Bronchoscopy for the diagnosis of peripheral lung lesions
Introduction
The last decade has been a game-changer in the arena of diagnostic bronchoscopy. The field of interventional pulmonary has blossomed with curvilinear endobronchial ultrasound (EBUS) becoming the preferred procedure for mediastinal staging of lung cancer. This has also been accompanied with significant improvement in the guidance technology for bronchoscopic sampling of peripheral lesions. This has the added benefit of simultaneous diagnosis and staging of lung cancer during a single procedure with lower risk of complications. Development of technologies like virtual bronchoscopy (VB), electromagnetic navigation bronchoscopy (ENB), ultra-miniature (UM) radial probe EBUS (RP-EBUS) and ultrathin bronchoscopes has been a boon for the bronchoscopists. One meta-analysis showed that the pooled diagnostic yield of these techniques was 70% and the complication rate was extremely low with a pneumothorax rate of 1.6% with only 0.7% requiring intercostal tube and no report of substantial bleeding or death (1). With such a low rate of complications and benefits of simultaneous staging along with an increasing yield of image guided bronchoscopy, wide spread interest has been generated in further advancing this capability and broader adoption of these technologies is already being seen.
Traditional flexible bronchoscopy
The procedure involves careful review of the CT anatomy of the lung to determine the bronchial pathway to the lesion before advancing the bronchoscope in the subsegment of interest and advancing the sampling equipment like brushes and transbronchial biopsy forceps distally towards the lesion. The sampling equipment typically doesn’t bend and tends to go straight which limits the ability to access many lesions. The normal anatomic variation in lobar airway anatomy needs to be carefully evaluated before memorizing the pathway. Although formalized nomenclature of the airways is not commonly used, using such terminology can be very helpful in memorizing the pathway to the lesion. Most of the studies of flexible bronchoscopy pre-navigation era were performed using CT images, which had only axial cuts, and thin cuts were not commonly performed. One commonly cited study from Houston VA described a low sensitivity of traditional bronchoscopy for lesions <2 cm at 25% although it was better at 69% for bigger lesions (2,3). Even analysis of several studies in American College of Chest Physicians (ACCP) guidelines indicates an overall sensitivity of flexible bronchoscopy at 34% for lesions <2 cm and 63% for >2 cm. In addition to the size of the lesion, the other factors contributing to higher yield were the presence of a bronchus sign and higher number of transbronchial biopsy specimens. Transbronchial needle aspiration probably had higher sensitivity than biopsy and brushes but was performed infrequently and the numbers were low to make an accurate conclusion in this analysis. Inter-bronchoscopists variability has not been well studied but one study described significant variability in diagnostic yield (4). Another study described very poor endobronchial path selection accuracy among bronchoscopists based on CT images, irrespective of the level of experience and this was improved with the use of virtual bronchoscopic based techniques (5).
VB and virtual bronchoscopic navigation
VB utilizes non-contrast CT of the chest to generate three-dimensional virtual image of the airways, which closely mimics the actual airways. As selection of endobronchial pathway to the lesion can be a potential major source of error in reaching a peripheral lesion (5), VB guidance can be very useful in selecting the appropriate airway. A recent study used only the VB feature of an available ENB system (superDimension i-LogicTM system) to plan pathways to 245 peripheral pulmonary lesions along with RP-EBUS and successfully localized 77% of lesions with a diagnostic yield of 54.7% (6). Addition of ENB to those cases where lesions were not confirmed by RP-EBUS or where rapid on site cytology was not achieved only modestly improved overall diagnostic yield to 58.4%. Of note, the prevalence of malignancy in this cohort was high at 82% and diagnostic yield for benign lesions was very low at 16.7%. Although not a direct comparison study of VB assistance with ENB technology, as ENB was performed only on the difficult cases, this study suggests that a combination of VB and RP-EBUS alone can be beneficial for a vast majority of the patients in a similar setting with a high prevalence of malignancy by assistance in selecting appropriate airways.
Virtual bronchoscopic navigation (VBN) involves navigation to the peripheral lesion-using pathway based on airways leading to lesion planned using VB and simultaneous aligning and superimposition of virtual views on the actual bronchoscopic views. There are two commercially available systems LungPoint® System (Bronchus Medical, San Jose, CA, USA) and the Bf-NAAVI® System (Cybernet Systems, Tokyo, Japan) now called Directpath® (Olympus, Japan). The LungPoint® System performs segmentation of ≥3 mm diameter airways and major vessels by calculating a central axis for each airway after obtaining a CT with 1.25 mm slice thickness and 0.0625 mm interval at end inspiratory hold (as the airways are maximally expanded). During bronchoscopy the live bronchoscopy and VB image are synchronized but requires an assistant to facilitate this by advancing and adjusting the VB image (7). The system can automatically select the virtual image corresponding to the bronchoscopic view using image pattern recognition along with superimposition of target and vessels surrounding the airway on the real bronchoscopy view however this feature requires that the bronchoscope be advanced slowly due to longer time required by image pattern recognition (8). Small human clinical trials have reported a diagnostic yield of 77–80% in cohorts with high prevalence of malignancy without any significant adverse effects (7,9). The Bf-NAAVI® System (not available in USA) has been studied more extensively and similarly used CT data for Multi-Planar Reconstruction and thus generates a three-dimensional image of the tracheobronchial tree. The VB images can be moved forward-backwards and rotated like a bronchoscope and similar to the other system, needs an assistance to perform this task. The Virtual Navigation in Japan (V-NINJA) trial group performed a randomized multicenter trial comparing VBN (N=102) using Bf-NAAVI® System vs. non-VBN guided bronchoscopy (N=97) on peripheral lesions ≤3 cm along with use of RP-EBUS with guide sheath (GS) and fluoroscopy (10). The study showed that the VBN group had significantly higher yield than non-VBN group (80.4% vs. 67.0%; P=0.032) and procedure duration was shorter (10). Another larger multicenter trial comparing the Bf-NAVI VBN and non-VBN (N= 167 each group) for ≤3 cm peripheral pulmonary lesions suspicious for lung cancer along with the use of ultrathin bronchoscopes (outer diameter of 2.8 mm and working channel of 1.2 mm) and with fluoroscopic guidance (RP-EBUS not utilized as working channel size was small) showed a trend of higher diagnostic yield in VBN group although this was not statistically significant (diagnostic yield 67.1% in VBN vs. 59.9% in Non-VBN; P=0.173) (11). The peripheral pulmonary lesions in this study were defined as ≤3 cm lesions surrounded by normal lung parenchyma, which were unlikely to be visualized by bronchoscopy. On subgroup analysis, the diagnostic yield was significantly higher for VBN group for right upper lobe lesions, lesions invisible on posterior-anterior chest radiographs and for lesions in peripheral third of lung field (64.7% vs. 52.1%, P=0.047). Variation in definition of peripheral pulmonary lesions used in each study needs to be kept in mind along with the use of additional technology utilized in each study (like radial EBUS, fluoroscopy, CT scan) before generalizing the results.
A recent review of literature reported an overall diagnostic yield of VBN to be 73.8% with a yield of 67.4% for lesions ≤2 cm (8) with most of included studies using Bf-Navi® system. Although no complications directly related to VB have been reported, the complication rate of VB guided procedures was very low at 1% (8). Factors affecting yield are hard to determine due to small sample size and considerable heterogeneity in studies although multivariate analysis have suggested that location of lesion (with low yield in left lower lobe superior segment), concentric RP-EBUS probe localization of lesion being better than eccentric and yield being higher for solid as compared to sub-solid lesions (8).
VB has several limitations. VB needs selection of appropriate threshold to differentiate airway wall from lumen or otherwise airway bifurcations can be missed (8,12). Presence of mucus blocking airway may make it hard to visualize small airways on CT. VB is also unable to factor in the target movement due to respiration or other factors that may change the target location after performance of CT like an enlarging pleural effusion or lesion movement related to bronchoscope. The procedure is not real time and rate of successful navigation may not translate into similar diagnostic yields (11). Peripheral lesions without an airway leading to them are difficult to sample using VBN. The high cost of the systems and the additional radiation exposure for CT acquisition are also potential drawbacks to wider adaption of this technology.
Identification of correct bronchus may be difficult as the scope is often rotated while being advanced during an actual bronchoscopic procedure. Additionally, if an exact bronchus of the desired pathway is missed due to poor vision during bronchoscopy or unexpected sudden movement like cough, then there is no feedback alerting about this mistake.
Ultrathin bronchoscopy
Small bronchoscopes with an outer diameter of 2.8–3.5 mm are considered ultrathin although a formal definition doesn’t exist. The small size of ultrathin bronchoscopes allows better maneuverability and they can visualize deeper into the tracheobronchial tree and can reach up to the sixth to eight-generation bronchi. The ultrathin bronchoscopes are usually used with image guidance technology to reach close to the peripheral lesions. The concept of ultrathin bronchoscopes is not new as Tanaka et al. described a small bronchoscope with 1.8 mm outer diameter but without a working channel in 1984 and it could reach up to eight generation airways (13). Subsequently working channel was added and an ultrathin bronchoscope with an outer diameter of 2.7 mm and working channel of 0.8 mm was described in 1996, which allowed suction, lavage and bronchography (14). The technology has come a long way since then and the new ultrathin bronchoscopes offer a variety of sizes of outer diameter/working channel and with improved flexion/extension along with brushings and transbronchial biopsies. For example, the current peripheral or thin series of Olympus bronchoscopes has outer diameter of 4.2 and working channel of 2.0 mm (BF-P190) while the “ultrathin” series has outer diameter of 3.1 mm and working channel of 1.2 mm (BF-XP190).
Direct comparison studies between ultrathin bronchoscopy and standard bronchoscopy or other image guidance techniques have not yet been performed. Ultrathin bronchoscopy has mostly been used in studies of other image guidance bronchoscopic techniques and one meta-analysis of image guided bronchoscopy showed that ultrathin bronchoscopy was used in 11 out of 39 studies that were included. Only two of the studies used ultrathin bronchoscopy alone with one small study having 60% yield (21/35) (15) and another study showed a yield of 69.4% (68/98) (16). Both studies reported no complications. One multicenter randomized prospective study compared a 3.0 mm outer diameter and 1.7 mm working channel ultrathin with 4.0 mm outer diameter and 2.0 mm working channel thin bronchoscope for PPLs (17). VBN, fluoroscopy and RP-EBUS guidance was used in both groups although a GS could be used in the latter group due to larger working channel. The diagnostic yield of ultrathin bronchoscope was significantly higher than the thin bronchoscope with GS (74% vs. 59%; P=0.044).
RP-EBUS
The UM RP-EBUS has a 20 MHz transducer at the tip which rotates 360° perpendicular to the direction of insertion and obtains real-time high-resolution images of the structures surrounding the airways. This can help in localization of the lesions, which are mostly hypoechoic and therefore have a well-defined border with normal lung tissue on ultrasound images, thus aiding in tissue sampling of the appropriate area. This technology can therefore allow the proceduralists to look into the distal small airways, something that was beyond the realm of flexible bronchoscope. The RP-EBUS can be inserted directly through the working channel of bronchoscopes or can be used with a GS or through extended working channel (EWC) of electromagnetic navigation systems into the target bronchus to confirm appropriate localization of the area of interest. The target bronchus can be identified by careful review of CT images by virtual navigation or electromagnetic navigation techniques. Once the lesion is confirmed, the GS is fixed in place and RP-EBUS is removed and various sampling instruments can be advanced through the GS to sample the lesion. Some proceduralists may use fluoroscopy for additional assistance. In cases of difficulty in accessing the lesion, a double-hinged curette can be inserted into the GS and can be directed under fluoroscopic guidance into the appropriate bronchus. The guiding device can then be removed and lesion confirmed with RP-EBUS before sampling.
Two kinds of UM RP-EBUS probes made by Olympus are commonly available with appropriate GS kits with outer diameter of 1.7 mm (UM-S20-20R), and 1.4 mm (UM-S20-17S) respectively. The 1.7 mm probe can fit in a working channel of 2.2 cm without a GS and 2.6 mm if used with a GS and the 1.4 mm probe can fit in a working channel as small as 2.0 mm. Thus the 1.4 mm probe can be used with smaller bronchoscopes with working channel of 2.0 mm, which may allow it to be directed deeper into bronchopulmonary segments (Figure 1). The GS may be kept in place for few minutes after biopsy as it may reduce the risk of bleeding due to tamponade effect. Unfortunately, the transbronchial needles are too large to be used with the available GS kits.
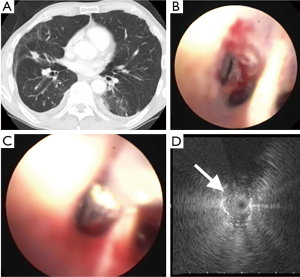
Direct contact with the lesion is important for ultrasound visualization. Usually the UM probes obtain a direct contact with the smaller peripheral airways without any difficulty and sometimes instillation of small amount of saline in GS or EWC can be helpful to obtaining better images. The normal lung typically has a “snowstorm” appearance due to the presence of air-filled alveolar tissue. Solid malignant lesions have a hyperechoic border separating it from normal lung. Small airways with trapped air appear as small hyperechoic dots, linear air bronchograms appear as continuous hyperechoic lines and blood vessels or areas of necrosis appear hypoechoic. Doppler mode is not available in UM-RP-EBUS to identify blood vessels.
The difference in echogenic strength of different tissues in the lung can be helpful in not only localizing the lesion but can also provide helpful clues to the etiology of the lesion. Kurimoto and colleagues described three type of lesions: Type I lesions have homogenous pattern and most of them were benign, type II lesions had hyperechoic dots and linear arc pattern and type III lesions had heterogeneous pattern and the later two were invariably malignant (18). Other authors have suggested that features like continuous margin, heterogeneous echogenicity and absence of linear-discrete air bronchograms may help differentiate malignant from benign lesions (19). However, additional validation in larger studies is needed before these features can be incorporated into routine clinical practice to make the critical decision about tissue sampling. Izumo et al. described the sonographic characteristic of ground glass opacity (GGO) peripheral lung lesions and their correlation with findings on chest CT imaging (20). Based on RP-EBUS images, a “blizzard sign” was described as an enlarged, diffuse hyperintense acoustic shadow. Peripheral lesions with more GGO component were associated with blizzard sign. Mixed blizzard signs were described as heterogeneous findings interrupting the blizzard sign and representing peripheral lung lesions with mixed GGO and solid components.
Studies have consistently shown much higher yield for RP-EBUS guided sampling of peripheral lesions as compared to traditional transbronchial biopsies. In one of the earlier performed randomized trial comparing RP-EBUS with traditional transbronchial biopsy the sensitivity of RP-EBUS for lesions <3 cm was 75% and for lesions <2 cm was 71% as compared with conventional transbronchial biopsy sensitivity of only 31% and 23%, respectively (21). The yield was however similar for lesions >3 cm. Kurimoto et al. used the guided sheath RP-EBUS to sample 150 lesions and were able to achieve diagnosis in 116 cases with a yield of 77% (22). The yield for malignant lesions was 81% (82/101) and for benign lesions was 73% (35/49). The diagnostic yield was significantly higher at 87% when the probe was in the center of the lesion (concentric view) and much lower at 42% when the probe was adjacent to the lesion (eccentric view) (22). This seems to be an important finding and has been confirmed in several other studies (23-26). Another recent retrospective study of 467 patients who underwent only RP-EBUS guided (GS used in 60%) bronchoscopy reported successful identification of 96% of lesions and a diagnostic yield of 69% and the yield increased with increasing size of the nodule (26). Even in small lesions not visible by fluoroscopy (mean diameter 2.2 cm), Herth et al. were able to localize the lesions in 89% of the cases and obtained a yield of 70%.
Based on systemic reviews and meta-analysis, the overall sensitivity of RP-EBUS for diagnosis of peripheral lesions is around 70% although there is considerable heterogeneity in nodule characteristics and variable use of additional image guiding technology in included studies (1,25). The diagnostic yield reported in studies depends on the prevalence of malignancy in their cohort, as higher prevalence of malignancy is associated with higher yield. Although the diagnostic yield seems to be dependent on the size of the nodule, a part of this finding could be due to lower prevalence of malignancy in smaller nodules (25). Although suggested, it is still not fully clear if yield is higher for malignant lesions as compared to benign lesions and if the lobar distribution or distance of lesion from hilum impacts the yield (25). The optimal number of biopsies to maximize yield by RP-EBUS method has been reported to be five (24). The procedure overall is safe with a meta-analysis reporting a pneumothorax rate of around 1% and intercostal catheter drainage required in only 0.4% of cases (25). Minor bleeding not requiring any intervening is another infrequent complication. Major limitations of the technique include operator dependence and poor visualization of ground glass opacities.
Electromagnetic navigational bronchoscopy
The ENB system works similar to the global positioning system (GPS) of the vehicles. The equipment generates an electromagnetic (EM) field around the thorax and a small EM sensor serves as the position locator inside this field. Like VB, the ENB technology requires thin-section CT to create a virtual bronchial tree. This CT is performed prior to the procedure and therefore ENB is not a “real-time” technique. The lesion is reviewed in the axial, sagittal, and coronal planes and marked as the target. Endobronchial pathways to the lesion are planned and marked using the virtual airways. The patient is placed in the EM field generated by a source connected to the computer loaded with the plan and sensors or pads are placed on the chest of the patient to help determine the position of EM sensor in the EM field and compensate for respiratory movements. The catheter with the EM sensor at tip may be initially advanced in various segments of airways which synchronies and matches the virtual airway with the real anatomy, a process called registration. The current systems can perform automatic registration. The success of procedure depends on the accuracy of registration (CT to body divergence). After successful registration, the target-locating sensor is steered towards the lesion and the relationship and proximity of the locating sensor to the lesion is depicted on the computer screen with several customizable views. Once the target lesion is navigated, appropriate sampling can be obtained with or without the help of fluoroscopy.
Two commercial ENB systems are currently available: superDimension i-LogicTM (Medtronic, Plymouth, MN, USA) and SPiN SystemTM (Veran Medical Technologies, Inc., St. Louis, MO, USA). Both techniques use EM fields although the i-LogicTM system generated the EM field with a board placed underneath the patient and the SPiN SystemTM system generates the EM field with a portable unit positioned over the thorax of the patient. The i-LogicTM system uses an end inspiratory hold CT chest for planning and the movement of target related to breathing is calculated mathematically. The SPiN SystemTM uses both inspiratory and expiratory CT and the sensor pads use respiratory gating to correct for lesion movement. The sensor (called locatable guided or LG) of the i-LogicTM system is placed in a sheath called the EWCs, and this assembly requires a larger working channel of the bronchoscope of at least 2.6 mm. The LG is removed once the lesion is navigated and various sampling instruments are passed through the EWC for sampling. The sampling tracker is incorporated inside the sampling devices (Always On Tip-Tracked® instruments) of SPiN SystemTM thus enabling direct navigation of sampling instruments to the lesions. The i-LogicTM system has several different catheters with different angles of tip (45, 90, 180 degree) to help in steering while the SPiN SystemTM can utilize smaller bronchoscopes and also has a phantom bronchoscopy catheter that helps in steering towards lesion. Unlike i-LogicTM system the SPiN SystemTM can also aid in transthoracic needle sampling although this is not “real-time” navigation either. These two systems have not yet been directly compared in any clinical trials and most of the available literature is based on superDimension i-LogicTM system. One retrospective study showed no difference in yield between conscious intravenous sedation and general anesthesia although the procedure time was shorter under general anesthesia (27). General anesthesia may also allow a brief “breath-hold’ to sample a lesion having significant respiratory motion.
Several factors need to be considered when making a decision about performing ENB over CT guided biopsy. Individual patient factors like the ability to tolerate sedation, location of the lesion, pre-test probability of successfully obtaining a diagnosis, and local expertise of technology plays a key role. Other factors that favor ENB are if CT guided biopsy is not feasible due to anatomical factors (presence of emphysema, lesion adjacent to major vessel) or has been non-diagnostic, when bronchoscopy is needed anyway for other indications like mediastinal staging, evaluation of central airways or work up of pulmonary infection, need for sampling of multiple lesions, obtaining additional tissue for subtyping or mutation testing or when placing markers for stereotactic radiation or lung resection(Figure 2) (28).
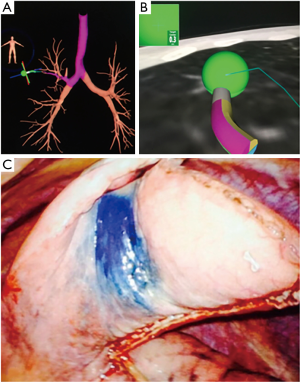
Many of the studies of ENB are small non-randomized single center studies with yield diagnostic yields ranging from 63% to 85% (29-33). A recent meta-analysis of ENB reported a pooled diagnostic sensitivity of 82% (34). Significant factors associated with higher sensitivity were larger nodule size, presence of bronchus sign, nodule visualization with RP-EBUS, lower registration error and use of catheter suction technique while nodule location in lower lobe was associated with lower yield (34). The complication rate is low with a pneumothorax rate of 3.1% with an intercostal drain placement rate of 1.6% (35). The highest yield with ENB to date has been reported with the use of multimodality technology. In a prospective randomized study ENB, RP-EBUS and the combination of both was evaluated (36). In the combination group the RP-EBUS was used for confirmation and if needed re-adjustment after navigation using ENB. The combined modality had a much higher diagnostic yield of 88% (which approximated the yield of CT guided sampling) as compared RP-EBUS and for ENB alone (69% and 59% respectively; P=0.02). Based on the results of this study, several centers routinely confirm ENB navigation with RP-EBUS.
The excitement surrounding ENB was dampened by the results of the AQuIRE (ACCP Quality Improvement Registry, Evaluation, and Education) registry, which unexpectedly reported a higher yield for non-image guided traditional bronchoscopy as compared to imaged guided bronchoscopy (37). The diagnostic yield of bronchoscopy without image guidance for peripheral nodules was 63.7%; with RP-EBUS guidance was 57%, with ENB was only 38.5% and with RP-EBUS along with ENB was slightly better at 47.1%. Significant variation in practice patterns of different centers was noted and peripheral transbronchial needle aspiration, which has been reported to be associated with higher yields, was infrequently used in only 16% of cases. The study raised an important question that diagnostic yield of RP-EBUS and ENB may be very low when performed outside the research setting. The authors felt that the lower yield could also be due to the lower prevalence of lung cancer in this cohort at 58% as compared to studies of RP-EBUS and ENB where prevalence of lung cancer was >70% (38). Other factors responsible for lower yield could be patient selection as the simple straightforward cases may have been performed just with traditional bronchoscopy and the fixation of location of EWC, which permits sampling of a small fixed area. Additional factors that may have contributed to lower yield include lack of follow up and final diagnosis for all patients included in the study and some of the lesions actually being central rather than peripheral (38). Although this study showed that bronchoscopy is safe for peripheral lesions with a low complication rate of 2.2% and a pneumothorax rate of 1.7%, it raised importance questions on performance of image guided bronchoscopy especially ENB outside research setting. Considering the several limitations of this study and limitations in general of registry data, additional data from properly conducted clinical trials will be helpful. The NAVIGATE (Clinical Evaluation of superDimensionTM Navigation System for Electromagnetic Navigation Bronchoscopy) trial is industry sponsored (Medtronic), prospective, single arm, non-randomized observational study of ENB use and plans to recruit 2,500 patients from 75 international sites (39). The primary aim is incidence of pneumothorax related to ENB with several other secondary endpoints. While there is no question about a low rate of pneumothorax with carefully performed ENB, the secondary end-points may provide us with information about various practice patterns and outcomes of a large group of patients.
Concerns have been raised about the use of ENB in patients with pacemakers and defibrillators. The magnetic field generated by the ENB is very weak with a strength of <0.0001 Tesla (40). In small studies, no complication or disruption in pacemaker function was noted after ENB (41,42). ENB is helpful for dye marking of PPLs for surgical resection (43,44). Placement of fiducials for surgical resection and radiation treatment with lower risk of pneumothorax as compared to CT placement has been reported with ENB (45-47). The cost of equipment and the need to perform additional CT imaging are potential drawbacks of this technology. Lesions without any airway leading into them may not be navigable although bronchoscopic transparenchymal nodule access (BTNA) techniques have been recently described (48-50). ENB is not a real-time technology and target displacement due to respiratory motion, bronchoscope wedging (51), new pleural effusions or pneumothorax can cause sampling errors.
Transparenchymal nodule access
Presence of air bronchograms sign or a bronchus leading to the peripheral pulmonary lesion has been a consistent factor associated with high yield with image guidance bronchoscopy. Unfortunately, several lesions do not have a bronchus leading to them and are not reachable by current bronchoscopic methods. BTNA involves the technique of making a transbronchial passage to the nodules, which are away from the airways.
The transbronchial access tool (TBAT) or Cross CountryTM (Medtronic, USA) is used with superDimension i-LogicTM system. It has a guide wire, which is used to penetrate the bronchial wall thus leading it to the PPL followed by advancing a dilation catheter over this guide wire followed by passage of ECW over the dilatation catheter. The TBAT is removed and sampling equipment passed into the ECW for sampling. Only limited literature in this technique is available. A recent case series descried three cases where additional imaging confirmation with cone beam CT (2/3 cases) and fluoroscopy was also utilized (50). No procedural complication was described. Another similar procedure in development is called BTNA where an exit route for transparenchymal pathway without any intervening blood vessels is selected. The ArchimedesTM planner (Bronchus Medical, San Jose, CA, USA) is the VBN system utilized and it has features like major vessel detection, segmentations of vessels down to 1 mm and virtual Doppler to assess proximity of vessels to the airways. It allows two airway pathways and two tunnels paths. One the airway closest to the lesion has been reached; a vessel free-tunneled path to the lesion is made using fused fluoroscopic assistance. A coring needle is passed inside flexible bronchoscope into the selected point of entry, followed by balloon dilatation of this hole and then creation of tunnel in lung parenchyma using a sheath with a blunt dissection stylet. Only preliminary data on this technology is available at this time and a single feasibility study of twelve patients with PPL showed that a tunnel pathway could be made in ten cases (83%) with successful biopsy sampling (48). The two cases where this was not feasible had PPL located in the left upper lobe apex and a vessel free straight path for tunnel could not be made (limitations of bronchoscope size and angulation and proximity of aorta and pulmonary artery).
Although promising, additional studies documenting superior outcomes and higher yield are required before these techniques are ready for prime time.
Future directions
The last decade has seen considerable progress in the field of bronchoscopic sampling of PPLs with significant increase in yield. Enhancement in current technology is developing at a rapid pace with easier operability of equipment and improved ability for real time confirmation with cone-beam CT and RP-EBUS (52). Development of advanced technologies like optical coherence technology (OCT) and fibered confocal laser microscopy (FCFM) may additionally help in precise selection of the site of sampling (Figure 3) (53,54). In OCT, the backscattering of light is used to attain cross-sectional images of tissue. Investigators compared the obtained images to the histopathologic diagnosis of endobronchial malignancy (55). Animal models have also been used to identify different layers of the trachea wall (56). McLaughlin et al. were able to image small bronchioles alveoli and blood vessels using OCT (57). They used small needles (30 gauge) to minimize parenchymal tissue distortion. Excised lungs from three different types of animals were used.
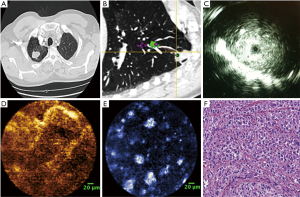
FCFM was also known as probe-based confocal laser microendoscopy uses a thin 1 mm fiberoptic probe to record real-time images (58). Yserbyt et al. published FCFM experience in five patients with lung diseases. They were able to identify alveolar duct diameter, the thickness of elastic fibers, identify and alveolar cells and determine their autofluorescence intensity (59). By recognizing certain endomicroscopic patterns, they were able to discriminate between normal and abnormal bronchial mucosa and alveolar tissue in four patients. Data on FCFM has not been consistent and further technique improvement, and more investigations are needed (60). FCFM and OCT have been combined to evaluate the alveolar dynamics and elastin functionality during ventilation in a mouse model (61). In human studies, they have the potential to providing near histology detailed images to the entire bronchial tree including the parenchymal and vascular tissue and mediastinal lymph nodes (62). Recently, Harris et al. described the use of diffuse reflectance and fluorescence spectroscopy to obtain images of lung tissue obtained by transbronchial biopsies. The study demonstrated the feasibility of imaging the small specimens with moderate sensitivity (77.3%) and specificity (73.1%) in discriminating benign from malignant samples and high sensitivity (90.9%) and specificity (100%) in distinguishing malignant from necrotic samples (63). The study was limited by the small sample size, and more studies are required to improve the technique and to investigate a bronchoscopic in vivo role for spectroscopic optical imaging.
These advances have instilled so much confidence in the interventional pulmonary community is gearing up to take the leap of treating peripheral lung cancers with bronchoscopic interventions. A wide variety of available options can be utilized including localized intratumoral chemotherapy, brachytherapy, radiofrequency ablation, interstitial photodynamic therapy etc. (64-67). While studies and clinical data of such studies will be a welcome step, it is extremely important to first fully optimize the current image guidance technology to achieve favorable outcomes. It appears that the current progress will continue and exciting future awaits interventional pulmonary medicine.
Acknowledgements
None.
Footnote
Conflicts of Interest: The authors have no conflicts of interest to declare.
References
- Wang Memoli JS, Nietert PJ, Silvestri GA. Meta-analysis of guided bronchoscopy for the evaluation of the pulmonary nodule. Chest 2012;142:385-93. [Crossref] [PubMed]
- Baaklini WA, Reinoso MA, Gorin AB, et al. Diagnostic yield of fiberoptic bronchoscopy in evaluating solitary pulmonary nodules. Chest 2000;117:1049-54. [Crossref] [PubMed]
- Rivera MP, Mehta AC, Wahidi MM. Establishing the diagnosis of lung cancer: Diagnosis and management of lung cancer, 3rd ed: American College of Chest Physicians evidence-based clinical practice guidelines. Chest 2013;143:e142S-65S.
- Minami H, Ando Y, Nomura F, et al. Interbronchoscopist variability in the diagnosis of lung cancer by flexible bronchoscopy. Chest 1994;105:1658-62. [Crossref] [PubMed]
- Dolina MY, Cornish DC, Merritt SA, et al. Interbronchoscopist variability in endobronchial path selection: a simulation study. Chest 2008;133:897-905. [Crossref] [PubMed]
- Steinfort DP, Bonney A, See K, et al. Sequential multimodality bronchoscopic investigation of peripheral pulmonary lesions. Eur Respir J 2016;47:607-14. [Crossref] [PubMed]
- Eberhardt R, Kahn N, Gompelmann D, et al. LungPoint--a new approach to peripheral lesions. J Thorac Oncol 2010;5:1559-63. [Crossref] [PubMed]
- Asano F, Eberhardt R, Herth FJ. Virtual bronchoscopic navigation for peripheral pulmonary lesions. Respiration 2014;88:430-40. [Crossref] [PubMed]
- Tamiya M, Okamoto N, Sasada S, et al. Diagnostic yield of combined bronchoscopy and endobronchial ultrasonography, under LungPoint guidance for small peripheral pulmonary lesions. Respirology 2013;18:834-9. [Crossref] [PubMed]
- Ishida T, Asano F, Yamazaki K, et al. Virtual bronchoscopic navigation combined with endobronchial ultrasound to diagnose small peripheral pulmonary lesions: a randomised trial. Thorax 2011;66:1072-7. [Crossref] [PubMed]
- Asano F, Shinagawa N, Ishida T, et al. Virtual bronchoscopic navigation combined with ultrathin bronchoscopy. A randomized clinical trial. Am J Respir Crit Care Med 2013;188:327-33. [Crossref] [PubMed]
- Asano F, Matsuno Y, Tsuzuku A, et al. Diagnosis of peripheral pulmonary lesions using a bronchoscope insertion guidance system combined with endobronchial ultrasonography with a guide sheath. Lung Cancer 2008;60:366-73. [Crossref] [PubMed]
- Tanaka M, Satoh M, Kawanami O, et al. A new bronchofiberscope for the study of diseases of very peripheral airways. Chest 1984;85:590-4. [Crossref] [PubMed]
- Hasegawa S, Hitomi S, Murakawa M, et al. Development of an ultrathin fiberscope with a built-in channel for bronchoscopy in infants. Chest 1996;110:1543-6. [Crossref] [PubMed]
- Yamamoto S, Ueno K, Imamura F, et al. Usefulness of ultrathin bronchoscopy in diagnosis of lung cancer. Lung Cancer 2004;46:43-8. [Crossref] [PubMed]
- Oki M, Saka H, Kitagawa C, et al. Novel thin bronchoscope with a 1.7-mm working channel for peripheral pulmonary lesions. Eur Respir J 2008;32:465-71. [Crossref] [PubMed]
- Oki M, Saka H, Ando M, et al. Ultrathin Bronchoscopy with Multimodal Devices for Peripheral Pulmonary Lesions. A Randomized Trial. Am J Respir Crit Care Med 2015;192:468-76. [Crossref] [PubMed]
- Kurimoto N, Murayama M, Yoshioka S, et al. Analysis of the internal structure of peripheral pulmonary lesions using endobronchial ultrasonography. Chest 2002;122:1887-94. [Crossref] [PubMed]
- Kuo CH, Lin SM, Chen HC, et al. Diagnosis of peripheral lung cancer with three echoic features via endobronchial ultrasound. Chest 2007;132:922-9. [Crossref] [PubMed]
- Izumo T, Sasada S, Chavez C, et al. Radial endobronchial ultrasound images for ground-glass opacity pulmonary lesions. Eur Respir J 2015;45:1661-8. [Crossref] [PubMed]
- Paone G, Nicastri E, Lucantoni G, et al. Endobronchial ultrasound-driven biopsy in the diagnosis of peripheral lung lesions. Chest 2005;128:3551-7. [Crossref] [PubMed]
- Kurimoto N, Miyazawa T, Okimasa S, et al. Endobronchial ultrasonography using a guide sheath increases the ability to diagnose peripheral pulmonary lesions endoscopically. Chest 2004;126:959-65. [Crossref] [PubMed]
- Chao TY, Chien MT, Lie CH, et al. Endobronchial ultrasonography-guided transbronchial needle aspiration increases the diagnostic yield of peripheral pulmonary lesions: a randomized trial. Chest 2009;136:229-36. [Crossref] [PubMed]
- Yamada N, Yamazaki K, Kurimoto N, et al. Factors related to diagnostic yield of transbronchial biopsy using endobronchial ultrasonography with a guide sheath in small peripheral pulmonary lesions. Chest 2007;132:603-8. [Crossref] [PubMed]
- Steinfort DP, Khor YH, Manser RL, et al. Radial probe endobronchial ultrasound for the diagnosis of peripheral lung cancer: systematic review and meta-analysis. Eur Respir J 2011;37:902-10. [Crossref] [PubMed]
- Chen A, Chenna P, Loiselle A, et al. Radial probe endobronchial ultrasound for peripheral pulmonary lesions. A 5-year institutional experience. Ann Am Thorac Soc 2014;11:578-82. [Crossref] [PubMed]
- Bowling MR, Kohan MW, Walker P, et al. The effect of general anesthesia versus intravenous sedation on diagnostic yield and success in electromagnetic navigation bronchoscopy. J Bronchology Interv Pulmonol 2015;22:5-13. [Crossref] [PubMed]
- Dhillon SS, Dexter EU. Advances in bronchoscopy for lung cancer. J Carcinog 2012;11:19. [Crossref] [PubMed]
- Bertoletti L, Robert A, Cottier M, et al. Accuracy and feasibility of electromagnetic navigated bronchoscopy under nitrous oxide sedation for pulmonary peripheral opacities: an outpatient study. Respiration 2009;78:293-300. [Crossref] [PubMed]
- Makris D, Scherpereel A, Leroy S, et al. Electromagnetic navigation diagnostic bronchoscopy for small peripheral lung lesions. Eur Respir J 2007;29:1187-92. [Crossref] [PubMed]
- Eberhardt R, Anantham D, Herth F, et al. Electromagnetic navigation diagnostic bronchoscopy in peripheral lung lesions. Chest 2007;131:1800-5. [Crossref] [PubMed]
- Lamprecht B, Porsch P, Wegleitner B, et al. Electromagnetic navigation bronchoscopy (ENB): Increasing diagnostic yield. Respir Med 2012;106:710-5. [Crossref] [PubMed]
- Pearlstein DP, Quinn CC, Burtis CC, et al. Electromagnetic navigation bronchoscopy performed by thoracic surgeons: one center's early success. Ann Thorac Surg 2012;93:944-9; discussion 949-50. [Crossref] [PubMed]
- Zhang W, Chen S, Dong X, et al. Meta-analysis of the diagnostic yield and safety of electromagnetic navigation bronchoscopy for lung nodules. J Thorac Dis 2015;7:799-809. [PubMed]
- Gex G, Pralong JA, Combescure C, et al. Diagnostic yield and safety of electromagnetic navigation bronchoscopy for lung nodules: a systematic review and meta-analysis. Respiration 2014;87:165-76. [Crossref] [PubMed]
- Eberhardt R, Anantham D, Ernst A, et al. Multimodality bronchoscopic diagnosis of peripheral lung lesions: a randomized controlled trial. Am J Respir Crit Care Med 2007;176:36-41. [Crossref] [PubMed]
- Ost DE, Ernst A, Lei X, et al. Diagnostic Yield and Complications of Bronchoscopy for Peripheral Lung Lesions. Results of the AQuIRE Registry. Am J Respir Crit Care Med 2016;193:68-77. [Crossref] [PubMed]
- McWilliams A. Does AQuIRE challenge the role of navigational bronchoscopy for peripheral pulmonary lesions? Ann Transl Med 2016;4:406. [Crossref] [PubMed]
- Folch EE, Bowling MR, Gildea TR, et al. Design of a prospective, multicenter, global, cohort study of electromagnetic navigation bronchoscopy. BMC Pulm Med 2016;16:60. [Crossref] [PubMed]
- Magnani A, Matheoud R, Brambilla M, et al. In vitro tests of electromagnetic interference of electromagnetic navigational bronchoscopy to implantable cardioverter defibrillators. Europace 2012;14:1054-9. [Crossref] [PubMed]
- Khan AY, Berkowitz D, Krimsky WS, et al. Safety of pacemakers and defibrillators in electromagnetic navigation bronchoscopy. Chest 2013;143:75-81. [Crossref] [PubMed]
- Magnani A, Balbo P, Facchini E, et al. Lack of interference of electromagnetic navigation bronchoscopy to implanted cardioverter-defibrillator: in-vivo study. Europace 2014;16:1767-71. [Crossref] [PubMed]
- Bolton WD, Richey J, Ben-Or S, et al. Electromagnetic Navigational Bronchoscopy: A Safe and Effective Method for Fiducial Marker Placement in Lung Cancer Patients. Am Surg 2015;81:659-62. [PubMed]
- Awais O, Reidy MR, Mehta K, et al. Electromagnetic Navigation Bronchoscopy-Guided Dye Marking for Thoracoscopic Resection of Pulmonary Nodules. Ann Thorac Surg 2016;102:223-9. [Crossref] [PubMed]
- Kupelian PA, Forbes A, Willoughby TR, et al. Implantation and stability of metallic fiducials within pulmonary lesions. Int J Radiat Oncol Biol Phys 2007;69:777-85. [Crossref] [PubMed]
- Minnich DJ, Bryant AS, Wei B, et al. Retention Rate of Electromagnetic Navigation Bronchoscopic Placed Fiducial Markers for Lung Radiosurgery. Ann Thorac Surg 2015;100:1163-5; discussion 1165-6. [Crossref] [PubMed]
- Nabavizadeh N, Zhang J, Elliott DA, et al. Electromagnetic navigational bronchoscopy-guided fiducial markers for lung stereotactic body radiation therapy: analysis of safety, feasibility, and interfraction stability. J Bronchology Interv Pulmonol 2014;21:123-30. [Crossref] [PubMed]
- Herth FJ, Eberhardt R, Sterman D, et al. Bronchoscopic transparenchymal nodule access (BTPNA): first in human trial of a novel procedure for sampling solitary pulmonary nodules. Thorax 2015;70:326-32. [Crossref] [PubMed]
- Harzheim D, Sterman D, Shah PL, et al. Bronchoscopic Transparenchymal Nodule Access: Feasibility and Safety in an Endoscopic Unit. Respiration 2016;91:302-6. [Crossref] [PubMed]
- Anciano C, Brown C, Bowling M. Going Off Road: The First Case Reports of the Use of the Transbronchial Access Tool With Electromagnetic Navigational Bronchoscopy. J Bronchology Interv Pulmonol 2017;24:253-6. [PubMed]
- Leira HO, Lango T, Sorger H, et al. Bronchoscope-induced displacement of lung targets: first in vivo demonstration of effect from wedging maneuver in navigated bronchoscopy. J Bronchology Interv Pulmonol 2013;20:206-12. [Crossref] [PubMed]
- Hohenforst-Schmidt W, Zarogoulidis P, Vogl T, et al. Cone Beam Computertomography (CBCT) in Interventional Chest Medicine - High Feasibility for Endobronchial Realtime Navigation. J Cancer 2014;5:231-41. [Crossref] [PubMed]
- Pahlevaninezhad H, Lee AM, Ritchie A, et al. Endoscopic Doppler optical coherence tomography and autofluorescence imaging of peripheral pulmonary nodules and vasculature. Biomed Opt Express 2015;6:4191-9. [Crossref] [PubMed]
- Hassan T, Piton N, Lachkar S, et al. A Novel Method for In Vivo Imaging of Solitary Lung Nodules Using Navigational Bronchoscopy and Confocal Laser Microendoscopy. Lung 2015;193:773-8. [Crossref] [PubMed]
- Michel RG, Kinasewitz GT, Fung KM, et al. Optical coherence tomography as an adjunct to flexible bronchoscopy in the diagnosis of lung cancer: a pilot study. Chest 2010;138:984-8. [Crossref] [PubMed]
- Rodrigues AJ, Takimura CK, Lemos Neto PA, et al. Optical coherence tomography in conjunction with bronchoscopy. J Bras Pneumol 2012;38:174-80. [Crossref] [PubMed]
- McLaughlin RA, Yang X, Quirk BC, et al. Static and dynamic imaging of alveoli using optical coherence tomography needle probes. J Appl Physiol 2012;113:967-74. [Crossref] [PubMed]
- Thiberville L, Salaun M, Lachkar S, et al. Confocal fluorescence endomicroscopy of the human airways. Proc Am Thorac Soc 2009;6:444-9. [Crossref] [PubMed]
- Yserbyt J, Dooms C, Ninane V, et al. Perspectives using probe-based confocal laser endomicroscopy of the respiratory tract. Swiss Med Wkly 2013;143:w13764. [PubMed]
- Streba CT, Giltan AM, Gheonea IA, et al. Utility of confocal laser endomicroscopy in pulmonology and lung cancer. Rom J Morphol Embryol 2016;57:1221-7. [PubMed]
- Thiberville L, Moreno-Swirc S, Vercauteren T, et al. In vivo imaging of the bronchial wall microstructure using fibered confocal fluorescence microscopy. Am J Respir Crit Care Med 2007;175:22-31. [Crossref] [PubMed]
- Wijmans L, d'Hooghe JN, Bonta PI, et al. Optical coherence tomography and confocal laser endomicroscopy in pulmonary diseases. Curr Opin Pulm Med 2017;23:275-83. [Crossref] [PubMed]
- Harris K, Rohrbach DJ, Attwood K, et al. Optical imaging of tissue obtained by transbronchial biopsies of peripheral lung lesions. J Thorac Dis 2017. [Epub ahead of Print]. [Crossref] [PubMed]
- Eberhardt R, Kahn N, Herth FJ. 'Heat and destroy': bronchoscopic-guided therapy of peripheral lung lesions. Respiration 2010;79:265-73. [Crossref] [PubMed]
- Harris K, Puchalski J, Sterman D. Recent Advances in Bronchoscopic Treatment of Peripheral Lung Cancers. Chest 2017;151:674-85. [Crossref] [PubMed]
- Harms W, Krempien R, Grehn C, et al. Electromagnetically navigated brachytherapy as a new treatment option for peripheral pulmonary tumors. Strahlenther Onkol 2006;182:108-11. [Crossref] [PubMed]
- Koizumi T, Tsushima K, Tanabe T, et al. Bronchoscopy-Guided Cooled Radiofrequency Ablation as a Novel Intervention Therapy for Peripheral Lung Cancer. Respiration 2015;90:47-55. [Crossref] [PubMed]