcell.2015.00077
Garikapati, K. R., Patel, N., Makani, V. K. K., Cilamkoti, P., Bhadra, U., and Bhadra, M. P. (2017). Down-regulation of BORIS/CTCFL efficiently regulates cancer stemness and metastasis in MYCN amplified neuroblastoma cell line by modulating Wnt/beta-catenin signaling pathway. Biochem. Biophys. Res. Commun. 484, 93–99. doi: 10.1016/j.bbrc.2017.01.066
Garner, E. F., and Beierle, E. A. (2015). Cancer stem cells and their interaction with the tumor microenvironment in neuroblastoma. Cancers 8:E5.
Gheeya, J. S., Chen, Q. R., Benjamin, C. D., Cheuk, A. T., Tsang, P., Chung, J. Y., et al. (2009). Screening a panel of drugs with diverse mechanisms of action yields potential therapeutic agents against neuroblastoma. Cancer Biol. Ther. 8, 2386–2395.
Goel, A., and Aggarwal, B. B. (2010). Curcumin, the golden spice from Indian saffron, is a chemosensitizer and radiosensitizer for tumors and chemoprotector and radioprotector for normal organs. Nutr. Cancer 62, 919–930. doi: 10.1080/01635581.2010.509835
Gottesman, M. M., Fojo, T., and Bates, S. E. (2002). Multidrug resistance in cancer: role of ATP-dependent transporters. Nat. Rev. Cancer 2, 48–58. doi: 10.1038/nrc706
Grinshtein, N., Datti, A., Fujitani, M., Uehling, D., Prakesch, M., Isaac, M., et al. (2011). Small molecule kinase inhibitor screen identifies polo-like kinase 1 as a target for neuroblastoma tumor-initiating cells. Cancer Res. 71, 1385–1395. doi: 10.1158/0008-5472.can-10-2484
Gudjonsson, T., and Magnusson, M. K. (2005). Stem cell biology and the cellular pathways of carcinogenesis. APMIS 113, 922–929. doi: 10.1111/j.1600-0463.2005.apm_371.x
Hallett, R. M., Kondratyev, M. K., Giacomelli, A. O., Nixon, A. M., Girgis-Gabardo, A., Ilieva, D., et al. (2012). Small molecule antagonists of the Wnt/beta-catenin signaling pathway target breast tumor-initiating cells in a Her2/Neu mouse model of breast cancer. PLoS One 7:e33976. doi: 10.1371/journal.pone.0033976
Hämmerle, B., Yañez, Y., Palanca, S., Cañete, A., Burks, D. J., Castel, V., et al. (2013). Targeting neuroblastoma stem cells with retinoic acid and proteasome inhibitor. PLoS One 8:e76761. doi: 10.1371/journal.pone.0076761
Hansford, L. M., Mckee, A. E., Zhang, L., George, R. E., Gerstle, J. T., Thorner, P. S., et al. (2007). Neuroblastoma cells isolated from bone marrow metastases contain a naturally enriched tumor-initiating cell. Cancer Res. 67, 11234–11243. doi: 10.1158/0008-5472.can-07-0718
Hao, H. X., Xie, Y., Zhang, Y., Charlat, O., Oster, E., Avello, M., et al. (2012). ZNRF3 promotes Wnt receptor turnover in an R-spondin-sensitive manner. Nature 485, 195–200. doi: 10.1038/nature11019
Hartomo, T. B., Van Huyen Pham, T., Yamamoto, N., Hirase, S., Hasegawa, D., Kosaka, Y., et al. (2015). Involvement of aldehyde dehydrogenase 1A2 in the regulation of cancer stem cell properties in neuroblastoma. Int. J. Oncol. 46, 1089–1098. doi: 10.3892/ijo.2014.2801
He, S., Liu, Z., Oh, D.-Y., and Thiele, C. (2013). MYCN and the epigenome. Front. Oncol. 3:1. doi: 10.3389/fonc.2013.00001
He, S. Z., Busfield, S., Ritchie, D. S., Hertzberg, M. S., Durrant, S., Lewis, I. D., et al. (2015). A Phase 1 study of the safety, pharmacokinetics and anti-leukemic activity of the anti-CD123 monoclonal antibody CSL360 in relapsed, refractory or high-risk acute myeloid leukemia. Leuk. Lymphoma 56, 1406–1415. doi: 10.3109/10428194.2014.956316
Held-Feindt, J., Schmelz, S., Hattermann, K., Mentlein, R., Mehdorn, H. M., and Sebens, S. (2012). The neural adhesion molecule L1CAM confers chemoresistance in human glioblastomas. Neurochem. Int. 61, 1183–1191. doi: 10.1016/j.neuint.2012.08.011
Hirsch, D., Barker, N., Mcneil, N., Hu, Y., Camps, J., Mckinnon, K., et al. (2014). LGR5 positivity defines stem-like cells in colorectal cancer. Carcinogenesis 35, 849–858. doi: 10.1093/carcin/bgt377
Hirschmann-Jax, C., Foster, A. E., Wulf, G. G., Nuchtern, J. G., Jax, T. W., Gobel, U., et al. (2004). A distinct ”side population” of cells with high drug efflux capacity in human tumor cells. Proc. Natl. Acad. Sci. U.S.A. 101, 14228–14233. doi: 10.1073/pnas.0400067101
Hjelmeland, A. B., and Rich, J. N. (2011). Molecular targeting of neural cancer stem cells: TTAGGG, you’re it! Clin. Cancer Res. 17, 3–5. doi: 10.1158/1078-0432.ccr-10-2686
Holmquist-Mengelbier, L., Fredlund, E., Lofstedt, T., Noguera, R., Navarro, S., Nilsson, H., et al. (2006). Recruitment of HIF-1alpha and HIF-2alpha to common target genes is differentially regulated in neuroblastoma: HIF-2alpha promotes an aggressive phenotype. Cancer Cell 10, 413–423. doi: 10.1016/j.ccr.2006.08.026
Hong, H., Brown, C. E., Ostberg, J. R., Priceman, S. J., Chang, W.-C., Weng, L., et al. (2016). L1 cell adhesion molecule-specific chimeric antigen receptor-redirected human T cells exhibit specific and efficient antitumor activity against human ovarian cancer in mice. PLoS One 11:e0146885. doi: 10.1371/journal.pone.0146885
Hong, H., Stastny, M., Brown, C., Chang, W. C., Ostberg, J. R., Forman, S. J., et al. (2014). Diverse solid tumors expressing a restricted epitope of L1-CAM can be targeted by chimeric antigen receptor redirected T lymphocytes. J. Immunother. 37, 93–104. doi: 10.1097/cji.0000000000000018
Hsu, D. M., Agarwal, S., Benham, A., Coarfa, C., Trahan, D. N., Chen, Z., et al. (2013). G-CSF receptor positive neuroblastoma subpopulations are enriched in chemotherapy-resistant or relapsed tumors and are highly tumorigenic. Cancer Res. 73, 4134–4146. doi: 10.1158/0008-5472.can-12-4056
Ikegaki, N., Shimada, H., Fox, A. M., Regan, P. L., Jacobs, J. R., Hicks, S. L., et al. (2013). Transient treatment with epigenetic modifiers yields stable neuroblastoma stem cells resembling aggressive large-cell neuroblastomas. Proc. Natl. Acad. Sci. U.S.A. 110, 6097–6102. doi: 10.1073/pnas.1118262110
Islam, M. O., Kanemura, Y., Tajria, J., Mori, H., Kobayashi, S., Hara, M., et al. (2005). Functional expression of ABCG2 transporter in human neural stem/progenitor cells. Neurosci. Res. 52, 75–82. doi: 10.1016/j.neures.2005.01.013
Jensen, T., Vadasz, S., Phoenix, K., Claffey, K., Parikh, N., and Finck, C. (2015). Descriptive analysis of tumor cells with stem like phenotypes in metastatic and benign adrenal tumors. J. Pediatr. Surg. 50, 1493–1501. doi: 10.1016/j.jpedsurg.2015.04.012
Jiang, M., Stanke, J., and Lahti, J. M. (2011). The connections between neural crest development and neuroblastoma. Curr. Top. Dev. Biol. 94, 77–127. doi: 10.1016/B978-0-12-380916-2.00004-8
Jin, J., Desai, B. N., Navarro, B., Donovan, A., Andrews, N. C., and Clapham, D. E. (2008). Deletion of Trpm7 disrupts embryonic development and thymopoiesis without altering Mg2+ homeostasis. Science 322, 756–760. doi: 10.1126/science.1163493
Jin, J., Wu, L. J., Jun, J., Cheng, X., Xu, H., Andrews, N. C., et al. (2012). The channel kinase, TRPM7, is required for early embryonic development. Proc. Natl. Acad. Sci. U.S.A. 109, E225–E233. doi: 10.1073/pnas.1120033109
Khalil, M. A., Hrabeta, J., Groh, T., Prochazka, P., Doktorova, H., and Eckschlager, T. (2016). Valproic acid increases CD133 positive cells that show low sensitivity to cytostatics in neuroblastoma. PLoS One 11:e0162916. doi: 10.1371/journal.pone.0162916
Kim, Y. (2010). Effect of retinoic acid and delta-like 1 homologue (DLK1) on differentiation in neuroblastoma. Nutr. Res. Pract. 4, 276–282. doi: 10.4162/nrp.2010.4.4.276
Kim, Y., Kim, K. H., Lee, J., Lee, Y. A., Kim, M., Lee, S. J., et al. (2012). Wnt activation is implicated in glioblastoma radioresistance. Lab. Invest. 92, 466–473. doi: 10.1038/labinvest.2011.161
Kim, Y., Lin, Q., Zelterman, D., and Yun, Z. (2009). Hypoxia-regulated delta-like 1 homologue enhances cancer cell stemness and tumorigenicity. Cancer Res. 69, 9271–9280. doi: 10.1158/0008-5472.CAN-09-1605
Kim, Y. S., Kim, E., Park, Y. J., and Kim, Y. (2016). Retinoic acid receptor beta enhanced the anti-cancer stem cells effect of beta-carotene by down-regulating expression of delta-like 1 homologue in human neuroblastoma cells. Biochem. Biophys. Res. Commun. 480, 254–260. doi: 10.1016/j.bbrc.2016.10.041
Kobayashi, K., Jakt, L. M., and Nishikawa, S. I. (2013). Epigenetic regulation of the neuroblastoma genes, Arid3b and Mycn. Oncogene 32, 2640–2648. doi: 10.1038/onc.2012.285
Koppaka, V., Thompson, D. C., Chen, Y., Ellermann, M., Nicolaou, K. C., Juvonen, R. O., et al. (2012). Aldehyde dehydrogenase inhibitors: a comprehensive review of the pharmacology, mechanism of action, substrate specificity, and clinical application. Pharmacol. Rev. 64, 520–539. doi: 10.1124/pr.111.005538
Krupkova, O Jr, Loja, T., Redova, M., Neradil, J., Zitterbart, K., Sterba, J., et al. (2011). Analysis of nuclear nestin localization in cell lines derived from neurogenic tumors. Tumour Biol. 32, 631–639. doi: 10.1007/s13277-011-0162-9
Kunkele, A., Taraseviciute, A., Finn, L. S., Johnson, A. J., Berger, C., Finney, O., et al. (2017). Preclinical assessment of CD171-directed CAR T-cell adoptive therapy for childhood neuroblastoma: CE7 epitope target safety and product manufacturing feasibility. Clin. Cancer Res. 23, 466–477. doi: 10.1158/1078-0432.ccr-16-0354
Kunnimalaiyaan, S., Schwartz, V. K., Jackson, I. A., Clark Gamblin, T., and Kunnimalaiyaan, M. (2018). Antiproliferative and apoptotic effect of LY2090314, a GSK-3 inhibitor, in neuroblastoma in vitro. BMC Cancer 18:560. doi: 10.1186/s12885-018-4474-7
Kuo, Y. T., Liu, Y. L., Adebayo, B. O., Shih, P. H., Lee, W. H., Wang, L. S., et al. (2015). JARID1B expression plays a critical role in chemoresistance and stem cell-like phenotype of neuroblastoma cells. PLoS One 10:e0125343. doi: 10.1371/journal.pone.0125343
Kwon, S. J., Kwon, O. S., Kim, K. T., Go, Y. H., Yu, S. I., Lee, B. H., et al. (2017). Role of MEK partner-1 in cancer stemness through MEK/ERK pathway in cancerous neural stem cells, expressing EGFRviii. Mol. Cancer 16:140. doi: 10.1186/s12943-017-0703-y
Laborda, J. (2000). The role of the epidermal growth factor-like protein dlk in cell differentiation. Histol. Histopathol. 15, 119–129. doi: 10.14670/HH-15.119
Lange, I., Espinoza-Fuenzalida, I., Ali, M. W., Serrano, L. E., and Koomoa, D. T. (2017). FTY-720 induces apoptosis in neuroblastoma via multiple signaling pathways. Oncotarget 8, 109985–109999. doi: 10.18632/oncotarget.22452
Lau, S. T., Hansford, L. M., Chan, W. K., Chan, G. C., Wan, T. S., Wong, K. K., et al. (2015). Prokineticin signaling is required for the maintenance of a de novo population of c-KIT+cells to sustain neuroblastoma progression. Oncogene 34, 1019–1034. doi: 10.1038/onc.2014.24
Lee, H. A., Park, S., and Kim, Y. (2013). Effect of beta-carotene on cancer cell stemness and differentiation in SK-N-BE(2)C neuroblastoma cells. Oncol. Rep. 30, 1869–1877. doi: 10.3892/or.2013.2643
Li, J., Zhong, L., Wang, F., and Zhu, H. (2017). Dissecting the role of AMP-activated protein kinase in human diseases. Acta Pharm. Sin. B 7, 249–259. doi: 10.1016/j.apsb.2016.12.003
Li, Q., Shi, L., Gui, B., Yu, W., Wang, J., Zhang, D., et al. (2011). Binding of the JmjC demethylase JARID1B to LSD1/NuRD suppresses angiogenesis and metastasis in breast cancer cells by repressing chemokine CCL14. Cancer Res. 71, 6899–6908. doi: 10.1158/0008-5472.can-11-1523
Lim, J. Y., Kim, Y. S., Kim, K. M., Min, S. J., and Kim, Y. (2014). Beta-carotene inhibits neuroblastoma tumorigenesis by regulating cell differentiation and cancer cell stemness. Biochem. Biophys. Res. Commun. 450, 1475–1480. doi: 10.1016/j.bbrc.2014.07.021
Lozier, A. M., Rich, M. E., Grawe, A. P., Peck, A. S., Zhao, P., Chang, A. T., et al. (2015). Targeting ornithine decarboxylase reverses the LIN28/Let-7 axis and inhibits glycolytic metabolism in neuroblastoma. Oncotarget 6, 196–206. doi: 10.18632/oncotarget.2768
Lubanska, D., and Porter, L. A. (2014). The atypical cell cycle regulator Spy1 suppresses differentiation of the neuroblastoma stem cell population. Oncoscience 1, 336–348. doi: 10.18632/oncoscience.36
Lutz, W., Fulda, S., Jeremias, I., Debatin, K. M., and Schwab, M. (1998). MycN and IFNgamma cooperate in apoptosis of human neuroblastoma cells. Oncogene 17, 339–346. doi: 10.1038/sj.onc.1200201
Marzi, I., D’amico, M., Biagiotti, T., Giunti, S., Carbone, M. V., Fredducci, D., et al. (2007). Purging of the neuroblastoma stem cell compartment and tumor regression on exposure to hypoxia or cytotoxic treatment. Cancer Res. 67, 2402–2407. doi: 10.1158/0008-5472.can-06-3208
Mehrazma, M., Madjd, Z., Kalantari, E., Panahi, M., Hendi, A., and Shariftabrizi, A. (2013). Expression of stem cell markers, CD133 and CD44, in pediatric solid tumors: a study using tissue microarray. Fetal Pediatr. Pathol. 32, 192–204. doi: 10.3109/15513815.2012.701266
Menaa, F., Houben, R., Eyrich, M., Broecker, E. B., Becker, J. C., and Wischhusen, J. (2009). Stem cells, melanoma and cancer stem cells: the good, the bad and the evil? G. Ital. Dermatol. Venereol. 144, 287–296.
Middelbeek, J., Kuipers, A. J., Henneman, L., Visser, D., Eidhof, I., Van Horssen, R., et al. (2012). TRPM7 is required for breast tumor cell metastasis. Cancer Res. 72, 4250–4261. doi: 10.1158/0008-5472.can-11-3863
Middelbeek, J., Visser, D., Henneman, L., Kamermans, A., Kuipers, A. J., Hoogerbrugge, P. M., et al. (2015). TRPM7 maintains progenitor-like features of neuroblastoma cells: implications for metastasis formation. Oncotarget 6, 8760–8776. doi: 10.18632/oncotarget.3315
Miraglia, S., Godfrey, W., Yin, A. H., Atkins, K., Warnke, R., Holden, J. T., et al. (1997). A novel five-transmembrane hematopoietic stem cell antigen: isolation, characterization, and molecular cloning. Blood 90, 5013–5021.
Mizrak, D., Brittan, M., and Alison, M. R. (2008). CD133: molecule of the moment. J. Pathol. 214, 3–9. doi: 10.1002/path.2283
Molofsky, A. V., Pardal, R., Iwashita, T., Park, I. K., Clarke, M. F., and Morrison, S. J. (2003). Bmi-1 dependence distinguishes neural stem cell self-renewal from progenitor proliferation. Nature 425, 962–967. doi: 10.1038/nature02060
Monajemzadeh, M., Soleimani, V., Vasei, M., Koochakzadeh, L., and Karbakhsh, M. (2014). Expression and prognostic significance of Oct4 and Nanog in neuroblastoma. APMIS 122, 734–741. doi: 10.1111/apm.12207
Moore, M. A., Williams, N., and Metcalf, D. (1973). In vitro colony formation by normal and leukemic human hematopoietic cells: characterization of the colony-forming cells. J. Natl. Cancer Inst. 50, 603–623.
Moreno-Smith, M., Lakoma, A., Chen, Z., Tao, L., Scorsone, K. A., Schild, L., et al. (2017). p53 non-genotoxic activation and mTORC1 inhibition lead to effective combination for neuroblastoma therapy. Clin. Cancer Res. 23, 6629–6639. doi: 10.1158/1078-0432.ccr-17-0668
Morozova, O., Vojvodic, M., Grinshtein, N., Hansford, L. M., Blakely, K. M., Maslova, A., et al. (2010). System-level analysis of neuroblastoma tumor-initiating cells implicates AURKB as a novel drug target for neuroblastoma. Clin. Cancer Res. 16, 4572–4582. doi: 10.1158/1078-0432.ccr-10-0627
Mosse, Y. P., Laudenslager, M., Longo, L., Cole, K. A., Wood, A., Attiyeh, E. F., et al. (2008). Identification of ALK as a major familial neuroblastoma predisposition gene. Nature 455, 930–935. doi: 10.1038/nature07261
Mouhieddine, T. H., Nokkari, A., Itani, M. M., Chamaa, F., Bahmad, H., Monzer, A., et al. (2015). Metformin and Ara-a effectively suppress brain cancer by targeting cancer stem/progenitor cells. Front. Neurosci. 9:442. doi: 10.3389/fnins.2015.00442
Munchar, M. J., Sharifah, N. A., Jamal, R., and Looi, L. M. (2003). CD44s expression correlated with the International Neuroblastoma Pathology Classification (Shimada system) for neuroblastic tumours. Pathology 35, 125–129.
Muñoz, P., Iliou, M. S., and Esteller, M. (2012). Epigenetic alterations involved in cancer stem cell reprogramming. Mol. Oncol. 6, 620–636. doi: 10.1016/j.molonc.2012.10.006
Nakata, S., Campos, B., Bageritz, J., Bermejo, J. L., Becker, N., Engel, F., et al. (2013). LGR5 is a marker of poor prognosis in glioblastoma and is required for survival of brain cancer stem-like cells. Brain Pathol. 23, 60–72. doi: 10.1111/j.1750-3639.2012.00618.x
Nardella, M., Guglielmi, L., Musa, C., Iannetti, I., Maresca, G., Amendola, D., et al. (2015). Down-regulation of the Lamin A/C in neuroblastoma triggers the expansion of tumor initiating cells. Oncotarget 6, 32821–32840. doi: 10.18632/oncotarget.5104
Naveen, C. R., Gaikwad, S., and Agrawal-Rajput, R. (2016). Berberine induces neuronal differentiation through inhibition of cancer stemness and epithelial-mesenchymal transition in neuroblastoma cells. Phytomedicine 23, 736–744. doi: 10.1016/j.phymed.2016.03.013
Ning, X., Zhang, H., Wang, C., and Song, X. (2018). Exosomes released by gastric cancer cells induce transition of Pericytes into cancer-associated fibroblasts. Med. Sci. Monit. 24, 2350–2359.
Nishimura, N., Hartomo, T. B., Pham, T. V., Lee, M. J., Yamamoto, T., Morikawa, S., et al. (2012). Epigallocatechin gallate inhibits sphere formation of neuroblastoma BE(2)-C cells. Environ. Health Prev. Med. 17, 246–251. doi: 10.1007/s12199-011-0239-5
Nishio, N., Fujita, M., Tanaka, Y., Maki, H., Zhang, R., Hirosawa, T., et al. (2012). Zoledronate sensitizes neuroblastoma-derived tumor-initiating cells to cytolysis mediated by human gammadelta T cells. J. Immunother. 35, 598–606. doi: 10.1097/CJI.0b013e31826a745a
Nowak, K., Kerl, K., Fehr, D., Kramps, C., Gessner, C., Killmer, K., et al. (2006). BMI1 is a target gene of E2F-1 and is strongly expressed in primary neuroblastomas. Nucleic Acids Res. 34, 1745–1754. doi: 10.1093/nar/gkl119
Opel, D., Poremba, C., Simon, T., Debatin, K. M., and Fulda, S. (2007). Activation of Akt predicts poor outcome in neuroblastoma. Cancer Res. 67, 735–745. doi: 10.1158/0008-5472.can-06-2201
Påhlman, S., and Mohlin, S. (2018). Hypoxia and hypoxia-inducible factors in neuroblastoma. Cell Tissue Res. 372, 269–275. doi: 10.1007/s00441-017-2701-1
Pajtler, K. W., Sadowski, N., Ackermann, S., Althoff, K., Schonbeck, K., Batzke, K., et al. (2017). The GSK461364 PLK1 inhibitor exhibits strong antitumoral activity in preclinical neuroblastoma models. Oncotarget 8, 6730–6741. doi: 10.18632/oncotarget.14268
Park, S., Kim, J., and Kim, Y. (2012). Mulberry leaf extract inhibits cancer cell stemness in neuroblastoma. Nutr. Cancer 64, 889–898. doi: 10.1080/01635581.2012.707280
Pode-Shakked, N., Harari-Steinberg, O., Haberman-Ziv, Y., Rom-Gross, E., Bahar, S., Omer, D., et al. (2011). Resistance or sensitivity of Wilms’ tumor to anti-FZD7 antibody highlights the Wnt pathway as a possible therapeutic target. Oncogene 30, 1664–1680. doi: 10.1038/onc.2010.549
Prasad, R., and Katiyar, S. K. (2016). “Honokiol, an active compound of magnolia plant, inhibits growth, and progression of cancers of different organs,” in Anti-inflammatory Nutraceuticals and Chronic Diseases, eds S. C. Gupta, S. Prasad, and B. B. Aggarwal (Cham: Springer), 245–265.
Rabadan, M. A., Usieto, S., Lavarino, C., and Marti, E. (2013). Identification of a putative transcriptome signature common to neuroblastoma and neural crest cells. Dev. Neurobiol. 73, 815–827. doi: 10.1002/dneu.22099
Rached, J., Nasr, Z., Abdallah, J., and Abou-Antoun, T. (2016). L1-CAM knock-down radiosensitizes neuroblastoma IMR-32 cells by simultaneously decreasing MycN, but increasing PTEN protein expression. Int. J. Oncol. 49, 1722–1730. doi: 10.3892/ijo.2016.3625
Rauschert, I., Aldunate, F., Preussner, J., Arocena-Sutz, M., Peraza, V., Looso, M., et al. (2017). Promoter hypermethylation as a mechanism for Lamin A/C silencing in a subset of neuroblastoma cells. PLoS One 12:e0175953. doi: 10.1371/journal.pone.0175953
Rehman, G., Shehzad, A., Khan, A. L., and Hamayun, M. (2014). Role of AMP-activated protein kinase in cancer therapy. Arch. Pharm. 347, 457–468. doi: 10.1002/ardp.201300402
Rettig, W. J., Spengler, B. A., Chesa, P. G., Old, L. J., and Biedler, J. L. (1987). Coordinate changes in neuronal phenotype and surface antigen expression in human neuroblastoma cell variants. Cancer Res. 47, 1383–1389.
Reya, T., Morrison, S. J., Clarke, M. F., and Weissman, I. L. (2001). Stem cells, cancer, and cancer stem cells. Nature 414, 105–111. doi: 10.1038/35102167
Ross, R. A., and Spengler, B. A. (2007). Human neuroblastoma stem cells. Semin. Cancer Biol. 17, 241–247. doi: 10.1016/j.semcancer.2006.04.006
Ross, R. A., Spengler, B. A., Domenech, C., Porubcin, M., Rettig, W. J., and Biedler, J. L. (1995). Human neuroblastoma I-type cells are malignant neural crest stem cells. Cell Growth Differ. 6, 449–456.
Ross, R. A., Walton, J. D., Han, D., Guo, H. F., and Cheung, N. K. (2015). A distinct gene expression signature characterizes human neuroblastoma cancer stem cells. Stem Cell Res. 15, 419–426. doi: 10.1016/j.scr.2015.08.008
Rouleau, C., Smale, R., Sancho, J., Fu, Y. S., Kurtzberg, L., Weber, W., et al. (2011). Endosialin: a novel malignant cell therapeutic target for neuroblastoma. Int. J. Oncol. 39, 841–851. doi: 10.3892/ijo.2011.1091
Ruella, M., Barrett, D. M., Kenderian, S. S., Shestova, O., Hofmann, T. J., Perazzelli, J., et al. (2016). Dual CD19 and CD123 targeting prevents antigen-loss relapses after CD19-directed immunotherapies. J. Clin. Invest. 126, 3814–3826. doi: 10.1172/jci87366
Ruggeri, P., Farina, A. R., Di Ianni, N., Cappabianca, L., Ragone, M., Ianni, G., et al. (2014). The TrkAIII oncoprotein inhibits mitochondrial free radical ROS-induced death of SH-SY5Y neuroblastoma cells by augmenting SOD2 expression and activity at the mitochondria, within the context of a tumour stem cell-like phenotype. PLoS One 9:e94568. doi: 10.1371/journal.pone.0094568
Sartelet, H., Imbriglio, T., Nyalendo, C., Haddad, E., Annabi, B., Duval, M., et al. (2012). CD133 expression is associated with poor outcome in neuroblastoma via chemoresistance mediated by the AKT pathway. Histopathology 60, 1144–1155. doi: 10.1111/j.1365-2559.2012.04191.x
Sayegh, J., Cao, J., Zou, M. R., Morales, A., Blair, L. P., Norcia, M., et al. (2013). Identification of small molecule inhibitors of Jumonji AT-rich interactive domain 1B (JARID1B) histone demethylase by a sensitive high throughput screen. J. Biol. Chem. 288, 9408–9417. doi: 10.1074/jbc.M112.419861
Seo, Y., Kim, Y. S., Lee, K. E., Park, T. H., and Kim, Y. (2017). Anti-cancer stemness and anti-invasive activity of bitter taste receptors, TAS2R8 and TAS2R10, in human neuroblastoma cells. PLoS One 12:e0176851. doi: 10.1371/journal.pone.0176851
Shah, R. D., Jagtap, J. C., Mruthyunjaya, S., Shelke, G. V., Pujari, R., Das, G., et al. (2013). Sodium valproate potentiates staurosporine-induced apoptosis in neuroblastoma cells via Akt/survivin independently of HDAC inhibition. J. Cell. Biochem. 114, 854–863. doi: 10.1002/jcb.24422
Shi, Y., Chichung Lie, D., Taupin, P., Nakashima, K., Ray, J., Yu, R. T., et al. (2004). Expression and function of orphan nuclear receptor TLX in adult neural stem cells. Nature 427, 78–83. doi: 10.1038/nature02211
Siapati, E. K., Rouka, E., Kyriakou, D., and Vassilopoulos, G. (2011). Neuroblastoma cells negative for CD44 possess tumor-initiating properties. Cell. Oncol. 34, 189–197. doi: 10.1007/s13402-011-0022-z
Singh, S. K., Clarke, I. D., Terasaki, M., Bonn, V. E., Hawkins, C., Squire, J., et al. (2003). Identification of a cancer stem cell in human brain tumors. Cancer Res. 63, 5821–5828.
Smith, K. M., Datti, A., Fujitani, M., Grinshtein, N., Zhang, L., Morozova, O., et al. (2010). Selective targeting of neuroblastoma tumour-initiating cells by compounds identified in stem cell-based small molecule screens. EMBO Mol. Med. 2, 371–384. doi: 10.1002/emmm.201000093
Suebsoonthron, J., Jaroonwitchawan, T., Yamabhai, M., and Noisa, P. (2017). Inhibition of WNT signaling reduces differentiation and induces sensitivity to doxorubicin in human malignant neuroblastoma SH-SY5Y cells. Anticancer Drugs 28, 469–479. doi: 10.1097/cad.0000000000000478
Suzuki, S., Namiki, J., Shibata, S., Mastuzaki, Y., and Okano, H. (2010). The neural stem/progenitor cell marker Nestin is expressed in proliferative endothelial cells, but not in mature vasculature. J. Histochem. Cytochem. 58, 721–730. doi: 10.1369/jhc.2010.955609
Takenobu, H., Shimozato, O., Nakamura, T., Ochiai, H., Yamaguchi, Y., Ohira, M., et al. (2011). CD133 suppresses neuroblastoma cell differentiation via signal pathway modification. Oncogene 30, 97–105. doi: 10.1038/onc.2010.383
Tenbaum, S. P., Ordonez-Moran, P., Puig, I., Chicote, I., Arques, O., Landolfi, S., et al. (2012). beta-catenin confers resistance to PI3K and AKT inhibitors and subverts FOXO3a to promote metastasis in colon cancer. Nat. Med. 18, 892–901. doi: 10.1038/nm.2772
Theiss, A. L., and Sitaraman, S. V. (2011). The role and therapeutic potential of prohibitin in disease. Biochim. Biophys. Acta 1813, 1137–1143. doi: 10.1016/j.bbamcr.2011.01.033
Thomas, S. K., Messam, C. A., Spengler, B. A., Biedler, J. L., and Ross, R. A. (2004). Nestin is a potential mediator of malignancy in human neuroblastoma cells. J. Biol. Chem. 279, 27994–27999. doi: 10.1074/jbc.M312663200
Tian, X., Hou, W., Bai, S., Fan, J., Tong, H., and Xu, H. (2014). XAV939 inhibits the stemness and migration of neuroblastoma cancer stem cells via repression of tankyrase 1. Int. J. Oncol. 45, 121–128. doi: 10.3892/ijo.2014.2406
Toh, T. B., Lim, J. J., and Chow, E. K.-H. (2017). Epigenetics in cancer stem cells. Mol. Cancer 16:29. doi: 10.1186/s12943-017-0596-9
Tong, Q. S., Zheng, L. D., Tang, S. T., Ruan, Q. L., Liu, Y., Li, S. W., et al. (2008). Expression and clinical significance of stem cell marker CD133 in human neuroblastoma. World J. Pediatr. 4, 58–62. doi: 10.1007/s12519-008-0012-z
van Limpt, V., Chan, A., Caron, H., Sluis, P. V., Boon, K., Hermus, M. C., et al. (2000). SAGE analysis of neuroblastoma reveals a high expression of the human homologue of the Drosophila Delta gene. Med. Pediatr. Oncol. 35, 554–558.
Vangipuram, S. D., Buck, S. A., and Lyman, W. D. (2012). Wnt pathway activity confers chemoresistance to cancer stem-like cells in a neuroblastoma cell line. Tumor Biol. 33, 2173–2183. doi: 10.1007/s13277-012-0478-0
Vangipuram, S. D., Wang, Z. J., and Lyman, W. D. (2010). Resistance of stem-like cells from neuroblastoma cell lines to commonly used chemotherapeutic agents. Pediatr. Blood Cancer 54, 361–368. doi: 10.1002/pbc.22351
Vasiliou, V., Vasiliou, K., and Nebert, D. W. (2009). Human ATP-binding cassette (ABC) transporter family. Hum. Genomics 3, 281–290.
Vella, S., Penna, I., Longo, L., Pioggia, G., Garbati, P., Florio, T., et al. (2015). Perhexiline maleate enhances antitumor efficacy of cisplatin in neuroblastoma by inducing over-expression of NDM29 ncRNA. Sci. Rep. 5:18144. doi: 10.1038/srep18144
Vieira, G. C., Chockalingam, S., Melegh, Z., Greenhough, A., Malik, S., Szemes, M., et al. (2017). Correction: LGR5 regulates pro-survival MEK/ERK and proliferative Wnt/beta-catenin signalling in neuroblastoma. Oncotarget 8:32381. doi: 10.18632/oncotarget.17685
Walton, J. D., Kattan, D. R., Thomas, S. K., Spengler, B. A., Guo, H.-F., Biedler, J. L., et al. (2004). Characteristics of stem cells from human neuroblastoma cell lines and in tumors. Neoplasia 6, 838–845.
Wang, F., Wang, A. Y., Chesnelong, C., Yang, Y., Nabbi, A., Thalappilly, S., et al. (2018). ING5 activity in self-renewal of glioblastoma stem cells via calcium and follicle stimulating hormone pathways. Oncogene 37, 286–301. doi: 10.1038/onc.2017.324
Wang, K., Ji, W., Yu, Y., Li, Z., Niu, X., Xia, W., et al. (2018). FGFR1-ERK1/2-SOX2 axis promotes cell proliferation, epithelial-mesenchymal transition, and metastasis in FGFR1-amplified lung cancer. Oncogene 37, 5340–5354. doi: 10.1038/s41388-018-0311-3
Wesbuer, S., Lanvers-Kaminsky, C., Duran-Seuberth, I., Bolling, T., Schafer, K. L., Braun, Y., et al. (2010). Association of telomerase activity with radio- and chemosensitivity of neuroblastomas. Radiat. Oncol. 5:66. doi: 10.1186/1748-717x-5-66
Yanai, H., Atsumi, N., Tanaka, T., Nakamura, N., Komai, Y., Omachi, T., et al. (2017). Intestinal cancer stem cells marked by Bmi1 or Lgr5 expression contribute to tumor propagation via clonal expansion. Sci. Rep. 7:41838. doi: 10.1038/srep41838
Yang, L., Tang, H., Kong, Y., Xie, X., Chen, J., Song, C., et al. (2015). LGR5 promotes breast cancer progression and maintains stem-like cells through activation of Wnt/beta-catenin signaling. Stem Cells 33, 2913–2924. doi: 10.1002/stem.2083
Yin, A. H., Miraglia, S., Zanjani, E. D., Almeida-Porada, G., Ogawa, M., Leary, A. G., et al. (1997). AC133, a novel marker for human hematopoietic stem and progenitor cells. Blood 90, 5002–5012.
Yin, D., Xie, D., Sakajiri, S., Miller, C. W., Zhu, H., Popoviciu, M. L., et al. (2006). DLK1: increased expression in gliomas and associated with oncogenic activities. Oncogene 25, 1852–1861. doi: 10.1038/sj.onc.1209219
Zaatiti, H., Abdallah, J., Nasr, Z., Khazen, G., Sandler, A., and Abou-Antoun, T. J. (2018). Tumorigenic proteins upregulated in the MYCN-amplified IMR-32 human neuroblastoma cells promote proliferation and migration. Int. J. Oncol. 52, 787–803. doi: 10.3892/ijo.2018.4236
Zhang, L., Smith, K. M., Chong, A. L., Stempak, D., Yeger, H., Marrano, P., et al. (2009). In vivo antitumor and antimetastatic activity of sunitinib in preclinical neuroblastoma mouse model. Neoplasia 11, 426–435.
Keywords: neuroblastoma, cancer stem cells, molecular signatures, therapeutic targets, genetic aberrations
Citation: Bahmad HF, Chamaa F, Assi S, Chalhoub RM, Abou-Antoun T and Abou-Kheir W (2019) Cancer Stem Cells in Neuroblastoma: Expanding the Therapeutic Frontier. Front. Mol. Neurosci. 12:131. doi: 10.3389/fnmol.2019.00131
Received: 27 February 2019; Accepted: 07 May 2019;
Published: 27 May 2019.
Edited by:
Michele Papa, Università degli Studi della Campania Luigi Vanvitelli, ItalyReviewed by:
Assunta Virtuoso, Second University of Naples, ItalyRosa Gomez-Villafuertes, Complutense University of Madrid, Spain
Copyright © 2019 Bahmad, Chamaa, Assi, Chalhoub, Abou-Antoun and Abou-Kheir. This is an open-access article distributed under the terms of the Creative Commons Attribution License (CC BY). The use, distribution or reproduction in other forums is permitted, provided the original author(s) and the copyright owner(s) are credited and that the original publication in this journal is cited, in accordance with accepted academic practice. No use, distribution or reproduction is permitted which does not comply with these terms.
*Correspondence: Tamara Abou-Antoun, tamara.abouantoun@lau.edu.lb; Wassim Abou-Kheir, wa12@aub.edu.lb
†These authors have contributed equally to this work as co-first authors
‡These authors have contributed equally to this work as joint senior authors
§Present address: Reda M. Chalhoub, Medical Scientist Training Program, College of Medicine, Medical University of South Carolina, Charleston, SC, United States